Accuracy and Precision in Spectrophotometry
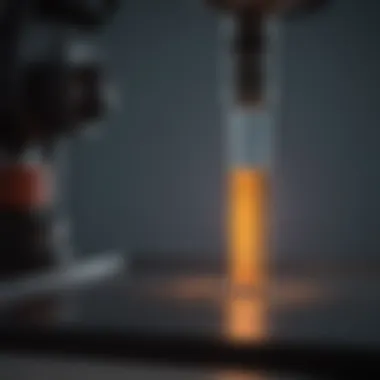
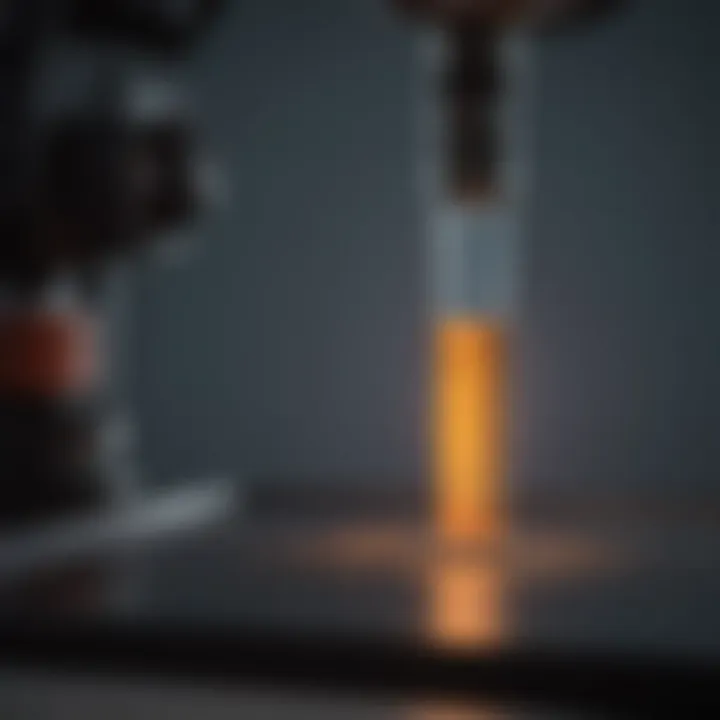
Intro
Spectrophotometry is a critical technique used widely in various scientific fields, including chemistry, biology, and environmental science. It serves as a fundamental tool for quantifying substances based on their interaction with light. In this article, we will examine the crucial concepts of accuracy and precision in spectrophotometric measurements. These two parameters, though often used interchangeably, possess distinct meanings and implications for the reliability of experimental data.
Understanding how to enhance both accuracy and precision is not just an academic exercise; it is essential for producing credible, reproducible results in scientific research. Researchers, educators, and students alike need to grasp these concepts to optimize their methodologies effectively.
Through this exploration, we will delve into definitions, discuss the factors influencing both accuracy and precision, and review methodologies for assessment and improvement. By emphasizing the interdependencies between these key facets of measurement, we aspire to equip readers with the tools necessary for better experimental practices.
Foreword to Spectrophotometry
Spectrophotometry is not just a fundamental laboratory technique; it is a cornerstone of modern scientific research. Its ability to quantify the concentration of substances based on their interaction with light plays a crucial role in numerous fields, including chemistry, biology, environmental science, and medicine. In exploring accuracy and precision within this domain, we must first comprehend what spectrophotometry entails. \
The importance of this topic lies in its direct implications for research reliability. Accurate measurements are vital for drawing valid conclusions, while precision ensures reproducibility. A proper grasp of these concepts not only enhances experimental outcomes but also enriches educational efforts. By understanding spectrophotometry, students and professionals can build a strong foundation for their scientific inquiries.
Definition of Spectrophotometry
Spectrophotometry refers to the measurement of how much a chemical substance absorbs light by measuring the intensity of light as a beam of light passes through a sample solution. Generally, this process involves a light source, a sample, and a detector. Different substances absorb light at different wavelengths, allowing for the identification and quantification of those substances. The spectral data can provide insight into the characteristics of the sample being analyzed, thus making spectrophotometry indispensable in various applications.
Applications of Spectrophotometry in Scientific Research
Spectrophotometry finds a multitude of applications across different scientific disciplines:
- Chemical Analysis: It is commonly used to determine the concentration of solutes in a solution. This is pivotal for reaction kinetics, where knowing the concentration at different time points is essential.
- Biology and Biotechnology: Researchers harness this technique for protein quantification, DNA analysis, and enzyme activity assays. Specific wavelengths can be used to assess the presence of biomolecules.
- Environmental Science: Spectrophotometry aids in measuring pollutants and assessing water quality by analyzing different absorbance spectra.
- Clinical Diagnostics: It plays a role in blood analysis and may be used to measure blood levels of various substances, including hormones and drugs.
These applications underscore the indispensable nature of spectrophotometry in discovering new knowledge and pushing the boundaries of understanding in various scientific arenas.
Defining Accuracy in Measurements
The process of defining accuracy in measurements is essential in the realm of spectrophotometry. It sets the foundation for ensuring that the results obtained from any spectrophotometric analysis are valid and dependable. Accuracy reflects the degree to which a measurement aligns with the true value. Therefore, a clear understanding of accuracy is paramount for researchers and professionals who rely on precise spectrophotometric data.
Accurate measurements are necessary for drawing correct conclusions in experiments. This is particularly significant in scientific research and industry applications where precision dictates the quality of results. In spectrophotometry, accuracy directly influences reliability. Without it, the integrity of the data may be compromised, potentially leading to erroneous interpretations and decisions.
Concept of Accuracy in Spectrophotometry
Accuracy in the context of spectrophotometry refers to how closely the measured absorbance corresponds with the actual concentration of the analyte. The concept is multifaceted. It is not merely about measuring values but ensuring those values reflect reality. Factors like instrument calibration, sample integrity, and environmental effects all contribute to accuracy assessment. Given the quantitative nature of spectrophotometric analysis, establishing a robust accuracy framework is vital.
Factors Influencing Accuracy of Spectrophotometers
Several key factors can influence the accuracy of spectrophotometers:
- Instrument Calibration: Regular calibration against known standards ensures that the spectrophotometer provides accurate readings. This involves comparing the instrument's results with reference values.
- Environment: Temperature, humidity, and light interference can all affect measurements. Conducting tests in a controlled environment can minimize these influences.
- Sample Properties: The physical and chemical nature of the sample, including turbidity and color, plays a crucial role. Inaccurate sample handling or preparation may lead to deviations from true values.
- Human Error: Operator skill and technique are also critical. Proper training can reduce these errors.
Calibration Techniques to Improve Accuracy
Improving accuracy in spectrophotometric measurements often involves several calibration techniques:
- Standard Calibration Curve: Creating a calibration curve using known concentrations improves the instrument's reliability. The curve establishes a relationship between absorbance and concentration.
- Frequency of Calibration: Regular calibration is essential. Changes in the environment or instrument can affect accuracy over time, necessitating frequent checks.
- Use of Certified Reference Materials: Employing high-quality reference materials can enhance validation efforts. Ensuring that these materials are traceable to international standards further verifies accuracy.
In summary, defining and understanding accuracy in spectrophotometry is crucial. It involves necessary considerations across multiple factors, from instrument calibration to environmental conditions. Employing robust calibration techniques ensures that experiments yield reliable results, ultimately enabling sound scientific reasoning and decision-making.
Understanding Precision in Measurements
Precision is a critical aspect in the field of spectrophotometry. Its importance lies in how closely the measured values cluster around a mean. In the realm of scientific measurements, particularly with instruments like spectrophotometers, precision reflects the instrument's ability to yield consistent results upon repeated tests under unchanged conditions. This consistency is crucial since experiments often require high levels of replicability to support reliability and validity of findings.
One of the primary benefits of understanding precision is that it allows researchers to gauge the quality of their measurements. When precision is assessed, scientists can ascertain whether variations in results are due to inherent instrument limitations or potential human error. Therefore, improving precision can directly enhance the overall quality of analytical results. This necessitates not only awareness of the definitions and statistical evaluations of precision but also a keen understanding of the components influencing it.
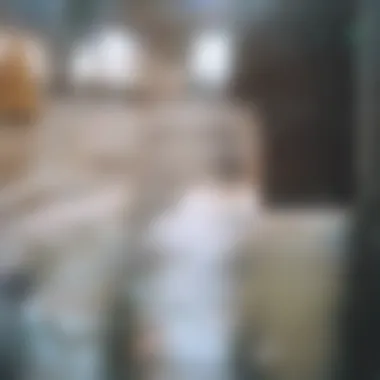
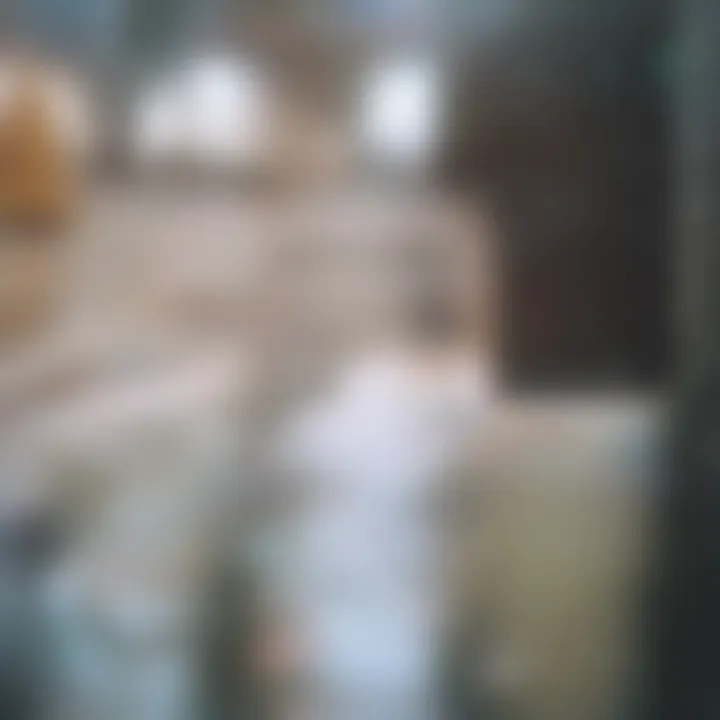
Defining Precision in the Context of Spectrophotometry
Precision in spectrophotometry refers to the degree to which repeated measurements under similar conditions yield the same results. It is essential to differentiate it from accuracy; while accuracy concerns the closeness of measurements to a true or accepted value, precision focuses solely on repeatability. In spectrophotometric applications, precise measurements ensure that the output is reliable, contributing to the integrity of scientific work.
Precision can be affected by numerous factors, including measurement conditions, calibration of the instrument, and even sample handling. Understanding these influences can foster improved practices that ultimately facilitate higher precision in measuring light absorption and transmission.
Components Affecting Precision
Multiple elements play a role in determining the precision of spectrophotometric measurements. Some key components include:
- Instrument Calibration: Regular calibration with known standards helps maintain accuracy over time. If the spectrophotometer drifts from its settings, precision can suffer.
- Sample Quality: Impurities or inconsistencies in samples can add variability, thus affecting precision. Pure and homogenous samples are preferable.
- Environmental Conditions: Changes in temperature, humidity, or electromagnetic interference can yield variable results. Keeping environmental factors constant is pivotal for precision.
- Operator skill: Inexperience or inconsistency in handling the equipment can lead to variations in results. Proper training can help reduce this source of error.
By addressing these components, laboratory personnel could significantly improve the precision of their measurements.
Statistical Methods for Evaluating Precision
Evaluating precision involves an array of statistical methods that provide insights into the distribution and variance of data collected. Common techniques include:
- Standard Deviation: A fundamental metric that quantifies the amount of variation or dispersion in a set of values, helping to indicate precision.
- Coefficient of Variation: This expresses the standard deviation as a percentage of the mean, providing a relative measure of precision.
- Control Charts: These graphical representations of data over time assist in monitoring process behavior. They help detect variations that may affect precision.
"Monitoring the precision of measurements is indispensable in maintaining scientific integrity."
Utilizing these statistical tools allows researchers to systematically assess precision, ensuring robustness in results over a range of experimental conditions. Understanding precision in this context leads to more informed adjustments in methodology, facilitating re-evaluations and optimizations that enhance scientific inquiry.
The Relationship Between Accuracy and Precision
The relationship between accuracy and precision is central to understanding the effectiveness and reliability of spectrophotometric measurements. Accuracy refers to how closely the measured value aligns with the true value, while precision pertains to the repeatability or consistency of measurements under the same conditions. Together, these elements provide insight into the validity of the results produced by spectrophotometers. Understanding their interplay is crucial for enhancing experimental outcomes in various scientific applications.
Both accuracy and precision play significant roles in the realm of scientific research. Without accuracy, the data derived from experiments can be misleading, leading to erroneous conclusions. On the other hand, high precision without accuracy can also be inconsequential, as consistent results that do not reflect the true values are ultimately unhelpful. The relationship emphasizes that to attain reliable results in spectrophotometric measurements, both parameters must be optimized simultaneously.
Interconnection of Accuracy and Precision
The interconnection between accuracy and precision is often illustrated through the concept of measurement uncertainty. When evaluating a new spectrophotometer or a measurement technique, it is necessary to assess both components together. If a spectrophotometer provides consistent measurements (high precision) but those measurements are consistently off from the true value (low accuracy), the data can yield a false sense of reliability.
Factors affecting both accuracy and precision include instrumental errors, environmental conditions, and human factors. Consequently, comprehending this interconnectedness allows researchers to calibrate and validate spectrophotometric equipment more effectively.
Examples of Accuracy and Precision Interplay in Spectrophotometry
To further illustrate the connection between accuracy and precision, consider a laboratory measuring the concentration of a dye solution using a specific spectrophotometer. If multiple measurements return a narrow range of values, this demonstrates high precision. However, if those values are far from the true concentration of the dye, it highlights a lack of accuracy.
One practical example can be found in the assessment of absorbance for various solutions. When comparing absorbance readings from a high-quality spectrophotometer and a lower-quality model, the former might show precise measurements that fall close to the expected absorbance based on known concentrations, indicating higher accuracy. In contrast, a lower-quality model might yield readings that are highly variable, showing low precision.
The importance of examining accuracy and precision collectively cannot be overstated. The interplay impacts data interpretation, affects the reliability of results, and influences subsequent experimental designs. Recognizing these relationships fosters a deeper understanding of spectrophotometric principles, ultimately contributing to more rigorous and scientifically sound research.
"In the pursuit of scientific knowledge, understanding the nuances of accuracy and precision is as critical as the methodologies employed to obtain data."
By emphasizing the importance of these concepts within spectrophotometry, researchers can make informed decisions to enhance the validity and reproducibility of their findings.
Assessing Spectrophotometer Performance
Assessing the performance of spectrophotometers is crucial for ensuring accurate and reliable measurements. This process highlights elements that directly influence scientific outcomes, making it pivotal in both research and educational settings. By examining performance parameters, users can determine how well a spectrophotometer operates under varied conditions. This can include assessing measurement accuracy, ensuring reproducibility, and identifying any deficiencies that may hinder effective use of this analytical tool.
The importance of performance assessment cannot be overstated. It allows researchers and technicians to confirm that their instruments meet predefined standards. Regular evaluations contribute to a deeper understanding of how these devices function in specific laboratory environments. Consequently, better decision-making can occur concerning instrument selection and its application in experimental designs.
Moreover, assessment fosters improved trust in results derived from spectrophotometric data. Ensuring that a device performs well builds confidence in its output, which is vital for peer-reviewed publications and industrial applications.
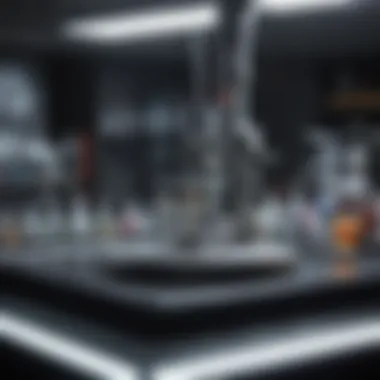
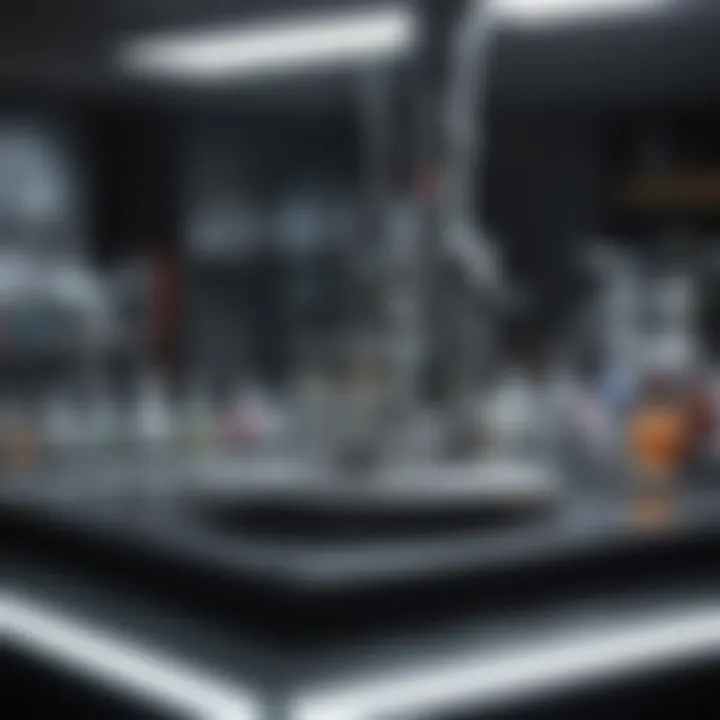
Key Performance Indicators for Spectrophotometers
Key performance indicators (KPIs) are essential for determining how well a spectrophotometer meets its intended purposes. Common KPIs include:
- Wavelength Accuracy: The precision with which the instrument can identify specific wavelengths. Accurate wavelength detection ensures reliable results across various applications.
- Photometric Accuracy: This measures how closely a spectrophotometer's readings match the true values. Photometric accuracy is critical when quantifying concentrations in samples.
- Linearity: The ability to produce consistent results across a range of concentrations. A linear response confirms that the instrument maintains reliability in different conditions.
- Sensitivity: Describes the lowest concentration level that can be detected. High sensitivity is required in applications involving dilute solutions.
- Dynamic Range: This indicates the range of concentrations over which the instrument provides accurate measurements. A broad dynamic range is essential for analyzing samples with wide concentration variations.
Consistent monitoring of these KPIs can lead to enhanced performance outcomes, highlighting areas needing improvement. For example, if a spectrophotometer exhibits low sensitivity, it may require calibration or even replacement.
Routine Maintenance and Performance Checks
Routine maintenance of spectrophotometers is vital for sustaining performance levels. Regular performance checks help in identifying potential issues before they escalate. This includes simple tasks such as:
- Cleaning Optical Components: Dust and residue on optical surfaces can lead to inaccurate measurements. Making sure these components are well-maintained improves overall results.
- Regular Calibration: Calibration against known standards helps maintain accuracy. This should be done frequently to comply with rigorous scientific standards.
- Checking Light Sources: Light sources can degrade over time, affecting measurement quality. Regular checks ensure that the light source functions optimally.
- Software Updates: Keeping software updated alleviates bugs and enhances functionalities. This is necessary for ensuring reliability in results.
Incorporating these practices into a routine schedule ensures that spectrophotometers remain reliable and accurate over time, ultimately leading to better experimental outcomes.
Enhancing Accuracy and Precision in Spectrophotometry
Enhancing accuracy and precision in spectrophotometry is crucial. These elements play a significant role in the reliability of data obtained from measurements. Accurate and precise spectrophotometric results are necessary for various applications, including quality control, environmental analysis, and clinical diagnostics. Without proper methods to enhance these factors, the integrity of scientific research can be undermined.
Accurate measurements reflect how close results are to the true value, while precision denotes the consistency and reproducibility of those measurements. To ensure our analysis meets rigorous standards, advanced methodologies and technology must be implemented effectively.
Advanced Calibration Methods
Calibration is a foundational aspect of enhancing both accuracy and precision. Spectrophotometers must be appropriately calibrated to ensure their measurements reflect true concentration levels of analytes. Various techniques exist to achieve this goal.
- Standardization: Using reference standards is essential. Known concentrations of substances allow for a benchmark to calibrate measurements.
- Multiple Point Calibration: This method involves utilizing several standard points, which can improve the accuracy across a range of concentrations. It helps to linearize the response of the spectrophotometer.
- Software-Assisted Calibration: Many modern spectrophotometers incorporate software that aids in calibration. This can automate the calibration process and account for non-linearity in response.
These techniques enhance the performance of the device and contribute to reliable output. By regularly applying these advanced calibration methods, researchers can minimize errors effectively.
Technology Advances in Spectrophotometer Design
Recent technological advances have significantly impacted how spectrophotometers operate. These innovations have improved measurement capabilities. Newer models are designed with enhanced detection systems, which provide higher sensitivity and broader spectral ranges.
- Miniaturization: Contemporary spectrophotometers are often more compact. This facilitates the portability and ease of use without compromising measurement quality.
- Integration with Other Technologies: Some spectrophotometers now include features such as multi-sampling, allowing for the simultaneous analysis of multiple samples.
- User-Friendly Interfaces: Many new devices come equipped with intuitive software, allowing users to obtain data easily and analyze results more efficiently.
These advancements not only boost performance but also encourage wider adoption across various fields. In essence, staying abreast of technological progress in spectrophotometry is fundamental for enhancing accuracy and precision.
Comparative Analysis of Spectrophotometer Types
The process of analyzing different types of spectrophotometers is vital in determining their operational effectiveness and application suitability. Understanding how each type of spectrophotometer measures accuracy and precision can drive informed decisions in choosing the right instrument for specific tasks. Spectrophotometers differ significantly in their design, function, and performance metrics, which can influence research outcomes greatly.
Types of Spectrophotometers and Their Accuracy
Spectrophotometers are classified mainly into single-beam and double-beam designs. Each type exhibits varied accuracy due to their operational mechanics.
Single-Beam Spectrophotometers measure absorbance or transmittance of samples by comparing the sample beam to a blank beam sequentially. The accuracy can be influenced by instrument drift and environmental variations. Proper calibration is crucial to maintain accuracy in results.
Double-Beam Spectrophotometers operate by splitting the light beam between a sample and a reference, allowing for simultaneous measurement. This design tends to enhance accuracy, providing built-in correction for fluctuations in light intensity. Consequently, many researchers prefer double-beam spectrophotometers for applications that demand high accuracy in results.
Other variants, like UV-Vis and Infrared Spectrophotometers, also show differences. UV-Vis models generally perform well in the 200-800 nm range, while infrared models cater to chemical bonding analysis, each bringing unique aspects in accuracy.
"The choice of spectrophotometer type profoundly impacts the validity of experimental outcomes."
Precision Differences Across Spectrophotometer Models
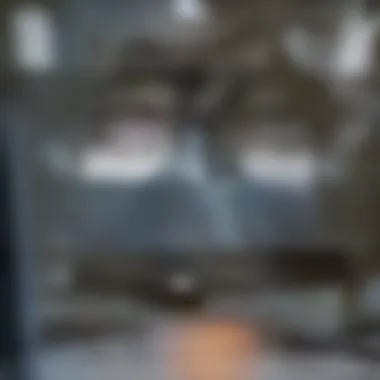
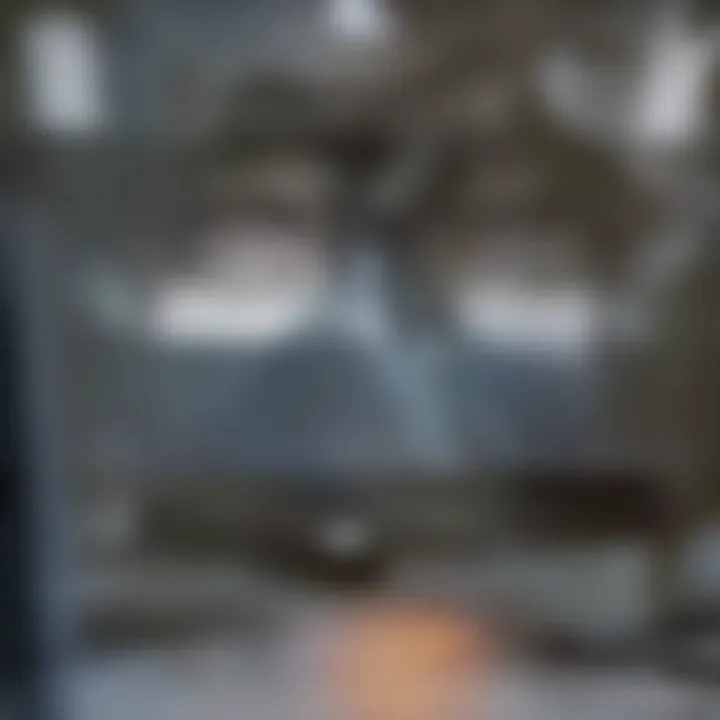
Precision, defined as the repeatability of measurements, varies among the spectrophotometer models as well.
Single-beam models can exhibit lower precision in high-throughput scenarios, especially if not recalibrated frequently. However, they still find use in routine applications with limited variance.
On the other hand, double-beam models usually provide superior precision. As they measure both sample and reference signals simultaneously, this minimizes errors caused by environmental changes that can affect single-beam measurements.
Examples of variations in precision include:
- Hand-held spectrophotometers: These often provide lower precision due to external operator variables and often lack the sophistication of larger systems.
- Benchtop spectrophotometers: Offer a higher degree of precision, making them suitable for laboratory settings.
Ultimately, choosing the correct spectrophotometer requires careful evaluation of both accuracy and precision needs. The interdependent relationship between the two determines the overall reliability of the spectrophotometric results.
Practical Considerations in Spectrophotometric Measurements
Practical considerations in spectrophotometric measurements play a crucial role in ensuring the reliability and accuracy of data obtained from spectrophotometers. The outcomes of experiments and analyses heavily depend on how well these considerations are understood and managed. Proper attention to these aspects not only enhances the quality of results but also mitigates errors that could arise during the measurement process.
Several key factors contribute to successful spectrophotometric practices. These include meticulous sample preparation, vigilance regarding environmental conditions, and adherence to best operational practices. Addressing these elements ultimately leads to more reliable data and a deeper understanding of the phenomena being studied.
Sample Preparation and Its Impact on Results
Sample preparation is one of the most influential aspects of spectrophotometric measurements. Inaccurate or improper sample preparation can lead to significant variations in results, skewing the data and potentially leading to incorrect conclusions.
- Homogeneity: Samples must be uniform. Any inconsistencies can cause absorbance values to reflect variations rather than true concentrations.
- Dilution Factors: Correct dilution techniques are essential. Miscalculations can lead to either too concentrated samples that exceed the linear range of the spectrophotometer or too diluted samples that result in lost sensitivity.
- Sample Matrix: The nature of the solvent and other matrix components can affect spectral readings. It is vital to understand how these elements interact with the analyte to avoid misleading data.
- Contamination: Protecting samples from contaminants is crucial. Even minute levels of impurities can alter the absorbance readings significantly.
Regular validation of sample preparation protocols can help researchers ensure high-quality measurements. This might include checking for consistency in absorbance readings across multiple tests.
Environmental Factors Affecting Accuracy and Precision
Environmental conditions can significantly influence the accuracy and precision of spectrophotometric measurements. Factors such as temperature, light conditions, and humidity should be monitored closely. They can introduce variability that leads to erroneous results.
- Temperature Sensitivity: Most spectrophotometers are calibrated at specific temperatures. Deviations from this can impact the kinetic properties of solutions, thus altering the absorbance.
- Lighting Conditions: Ambient light can interfere with measurements, particularly in colorimetric analyses. Ensuring that experiments are conducted in controlled lighting reduces this risk.
- Humidity Levels: High humidity can affect the behavior of hygroscopic materials within the sample or the cuvette itself. This can result in changes to light transmission properties.
Accurate spectrophotometric measurements depend not just on the instrument, but also on the environment in which they are used.
By understanding and managing these environmental factors, researchers can maintain the integrity of their measurements, leading to more consistent outcomes and substantial insights into their research topics. Proper calibration and regular maintenance of the spectrophotometer are also pivotal steps that support the overall measurement process, ensuring that both accuracy and precision are upheld.
Ending and Implications for Future Research
The exploration of accuracy and precision in spectrophotometry reveals pivotal insights for scientific measurement. This article addresses how the interplay between these two elements can significantly shape the reliability of results across various applications. Understanding their nuanced definitions and how they correlate enhances the quality of experimental outcomes in research settings.
Summary of Key Findings
In this article, several key points stand out. First, accuracy pertains to how close a measurement is to the true value, while precision relates to the reproducibility of measurements under unchanged conditions. Both factors are deeply influenced by calibration methods, sample preparation, and environmental settings.
Significant findings include:
- The importance of maintaining routine checks to ensure optimal device performance.
- Advanced calibration techniques can vastly improve measurement accuracy.
- Environmental factors, such as temperature and light conditions, must be controlled to avoid skewed results.
These findings will guide future measurements in laboratories, ensuring a robust framework for enhancing data reliability.
Importance of Ongoing Research in Spectrophotometric Techniques
Ongoing research is essential to advance techniques in spectrophotometry. New technology developments can enhance both accuracy and precision, allowing scientists to explore deeper analytical possibilities.
Key areas for future inquiry include:
- Exploring novel calibration protocols to ensure greater accuracy across diverse sample types.
- Investigation into automated spectrophotometers which could offer efficiency and consistency in measurements.
- Continuous evaluation of software improvements that can analyze data with greater statistical rigor.
As scientists strive for more dependable results, understanding the factors that influence measurement quality is crucial. Expanding research in spectrophotometric techniques is vital for maximum reliability and reproducibility, fundamental for scientific integrity.
"Precision is not enough; accuracy is the cornerstone of trust in measurements."
The implications of this ongoing work illustrate a continual quest for excellence in measurement sciences, ensuring that spectrophotometry remains a trusted tool for researchers worldwide.