Advances and Challenges in Anode Materials for Lithium-Ion Batteries
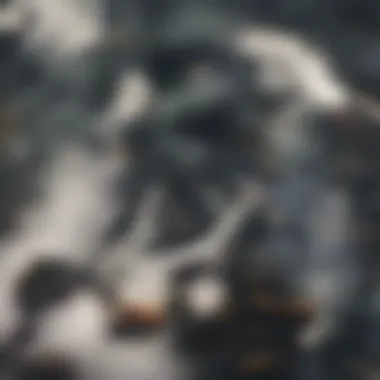
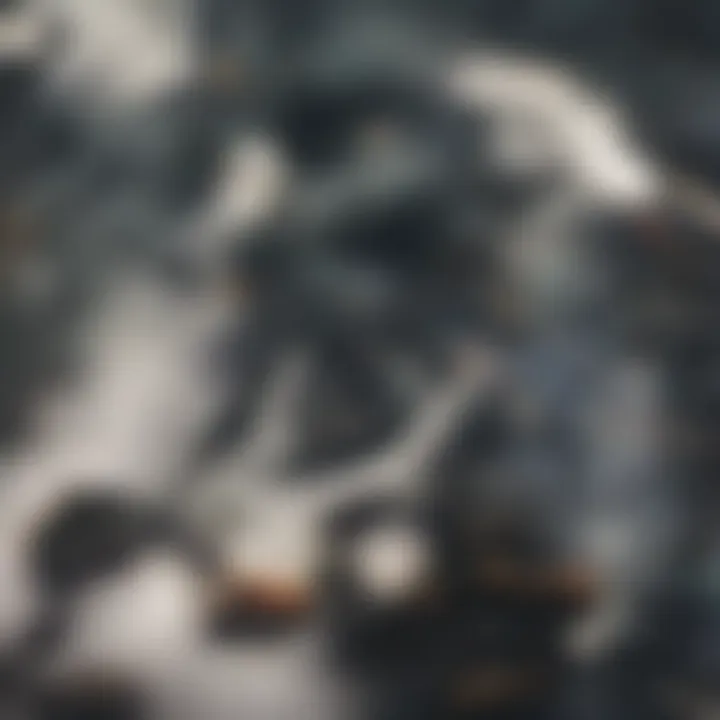
Intro
The field of lithium-ion batteries has expanded remarkably over the past few decades, catalyzing a transformation in energy storage technologies. Central to this evolution is the development of anode materials. The anode plays a pivotal role in determining the battery's overall performance, including aspects such as energy density, charge capacity, and cycle life. As the demand for more efficient and sustainable energy storage solutions grows, it is essential to analyze both traditional and innovative materials for their electrochemical properties and functionalities.
In this article, we will explore the ongoing research, the properties of various anode materials, and the challenges researchers face in optimizing these components. Understanding these materials is vital for advancing battery technology, especially as global reliance on renewable energy sources increases.
Research Methodology
Description of research design and approach
The research design for this exploration involves a systematic review of current literature and experimental studies focused on anode materials for lithium-ion batteries. This includes peer-reviewed articles, industry reports, and scholastic papers that delineate various materials utilized in battery technology. The objective is to collate findings from diverse studies to present a synthesized view of advancements and hurdles in the field.
Materials and methods used in the study
The examination involves a detailed investigation of several types of anode materials, such as graphite, silicon, lithium titanium oxide, and emerging materials like tin and metal oxides. These materials are analyzed based on their electrochemical characteristics, compatibility, and performance metrics. Key methods employed in these studies typically involve:
- Electrochemical testing: Analyzing charge-discharge profiles, potential mapping, and cycle life measurements.
- Characterization techniques: Using X-ray diffraction, scanning electron microscopy, and transmission electron microscopy to study material structures.
- Simulation models: Applying computational methods to predict various thermal and electrochemical behaviors.
From this framework, the research aims to provide clear insights into which materials yield the most promise for future battery development.
Discussion and Interpretation
Interpretation of results in the context of existing literature
The tectonic shifts in anode material development invite a closer inspection of their properties compared to established standards like graphite. Emerging materials, particularly silicon, show a significant increase in theoretical capacity, but their practical implementation raises concerns with expansion and stability over cycles. Reviewing existing literature reveals a dichotomy between capacity and longevity.
Implications for future research or practical applications
As innovations continue to permeate the domain of energy storage, the importance of sustainable anode materials cannot be understated. Future research must not only focus on optimizing performance but also address the economic and environmental impacts of sourcing and processing these materials. Practical applications will likely hinge on balancing cost, efficiency, and sustainability as the world moves towards greener energy solutions.
Prologue
Anode materials hold a significant position in the performance and functionality of lithium-ion batteries. As the demand for advanced energy storage systems increases, understanding these materials becomes crucial for both researchers and manufacturers. Lithium-ion batteries are widely utilized in consumer electronics, electric vehicles, and renewable energy storage, making the optimization of anode materials essential for improved battery performance and longevity.
Advances in anode materials can lead to enhanced energy density, faster charge times, and longer cycle life of batteries. Moreover, sustainable practices in material sourcing and recycling are becoming increasingly important in mitigating environmental impact. Thus, the ongoing research into anode development not only influences the efficiency of batteries but also addresses critical sustainability challenges. Evaluating the properties and potential applications of both traditional and emerging materials helps to outline future directions for innovation in the field.
Importance of Anode Materials
The choice of anode material directly affects the overall efficiency of lithium-ion batteries. The most common anode material, graphite, offers certain advantages, but its limitations prompt the exploration of alternatives. The capacity for lithium-ion storage, ion conductivity, and structural integrity during charge and discharge cycles are all critical factors that stem from the material composition.
Innovative anode materials, such as silicon-based compounds, show great promise for higher energy densities. The ability of silicon to accommodate more lithium ions than graphite makes it an attractive candidate, although it presents its own challenges regarding volume expansion. Thus, understanding the importance of these materials sheds light on how they can drive future innovations in energy storage technology.
Overview of Lithium-Ion Battery Technology
Lithium-ion battery technology operates on the principle of lithium ion movement between cathode and anode during charge and discharge cycles. The fundamental components include the anode, cathode, electrolyte, and separator. The anode, specifically, serves as the negative electrode where lithium ions are stored during charging. The cathode acts as the positive electrode, allowing lithium ions to flow back during discharge.
When these batteries are charged, lithium ions migrate from the cathode through the electrolyte and are embedded in the anode material. During discharge, the reverse occurs, releasing electrical energy for external use. The efficiency of this process heavily relies on the nature and characteristics of the anode material. As a result, significant advancements in anode technologies are vital for enhancing the overall performance, safety, and environmental sustainability of lithium-ion batteries.
Lithium-Ion Battery Fundamentals
The significance of understanding lithium-ion battery fundamentals is paramount for grasping the innovations in anode materials. Lithium-ion batteries are critical components of modern technology, powering a wide range of devices from smartphones to electric vehicles. The performance of these batteries relies heavily on the properties and functionalities of their components, particularly the anode. Insights into electrochemical principles and the various parts of these batteries help researchers and developers make informed decisions in material selection and optimization strategies.
Electrochemical Principles
Electrochemical principles govern the operation of lithium-ion batteries. These principles focus on the transfer of ions and electrons, which is crucial for energy storage and release. At the heart of this is the redox reaction, where lithium ions move from the anode to the cathode during charging, and reverse during discharging. This transfer is underpinned by the behavior of the electrolyte and the efficiency of the anode materials. The energy density, cycling stability, and overall efficiency of a battery are fundamentally influenced by these processes.
Components of Lithium-Ion Batteries
Lithium-ion batteries consist of several key components that work interdependently. The main components are the anode, electrolyte, cathode, and separator.
Anode
The anode serves as the site for lithium ion storage during the charging phase. It is a critical element that determines the battery's capacity and overall performance. Graphite is the most widely used material for anodes due to its high conductivity and reasonable capacity. It allows for efficient intercalation of lithium ions. However, graphite has limitations in terms of capacity scalability. Anode development focuses on alternatives such as silicon, which has a much higher capacity. Nonetheless, silicon can suffer from expansion and contraction during charge cycles, leading to mechanical failure. Thus, ongoing research aims to enhance silicon's performance through composite and nano-engineered approaches.
Electrolyte
The electrolyte is yet another vital component as it facilitates the movement of lithium ions between the anode and cathode. Liquid electrolytes are commonly used, though solid-state electrolytes are emerging due to their potential in improving safety and performance. The choice of electrolyte impacts not only the ionic conductivity but also the thermal stability and safety of the battery system. For instance, many liquid electrolytes can be flammable, raising safety concerns. Therefore, researchers are exploring alternative formulations that provide better stability and performance.
Cathode
The cathode complements the anode in the battery's function. It hosts lithium ions during the charging process and releases them during discharge. Common materials include lithium cobalt oxide, lithium iron phosphate, and lithium manganese oxide. The cathode material's energy density and thermal stability directly affect battery performance. Each material has its advantages and disadvantages; for example, lithium iron phosphate offers thermal stability but lower energy density compared to lithium cobalt oxide. Understanding the trade-offs among cathode materials is crucial for enhancing lithium-ion battery efficiency.
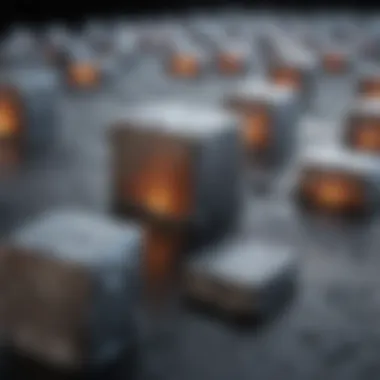
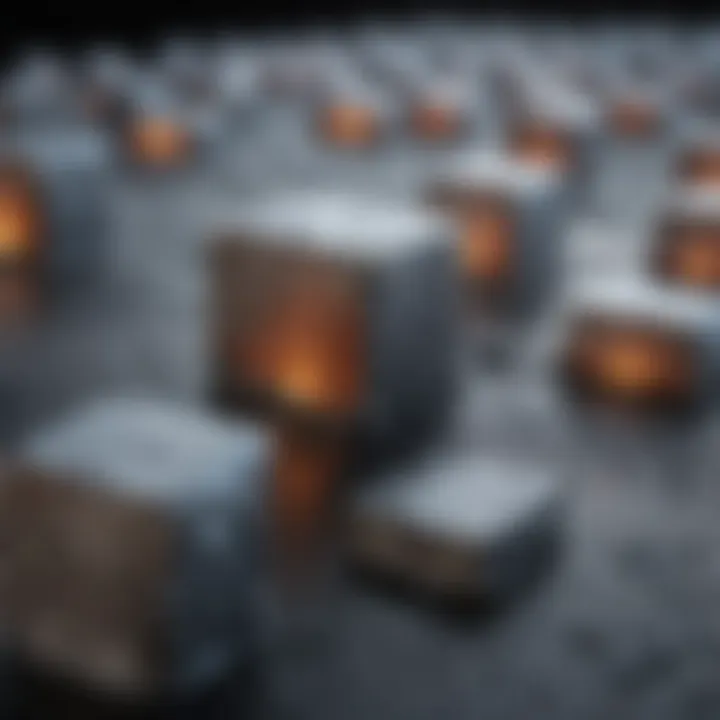
Separator
The separator plays an equally significant role in the safety and efficiency of lithium-ion batteries. It prevents electrical short circuits while allowing ionic transport. Typically made from porous materials, the separator needs to maintain mechanical integrity under varying thermal conditions while ensuring minimal impedance to ionic flow. Innovations in separator technologies can lead to improved safety profiles and energy efficiency. New materials and designs are being researched to enhance the performance and reliability of separators under extreme conditions.
The performance of lithium-ion batteries is fundamentally tied to their component materials. Understanding each part's role enables better innovation in energy storage solutions.
Traditional Anode Materials
Traditional anode materials play a vital role in the functioning of lithium-ion batteries. They have been extensively used for many years and provide a foundation for understanding newer materials. These materials offer consistent performance metrics and are widely available, making them a reliable choice for many applications. However, they also come with their own set of challenges, prompting the exploration of alternative options. Understanding the fundamental characteristics, performance, and limitations of traditional anode materials will enhance our insight into the advances and challenges currently facing the field.
Graphite as a Primary Anode Material
Structure and Characteristics
Graphite is the most prominent anode material in lithium-ion batteries. The structure of graphite consists of layered carbon atoms arranged in a hexagonal lattice. This structure allows lithium ions to intercalate between the layers during battery charging and discharging. One of the key characteristics of graphite is its high electrical conductivity, which greatly aids in efficient charge transport. Its well-known stability contributes to the overall performance and lifespan of the battery. However, one notable disadvantage is that it has a limited theoretical capacity of about 372 mAh/g, which constrains its performance in high-energy applications.
Performance Metrics
When considering performance, graphite anodes demonstrate excellent charge-discharge cycle stability. Graphite maintains a reliable voltage profile and good rate capability under conditions of moderate demand. One of the main reasons for its continued use is its ability to provide high efficiency for energy storage. It also exhibits minimal volume expansion during cycling, which is beneficial for long-term durability. Nonetheless, the finite capacity limits its effectiveness as technology pushes towards batteries that demand higher capacity outputs.
Limitations
Despite its advantages, graphite has significant limitations that must be addressed. Its low theoretical capacity limits the energy density of lithium-ion batteries. Additionally, as energy requirements of applications increase, the performance of graphite anodes may not scale sufficiently. There is also a challenge pertaining to the charging rates; graphite can experience sluggish kinetics when high charging rates are required. These limitations prompt research into alternative materials that can overcome such restrictions and improve battery performance.
Alternative Carbon-Based Materials
The search for alternatives to graphite has given rise to other carbon-based materials such as hard carbon, soft carbon, and graphene. Each of these materials offers unique properties that could enhance the performance of lithium-ion batteries.
Hard Carbon
Hard carbon can serve as an effective anode material due to its unique disordered structure, which allows for higher lithium ion storage capacity compared to graphite. The key characteristic of hard carbon is its ability to absorb lithium ions in a non-intercalated manner, leading to a theoretically higher capacity. This material performs well at high charge rates, making it suitable for applications where fast charging is essential. However, the challenge lies in its less stable cycling performance compared to graphite, affecting its longer-term usage.
Soft Carbon
Soft carbon exhibits a more ordered structure compared to hard carbon, leading to different electrochemical characteristics. It has good electrical conductivity and thus can provide decent performance in lithium-ion batteries. The key advantage of soft carbon is its ability to provide a balance between capacity and cycle stability. However, it lacks some of the high-capacity benefits seen in other anode options, limiting its overall competitiveness.
Graphene
Graphene is recognized for its exceptional electrical conductivity and high surface area, allowing for high lithium ion storage potential. Its unique single-atom thickness offers mechanical strength as well as excellent thermal and electrical properties. This material's potential to be used in lithium-ion batteries contributes to significant discussions within the field. However, the challenges surrounding production and scalability of graphene still hinder its widespread commercial use, emphasizing the need for ongoing research into practical applications.
As we continue to explore and optimize anode materials, understanding the strengths and weaknesses of traditional and coming materials will inform the future of battery technology.
Emerging Anode Materials
Emerging anode materials are a critical focus in the development of lithium-ion batteries. They promise to enhance battery performance, energy density, and overall efficiency. Traditional materials like graphite face limitations that emerging materials aim to overcome. For instance, many new materials show potential for higher capacity and faster charging times. Understanding these advancements is vital for realizing the next generation of energy storage systems.
Silicon-Based Anodes
Silicon Nanoparticles
Silicon nanoparticles have emerged as a promising alternative to traditional anode materials, primarily due to their high theoretical capacity for lithium storage, 4200 mAh/g, which is significantly higher than that of graphite. Their small size contributes to improved electrochemical performance. Their structure allows for rapid lithium-ion diffusion and a larger surface area, which can enhance the overall efficiency of a lithium-ion battery. However, silicon nanoparticles experience significant expansion and contraction during charge and discharge cycles. This unique feature can lead to mechanical instability, resulting in material degradation. Engineers and researchers are investigating methods to mitigate these issues, making silicon nanoparticles an increasingly popular choice in the field.
Silicon-Carbide Composites
Silicon-carbide composites combine the high capacity of silicon with the structural integrity of carbide materials. This composite exhibits better mechanical properties than silicon alone, and it addresses some challenges related to cycling stability. The composites maintain structural integrity during battery operation, thus minimizing degradation. This characteristic makes silicon-carbide a beneficial option for anodes in lithium-ion batteries. Testing has shown that these composites often display improved charge/discharge rates, making them an attractive choice for applications requiring rapid energy release. Each of these features contributes to their growing interest in research and potential commercial applications.
Challenges and Solutions
Developing emerging anode materials, such as silicon-based compounds, requires navigating several challenges. A significant issue includes volumetric changes occurring during charge cycles. Solutions being explored involve material engineering techniques, such as the creation of composite structures or surface coatings to enhance durability. The flexibility in design allows for tailored solutions to specific performance issues. Such engineering is pivotal for integrating silicon materials into commercial battery systems. Addressing these challenges is crucial to fostering both performance and lifespan in next-generation batteries.
Metallic Anodes
Lithium Metal Anodes
Lithium metal anodes present another high-capacity option, with theoretical capacities of 3860 mAh/g. Their potential for achieving high energy density makes them a focal point of current research. This type of anode can drastically improve the overall efficiency of lithium-ion batteries. Nevertheless, safety concerns arise from the risk of dendrite formation during cycling, which can lead to short-circuits and battery failures. Balancing the performance benefits with safety is a key aspect of ongoing research.
Tin-Based Alloys
Tin-based alloys also represent a noteworthy advancement in anode materials. They offer a decent capacity, around 990 mAh/g. Unlike pure tin, tin-based alloys can provide improved cycling stability by minimizing the strain caused by high capacity. This results in longer life for battery systems. Tin's unique properties make it a popular choice, especially when mixed with other materials to enhance performance. However, like other emerging materials, the trade-off between capacity and stability needs careful consideration.
Performance Considerations
When it comes to performance, different anode materials present distinct characteristics. Factors such as charge/discharge rates, cycling stability, and temperature range significantly influence their practical application. Research is essential to establish optimal conditions and configurations to maximize these performance metrics. Understanding each material's limitations informs the best use cases, ensuring that energy storage systems operate at peak efficiency.
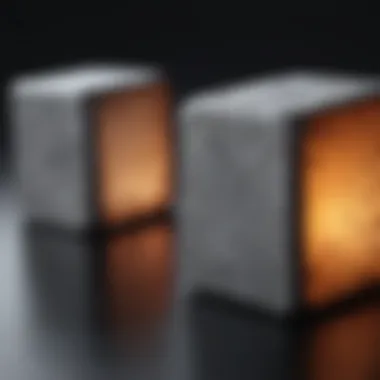
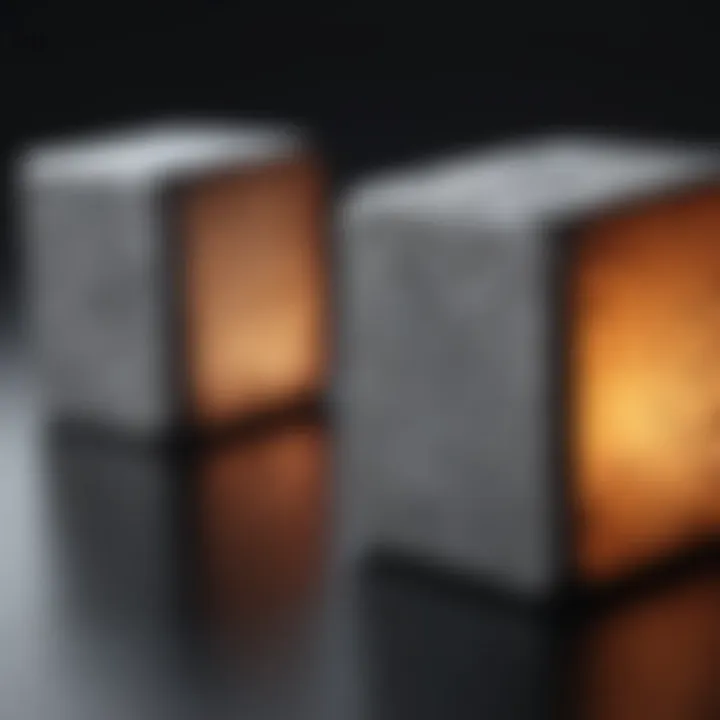
Conductive Polymer Anodes
Conductive polymer anodes are also gaining attention in the field of lithium-ion batteries. Their inherent conductivity facilitates electron transfer, which is essential for battery operation. These polymers can provide flexibility and lightweight properties that are favorable in various applications. Still, researchers are addressing their relatively low capacity and stability compared to traditional materials. Innovations in polymer chemistry are likely to enhance their effectiveness, opening up new avenues for energy storage solutions.
Characterization Techniques for Anode Materials
Understanding the characterization techniques for anode materials is crucial in the development and optimization of lithium-ion batteries. These techniques provide insights into the electrochemical properties and structural characteristics of the materials. A comprehensive analysis of anode materials can help in identifying their strengths and weaknesses, guiding researchers toward more effective designs and applications.
The techniques employed for characterization serve essential roles in assessing material performance. They reveal important data on efficiency, capacity, and the overall stability of anodes. More specifically, they aid in understanding how these materials interact with electrolytes and affect battery performance under various conditions.
Additionally, proper characterization is necessary for advancing sustainability efforts in battery technology. With ongoing research into recycling and reuse, knowing the properties of various materials can align with environmental goals.
Electrochemical Characterization
Electrochemical characterization techniques are vital for determining the performance metrics of anode materials. Among these, cyclic voltammetry and charge-discharge testing stand out as particularly important methods.
Cyclic Voltammetry
Cyclic voltammetry is an electrochemical technique that allows for the exploration of the redox behavior of anode materials. This method works by cycling the potential of an electrode and measuring the resultant current. One of its key characteristics is its ability to provide insights into kinetic parameters and thermodynamics of lithium-ion intercalation.
Cyclic voltammetry is a beneficial choice in this context because it helps researchers quantify the electrochemical properties of new anode materials efficiently. The technique is also relatively simple to execute and can be adapted for various materials. Its unique feature lies in the fact that the data collected can help assess reaction mechanisms and diffusion rates.
However, cyclic voltammetry does have drawbacks, like not providing direct insights on the capacity under practical conditions. Interpretations may vary based on experimental setup, which could lead to inconsistent results across studies.
Charge-Discharge Testing
Charge-discharge testing evaluates the actual performance of anode materials in simulated real-world conditions. This method involves cycling a battery through charge and discharge cycles to measure capacity and energy efficiency. The key characteristic of charge-discharge testing is its reflection of how materials would perform in practical applications.
This testing is a popular method within the context of characterizing lithium-ion battery anodes. It provides direct and essential data regarding the maximum capacity and cycle life. A unique feature of charge-discharge testing is its ability to simulate prolonged usage over many cycles, revealing long-term behavior that may not be reflected in other assessments.
Yet, it is important to note that charge-discharge testing often requires more time and resources. Results can be influenced by external factors such as temperature and the specific design of the testing apparatus.
Structural Analysis Methods
Understanding anode material structures is equally important for their characterization. Structural analysis methods such as semi- and full-automatic microscopy, as well as X-ray diffraction, have emerged as key tools for detailed examination.
Semi- and Full-Automatic Microscopy
Semi- and full-automatic microscopy techniques extensively analyze the microstructure of anode materials. These methods provide high-resolution imaging, allowing for the examination of surface features and morphology. One of the key characteristics of these microscopy methods is their ability to produce detailed images efficiently.
This method is beneficial because it enables researchers to observe surface characteristics and defects that might directly impact electrochemical performance. The unique element of this technique is its relatively fast data acquisition and analysis.
However, one disadvantage of microscopy techniques is the cost associated with high-resolution equipment. Moreover, interpretation of microscopic images can sometimes require expert knowledge to correctly assess what is seen.
X-Ray Diffraction
X-ray diffraction serves as a fundamental technique for analyzing the crystalline structure of anode materials. By measuring the scattering of X-rays as they interact with the crystal lattice, it provides precise information about phase composition and crystallinity. A key characteristic is its ability to determine the structural properties with high accuracy.
The relevance of X-ray diffraction in this article stems from its widespread use in materials characterization. It is effective in identifying phase transitions, which is vital for understanding how materials will behave during lithium-ion intercalation.
A unique feature of this method is its non-destructive nature, allowing materials to be analyzed without altering their properties. However, a limitation is that the technique may have difficulty analyzing amorphous materials or those with complex structures.
Performance Optimization of Anode Materials
Performance optimization of anode materials is crucial in enhancing the overall efficiency of lithium-ion batteries. This topic delves into various strategies that can improve the function and longevity of anodes, leading to better energy storage solutions. By optimizing anode performance, one can address key issues such as capacity degradation, charge and discharge rates, and thermal stability, thus ensuring a safer and more reliable battery operation.
In the context of this article, the focus on performance optimization reveals the necessity for advanced materials that can withstand rigorous cycling without significant loss in efficiency. Various approaches are being researched, including material engineering and understanding electrolyte interactions, each contributing to the ultimate goal of creating batteries that are not only high-performing but also sustainable.
Material Engineering Approaches
Nanoengineering
Nanoengineering refers to the manipulation of materials at the nanoscale to enhance their properties. This technique offers several advantages in the development of anode materials. One key characteristic of nanoengineering is its ability to increase the surface area of the anode, which facilitates better lithium ion diffusion. As a result, batteries can achieve higher capacity and charging rates.
Moreover, the small particle size often leads to improved cycle stability. However, the production of nanoscale materials can involve complex processes, which may result in higher costs. Careful control of these production methods is necessary to ensure that the benefits outweigh the drawbacks.
Composite Approaches
Composite approaches involve combining different materials to create anode structures that leverage the strengths of each component. This strategy is popular for its ability to balance conductivity, mechanical strength, and electrochemical performance. The unique feature of composite materials is that they can produce synergies that single-phase materials cannot achieve.
For instance, combining silicon with carbon can improve the stability and capacity of silicon-based anodes while mitigating issues related to silicon's volume expansion during cycling. However, the complexity of the composites can introduce challenges in processing and optimization. Ensuring compatibility between different materials in the composite is vital for maintaining reliable battery performance.
Electrolyte Interactions
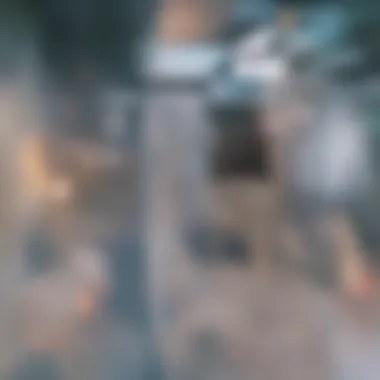
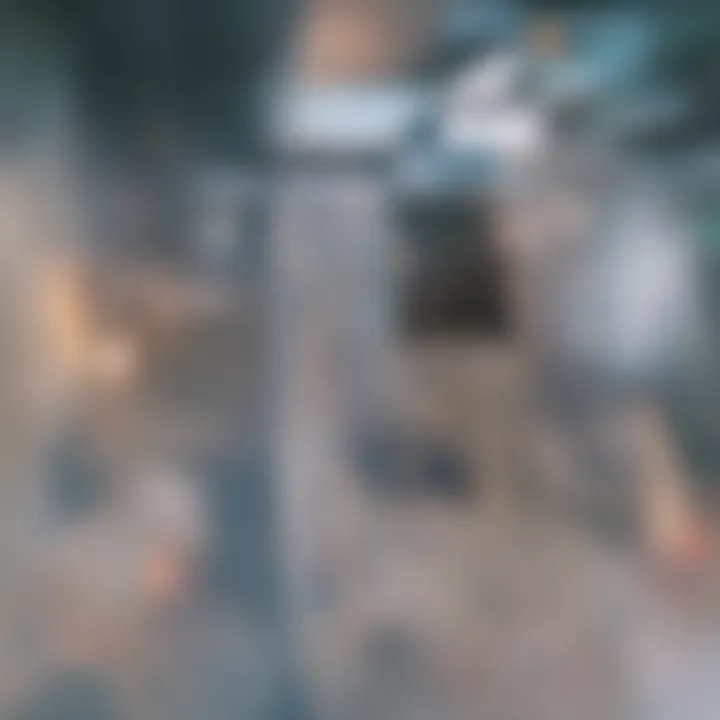
Electrolyte interactions play a significant role in anode performance. The interface between the anode and electrolyte can significantly affect charge transfer dynamics and overall battery efficiency. Understanding how different anode materials interact with various electrolytes is crucial for optimizing cell performance.
Careful selection of electrolytes can enhance lithium-ion conductivity and minimize detrimental side reactions. As an example, solid-state electrolytes are gaining interest for their potential to improve safety and energy density. Moreover, ongoing research is focused on developing new electrolyte formulations that can further enhance the stability and performance of next-generation anodes.
By addressing these various aspects of performance optimization, researchers and developers can move closer to achieving the ideal specifications for lithium-ion batteries, thus fostering developments in the energy storage sector.
Sustainability and Recycling Aspects
As the demand for lithium-ion batteries increases, particularly in electric vehicles and renewable energy systems, the need for sustainable approaches becomes critical. The sustainability and recycling aspects of anode materials play a vital role in minimizing environmental impact and ensuring resource efficiency. Analyzing these factors can help improve the overall lifecycle of batteries, from production to disposal.
Environmental Impact of Anode Materials
The environmental footprint of anode materials is a pressing concern. Traditional anode materials like graphite, while widely used, have associated extraction and processing impacts. Mining for graphite and other materials can lead to habitat destruction, pollution, and excessive energy consumption. The production process often emits greenhouse gases, thus contributing to climate change.
Emerging materials, such as silicon and tin, also present environmental challenges. Although these materials can significantly enhance battery performance, their extraction and production processes can create waste and energy demands that may offset their benefits. There is an urgent need to evaluate the full life cycle of these materials to understand their net environmental impact.
Additionally, the sourcing of materials, such as cobalt and lithium from mining operations, can result in human rights violations and community displacement. Thus, understanding the ethics behind sourcing is crucial for a holistic sustainability approach.
"The transition to sustainable battery technologies must involve not only cleaner extraction processes but also materials that can be recycled effectively."
Recycling and Reuse Strategies
Recycling initiatives for anode materials are becoming increasingly important. As lithium-ion batteries reach the end of their life cycle, effective recycling methods are critical for recovering valuable materials. These strategies can significantly reduce energy consumption and raw material demand while minimizing waste.
- Mechanical Recycling: This process often involves shredding the batteries to separate their components. The anodes can then be processed to extract reusable materials, such as graphite.
- Hydrometallurgical Processes: This method utilizes chemical solvents to dissolve specific materials, allowing for more targeted recovery of metals and other components. It provides the ability to reclaim high-purity materials.
- Direct Recycling: Research is advancing on methods that allow for the direct reuse of anode materials without extensive processing. This can lead to lower operational costs and resource use.
- Design for Recycling: Future anode development should take recycling into account from the design phase. This involves creating materials that can be easily separated during processing, ensuring that their components retain value after use.
As the battery market expands, integrating recycling strategies into the overall lifecycle of anode materials ensures a more sustainable future.
In summary, addressing the sustainability issues associated with anode materials for lithium-ion batteries is essential. Weighing environmental impacts alongside recycling strategies can lead to more responsible production and consumption cycles.
If you would like more information on battery recycling, please visit Wikipedia or check out various forums on Reddit for community opinions.
Future Directions in Anode Research
The exploration of future directions in anode research is crucial for the advancement of lithium-ion battery technology. As energy storage demands grow in various applications, enhancing the performance and sustainability of anode materials takes center stage. The emerging challenges necessitate innovative solutions that can support the ongoing transition to a more electrified world.
Researchers and industry professionals must navigate various elements such as efficiency, longevity, recycling, and environmental impact. It is essential for future studies to focus on optimizing material properties and addressing practical concerns such as manufacturability and cost. Advances in this area can significantly influence the broader implications for battery technologies and renewable energy adoption.
Innovations on the Horizon
Emerging technologies in the realm of anode materials are positioned to redefine the performance capabilities of lithium-ion batteries. Innovations include the development of advanced silicon-based anodes, where silicon's high theoretical capacity for lithium storage has captured significant attention. However, challenges such as volume expansion during charge and discharge cycles remain critical hurdles. Research into novel composites and hybrid structures aims to mitigate these issues while maximizing capacity and cycle stability.
Other innovations, such as the integration of conductive polymers and metallic anodes, promise enhanced conductivity and safety profiles. Furthermore, the application of artificial intelligence in material discovery and optimization has the potential to accelerate the identification of suitable candidates for future batteries.
"The next generation of anode materials will be a key player in improving battery performance and sustainability."
Potential Market Trends
The market for lithium-ion batteries continues to expand, driven by the growth of electric vehicles, renewable energy systems, and consumer electronics. Future trends indicate a marked shift towards higher energy densities and faster charging times. Anodes must evolve in response to these market demands, coupling performance improvements with sustainability considerations.
Here are key trends expected in the near future:
- Increased Focus on Sustainability: As environmental regulations tighten, researchers will prioritize eco-friendly materials and recycling solutions for anode production.
- Growth of Silicon-Based Anodes: The shift towards silicon will likely accelerate as effective mitigation strategies for expansion are developed.
- Innovative Fabrication Techniques: Advanced manufacturing methods, such as 3D printing, will enhance precision and customization of anode architectures.
- Standardization and Integration: There is a movement towards standardizing anode materials across different battery applications to foster interoperability and reduction in costs.
The combination of these elements will shape the future landscape of anode materials, making significant contributions to the ongoing evolution of energy storage technologies.
Closure
The conclusion serves as a critical summation within this article, providing final insights on the advances and challenges associated with anode materials in lithium-ion batteries. As energy storage technology becomes increasingly prominent, understanding the nuances of anode materials is essential for optimizing battery performance and sustainability.
In summary, we see that key findings include a clear identification of the strengths and weaknesses of various anode materials. Traditional materials, such as graphite, although widely used, exhibit limitations that may hinder performances in high-demand applications. On the other hand, emerging materials such as silicon-based and metallic anodes hold promise, yet they also present distinct challenges that need resolution.
Benefits of Understanding Anode Technology
- Enhanced Battery Efficiency:
Analyzing the electrochemical properties of anode materials can lead to breakthroughs in energy density and charge-discharge cycles. - Sustainable Practices:
A detailed evaluation of materials promotes sustainable development through recycling and responsible sourcing. - Market Innovations:
Such insights are invaluable in anticipating market trends and guiding research directions in battery technology.
"As we navigate the future of energy storage, the evolution of anode materials will continue to shape technology in unexpected ways."
Overall, this article paints a comprehensive picture of anode materials in lithium-ion batteries. By assessing both the current landscape and future possibilities, we equip researchers, students, and industry professionals with the knowledge necessary to address existing challenges and leverage upcoming opportunities in this dynamic field.
Summary of Key Findings
This article highlighted several significant aspects regarding anode materials:
- Traditional graphite remains a staple but faces limitations in capacity and cycle life.
- Alternative carbon-based materials, like hard carbon and graphene, show potential to address some of these shortcomings.
- Silicon and metallic anodes offer substantial capacity improvements; however, they have durability and manufacturing challenges.
- Various characterization techniques are essential to effectively evaluate the performance of new materials and ensure they meet required standards.
Final Thoughts on Anode Development
Moving forward, the development of anode materials symbolizes a convergence of science, engineering, and sustainable technology. Focused research and innovation in this area can lead to drastic improvements in lithium-ion battery efficacy, making them more useful in everyday applications as well as large-scale energy solutions. Bridging the gap between these advanced materials and practical applications will be vital for future technologies, thus necessitating ongoing dialogue among researchers and professionals.