Examining Carbon Dioxide Levels and Climate Impact
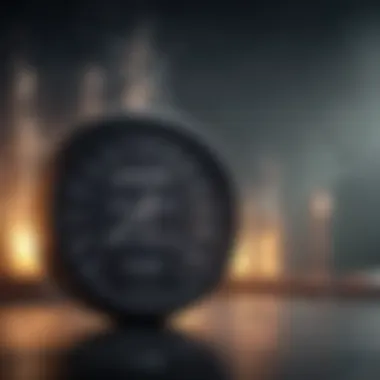
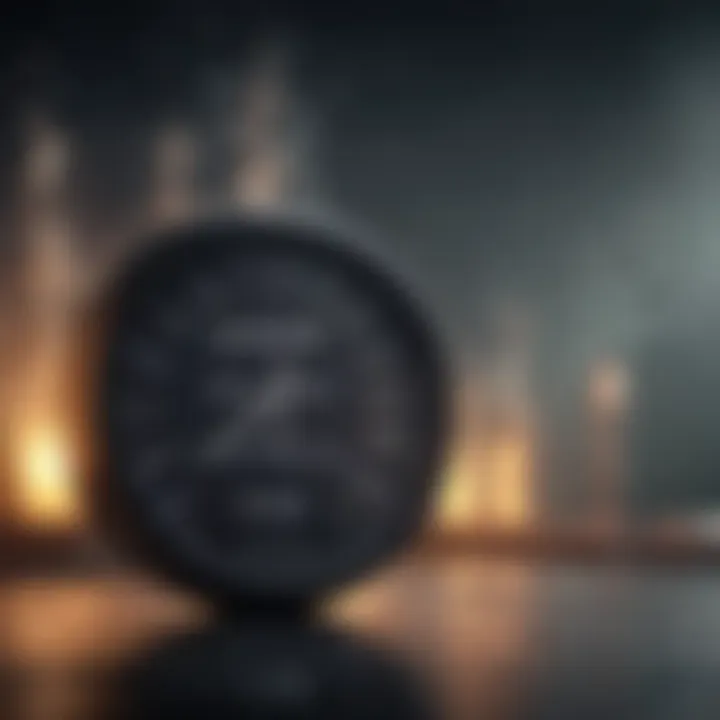
Intro
As climate change continues to dominate global discussions, understanding carbon dioxide (CO2) levels in our atmosphere becomes increasingly essential. CO2 is not just a statistic; it's a significant player in the unfolding drama of our planet’s health. This article delves into the multiple facets of atmospheric CO2, unpacking historical trends, the methodologies behind its measurement, and the impact of human activities alongside natural occurrences.
We will look at how human practices, like industrialization and deforestation, have nudged CO2 levels upward, while also identifying natural processes that influence atmospheric concentrations. In acknowledging these layers of complexity, we aim to clarify how intertwined these elements are, shaping policies and future research directions.
Moreover, understanding CO2 levels is not just about tracking numbers; it is inherently connected to environmental health and public welfare. The implications of this atmospheric component extend across ecosystems, economies, and society at large. Let’s dive into this intricate subject, unraveling the threads of scientific inquiry and the urgent need for informed action.
Research Methodology
Description of Research Design and Approach
To grasp the atmospheric levels of carbon dioxide, various methodological frameworks have been employed. A combination of longitudinal studies and cross-sectional analyses provides a comprehensive view. Researchers have typically relied on established databases such as those from NOAA and NASA, which meticulously collect and maintain records of atmospheric CO2 measurements collected from various global sites.
These datasets have been invaluable, offering historical context and real-time data that inform researchers and policymakers alike. Moreover, utilizing advanced technologies, such as remote sensing and ground-based observations, helps to quantify the variations in CO2 concentrations with high precision.
Materials and Methods Used in the Study
The materials and methods used to study CO2 levels generally include:
- Spectrometers: Used to measure the concentration of gases in the atmosphere, with high accuracy and sensitivity.
- Air Sampling Equipment: Devices range from simple canisters to sophisticated stations that constantly oxidize air and analyze its makeup.
- Computational Models: Models simulate the interactions between CO2 and other greenhouse gases, predicting future trends based on current data.
Data Collection Points
Research typically centers on specific regions that are representative of global trends:
- Remote islands, free from industrial influence, which provide baseline levels.
- Urban locations, where vehicular emissions might skew data, but are also critical for understanding pollution dynamics.
- Forested areas, where natural processes can counterbalance human-induced changes.
As we scrutinize these data points, it's essential to remember that atmospheric CO2 trends are not merely numerical; they reflect deep-seated ecological and societal issues.
Discussion and Interpretation
Interpretation of Results in the Context of Existing Literature
Results from atmospheric studies have consistently shown a steady increase in CO2 levels over the decades, aligning with findings from various research bodies around the world. The relationship between CO2 concentrations and global temperature rise, often highlighted in climate literature, underpins many environmental models.
Furthermore, historical data illustrates a stark rise post-Industrial Revolution, underscoring the impact of human activity. Mitigating this increase calls for more than just acknowledgment; it necessitates action.
Implications for Future Research or Practical Applications
The intricate understanding of CO2 levels opens avenues for significant effects—both for future research and practical policy implementations. Future efforts could focus on the following:
- Innovative Measuring Techniques: Improving technologies for better precision in data collection.
- Longitudinal Studies: Continued monitoring for long-term impacts of CO2 reductions in various environments.
- Public Awareness Campaigns: Educating populations about human behaviors that exacerbate CO2 levels.
Prelims to Carbon Dioxide in the Atmosphere
Carbon dioxide (CO2) holds a prominent position in discussions surrounding environmental science and climate change. Its significance cannot be overstated, as it plays a crucial role in both natural processes and human activities. Understanding CO2 is fundamental to grasping the complexities of the greenhouse effect, which ultimately affects global temperatures and climate patterns.
To start with, carbon dioxide is not just a mere byproduct of human industry; it is an essential component of the Earth's atmosphere. Through photosynthesis, plants convert CO2 into oxygen, serving as the foundation of life for countless organisms. This duality of CO2, being both vital for life and a potential climate disruptor, lends itself well to an in-depth examination.
Defining Carbon Dioxide
Carbon dioxide is a colorless, odorless gas that is naturally present in the atmosphere at trace levels. Its chemical formula, CO2, reveals that it comprises one carbon atom and two oxygen atoms. This simple structure, however, belies its powerful influence on the Earth’s climate system. The gas is primarily produced through natural processes such as respiration and volcanic eruptions, as well as anthropogenic activities like fossil fuel combustion.
Comments about its characteristics often focus on its role as a greenhouse gas. When sunlight reaches the Earth, some of it is absorbed and warms the surface. Carbon dioxide, along with other greenhouse gases, traps some of this heat, creating a warming effect that is vital for maintaining life as we know it. Yet, when levels of CO2 increase due to human actions, this natural balance is disrupted, leading to climate-related challenges.
Historical Overview
The history of carbon dioxide in the atmosphere can be traced back millions of years. At that time, levels of CO2 varied significantly due to natural processes. For instance, during the Paleozoic era, CO2 concentrations were much higher than today, contributing to a significantly warmer climate.
As we entered the Industrial Revolution in the late 18th century, things began to take a dramatic turn. The burning of coal, oil, and gas became prevalent, leading to an exponential rise in CO2 levels. From about 280 parts per million (ppm) before the Industrial Revolution, atmospheric CO2 surged to over 400 ppm by the early 21st century. This timeline highlights a clear correlation between human activity and greenhouse gas concentrations.
To encapsulate this section, the intricate relationship between carbon dioxide levels and climate must be understood through historical context and natural definitions. Discovering how CO2 levels have changed over time help researchers assess potential future trends. As we delve into the subsequent sections, it becomes imperative to examine measurement techniques and the factors driving these changes.
"As the world changes, so too must our understanding of the elements that shape our environment. Only through informed analysis can we hope to tackle the challenges ahead."
In summary, the study of carbon dioxide is not just an academic exercise but a necessity for shaping policies and practices that aim to mitigate climate change. Understanding where we've been and where we stand today is crucial for planning our sustainable future.
Measurement of Atmospheric CO2 Levels
Measuring atmospheric CO2 levels is fundamental to our understanding of climate change and its far-reaching effects. This measurement provides critical insights into the ongoing changes in our environment, serving as a solid basis for both scientific research and public policy. The benefits of accurately measuring CO2 levels cannot be overstated; it allows scientists to identify trends, analyze fluctuations, and predict future scenarios. By quantifying carbon dioxide, we create a clearer picture of how human activities and natural processes interact. The techniques used, as well as the extensive networks in place, play a pivotal role in achieving this understanding.
Techniques and Technologies
Satellite Observations
Satellite observations have brought a paradigm shift in our approach to monitoring CO2 levels. This method provides a global perspective that is invaluable for understanding the sprawling impact of carbon emissions across diverse geographical areas. One key characteristic of satellite observations is their vast reach—covering remote and hard-to-reach locations without the limitations of ground-based methods. This makes them a popular choice for gathering comprehensive data on atmospheric carbon levels.
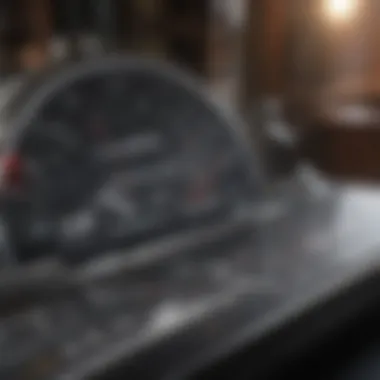
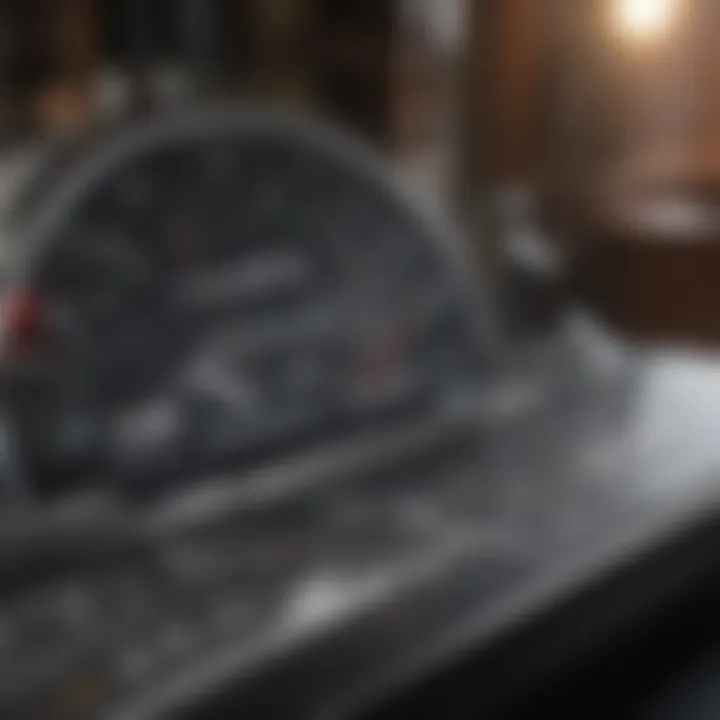
A unique feature of satellite observations lies in their ability to collect data at different altitudes, allowing for a three-dimensional view of CO2 distribution. Advantages include the ability to track changes over time and the capacity to monitor large regions simultaneously. However, there are disadvantages as well, such as limited resolution in detecting local sources of emissions and difficulties in differentiating between natural and anthropogenic CO2.
In-situ Measurement Methods
In-situ measurement methods involve collecting data at ground level, where real-time atmospheric conditions prevail. These methods contribute significantly by offering direct and precise measurements of CO2 concentrations at specific sites. Their key characteristic is accuracy— measurements obtained from these methods are often regarded as "ground truth" data, providing reliable benchmarks for satellite data.
Moreover, in-situ methods facilitate the study of local sources of carbon emissions and enhancing our understanding of regional variations. A unique feature is their adaptability; they can be deployed in various environments—urban, rural, or remote—enabling localized studies. Despite the accuracy and specificity, disadvantages might include logistical challenges, higher costs associated with maintenance, and the potential for sampling bias if monitoring stations are not well-distributed.
Data Collection Networks
Global Monitoring Programs
Global monitoring programs serve as a backbone for effective carbon tracking across the planet. These initiatives consolidate data from myriad sources and provide an extensive overview of atmospheric CO2 levels. Their key characteristic is the standardization of data collection methods across different regions, promoting consistency and comparability.
Such programs, like the Global Carbon Project, offer scientists and policymakers essential tools to understand the dynamics of carbon emissions on a larger scale. A unique feature is their extensive collaboration with various national and international bodies, enhancing data reliability. Advantages include a comprehensive perspective on global trends, while disadvantages may involve gaps in data from less monitored regions, which could lead to skewed interpretations.
Regional Monitoring Stations
Regional monitoring stations focus on localized areas and are complemented by global efforts. Their specific aspect is to provide a more granular analysis of CO2 levels, which can reveal intricate interactions between local human activities and natural phenomena. A key characteristic of these stations is their ability to draw detailed insights into how emissions vary within particular areas, whether urban or rural.
The unique feature here is the capacity to assess the effectiveness of local emissions reduction strategies, thus offering a platform for adaptive policy measures. Advantages include the immediacy of data relevant to local stakeholders, while disadvantages may encompass the limited scope of their findings in a broader context, highlighting the necessity for integration with global datasets.
Overall, both techniques and networks of measurement collectively contribute to a shared goal: understanding atmospheric CO2 levels to inform policy and global action against climate change.
Trends in CO2 Concentrations
Understanding the trends in CO2 concentrations is crucial in grasping the ramifications of this greenhouse gas on our planet. As we delve into this topic, it becomes imperative to recognize how fluctuations in atmospheric CO2 levels correlate with various factors, including human activity and natural processes. This section explores the patterns and shifts in CO2 levels, providing a detailed account of why monitoring these trends is essential for scientists, policymakers, and the general public alike.
Annual Increases in Atmospheric CO2
The pace at which atmospheric CO2 increases annually is alarming and worth scrutiny. Data from various monitoring stations reveal a consistent upward trajectory, significantly impacted by industrial activities and fossil fuel usage. For instance, a striking data point is the record level observed at the Mauna Loa Observatory, which indicated seasonal oscillations superimposed on an underlying upward trend.
The increase isn't merely a number; it has reverberations across multiple fields. From agriculture, where farmers must adapt their practices to changing conditions, to healthcare, where respiratory issues could rise due to poorer air quality, the impacts are vast.
Here are a few critical considerations regarding annual CO2 trends:
- Human Impact: Industrial outputs, deforestation, and urbanization all contribute intrinsically to the rise in CO2.
- Climate Models: Predictions based on historical data are used to project future concentration levels. Understanding these trends is vital for anticipating necessary shifts in public policy and individual behavior.
- Ambient Impacts: A rise in CO2 levels affects ocean chemistry, resulting in ocean acidification, which in turn jeopardizes marine life and ecosystems.
"We can no longer afford to ignore the escalating levels of CO2. Each year's data adds a piece to a very complex puzzle."
Seasonal Variations
The phenomenon of seasonal variations in atmospheric CO2 offers a fascinating glimpse into the interplay between natural processes and human influence. For example, CO2 levels typically experience a rise during the colder months when heating demands peak and vegetative decaying increases. In contrast, spring and summer see a decline due to enhanced photosynthetic activity.
These seasonal patterns are not just academic musings; they have practical applications and implications. Understanding these variations assists in:
- Climate Predictions: They help refine climate models for better accuracy in forecasting.
- Ecosystem Management: Recognizing how different seasons influence CO2 concentrations allows for better management of agricultural practices according to local climatic conditions.
- Policy Formulation: Policymakers can design more effective strategies for carbon mitigation by embracing a clear picture of seasonal dynamics.
In summary, tracking these trends provides a comprehensive baseline against which both natural and anthropogenic effects on atmospheric CO2 can be measured and understood. The intricate dance between human action and Earth's natural rhythm illustrates the need for ongoing observation and analytical discourse.
Human Contributions to Atmospheric CO2
The role of human activities in shaping atmospheric levels of carbon dioxide cannot be understated. It is pivotal to grasp how our daily actions and industrial practices significantly influence this greenhouse gas's concentration in the atmosphere. From driving vehicles that burn fossil fuels to the extensive clearing of forests, human behaviors contribute to the increasing levels of CO2, which in turn affect global climate patterns.
Fossil Fuel Combustion
Fossil fuel combustion stands as one of the primary culprits in the rise of atmospheric CO2. While these energy sources, such as coal, oil, and natural gas, have powered industrial advancements and everyday conveniences, they also release significant amounts of carbon dioxide when burned.
Each year, billions of tons of CO2 enter the atmosphere as a result of this combustion. Power plants, transportation, and heating systems all rely heavily on fossil fuels. In fact, according to various studies, nearly 70% of global carbon emissions come from fossil fuel use.
- Transportation: Cars, trucks, ships, and planes release vast amounts of CO2.
- Energy Production: Coal-fired plants are particularly notorious for high emissions.
- Heating: Natural gas heating systems in buildings also contribute considerably.
Addressing fossil fuel emissions is crucial for any climate change mitigation strategy. Transitioning to renewable energy sources, such as wind and solar, can provide cleaner alternatives that do not emit carbon dioxide during operation.
Deforestation and Land Use Changes
The act of deforestation and changes in land use is another major pathway through which humans contribute to CO2 levels. Forests act as significant carbon sinks, absorbing CO2 from the atmosphere. However, when these trees are cut down for agriculture, urban development, or logging, the stored carbon is released back into the atmosphere.
Key considerations include:
- Loss of Biodiversity: Deforestation often leads to habitat loss for countless species.
- Soil Degradation: Removing trees can result in soil erosion, which further impairs the land's ability to sequester carbon.
- Economic Factors: Many regions rely on deforestation for economic gain, creating a complex dilemma between development and conservation.
To combat these effects, reforestation efforts and sustainable land management practices need more focus. Restoring degraded landscapes can significantly help sequester carbon and improve biodiversity.
Industrial Processes
Finally, industrial processes contribute significantly to atmospheric CO2 levels as well. From cement production to chemical manufacturing, various industries release carbon dioxide as a byproduct of their operations. This contribution includes both direct emissions from fossil fuel combustion and indirect emissions from the energy consumed.
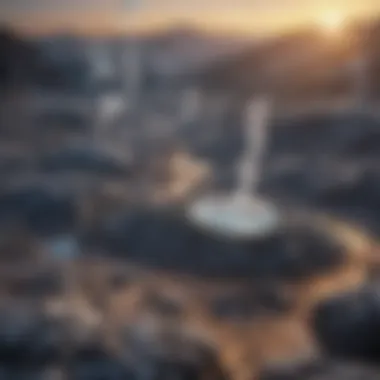
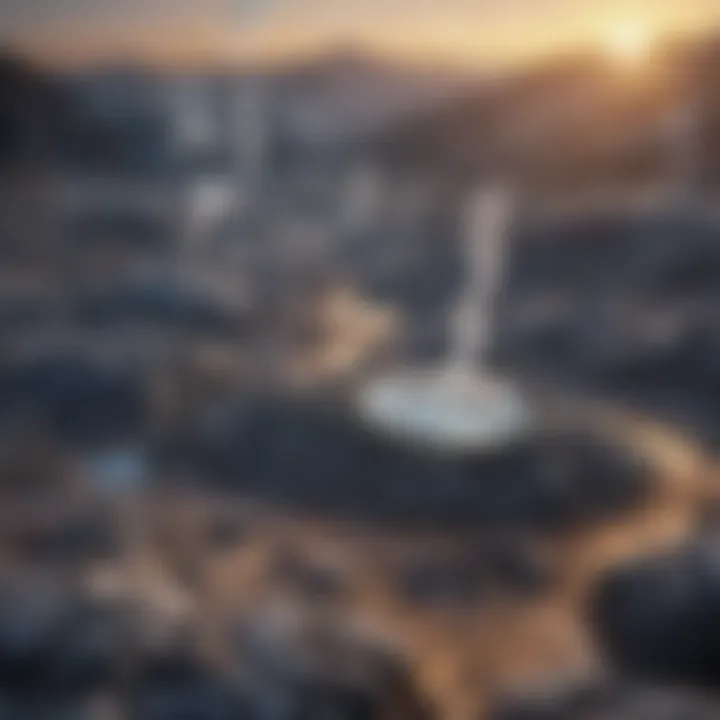
Industries particularly involved in emissions include:
- Cement Manufacturing: Producing cement involves heating limestone, which releases CO2 – approximately 0.5 tons of CO2 is emitted for every ton of cement.
- Chemical Industry: Production processes often release CO2 and other greenhouse gases.
- Waste Management: Incineration and landfilling of waste can also produce CO2 and methane, adding to the overall greenhouse gas inventory.
The path forward includes adopting cleaner technologies, enhancing energy efficiency, and investing in carbon capture methods that can mitigate these emissions.
"The vast majority of recent warming is a result of human activities, particularly the burning of fossil fuels and deforestation".
(Intergovernmental Panel on Climate Change)
Natural Influences on CO2 Levels
In the grand tapestry of Earth's climate, natural influences on carbon dioxide levels play a pivotal role. Understanding these factors is crucial, not just for grasping CO2 dynamics, but also for contextualizing human impacts. The interplay between natural processes and anthropogenic activities often shapes the atmospheric landscape of carbon dioxide. This section elucidates several key elements that contribute to changes in CO2 concentrations, cultivating a deeper appreciation for the complexity of our planet's systems.
Oceanic Absorption
The oceans, covering more than 70% of the Earth's surface, serve as significant carbon sinks, absorbing about 30% of emitted carbon dioxide. This absorption process is largely governed by ocean chemistry and temperature dynamics. As CO2 mixes with seawater, it forms carbonic acid, which alters the pH and may lead to ocean acidification. The variability in absorption rates can depend on factors like:
- Temperature: Warmer waters absorb CO2 less efficiently.
- Biological activity: Marine organisms such as phytoplankton utilize CO2 for photosynthesis, indirectly regulating CO2 levels.
- Currents: Oceanic currents can distribute CO2 differently across regions, affecting local ecosystems and carbon storage capacities.
A decline in oceanic absorption capacity could exacerbate atmospheric CO2 levels, emphasizing the delicate balance within marine ecosystems. The interdependence of biological and chemical processes in the ocean warrants significant attention, especially as climate change progresses.
Photosynthesis and Respiration
Photosynthesis stands as nature's bulwark against rising carbon levels. Through this process, green plants, algae, and certain bacteria convert sunlight, water, and CO2 into glucose and oxygen. Annually, terrestrial plants absorb approximately 123 gigatons of carbon through photosynthesis, effectively reducing atmospheric CO2. However, this beneficial act hinges on several considerations:
- Ecosystem health: Ecosystems under stress—whether from pollution, deforestation, or climate change—may be less efficient at photosynthesis.
- Plant diversity: Different species have varied capacities for carbon absorption; diverse ecosystems generally perform better.
On the flip side, respiration—a process carried out by almost all living organisms—releases CO2 back into the atmosphere. This cycle of photosynthesis and respiration is paramount for maintaining the carbon balance. Facilitating conditions for robust plant life could strengthen natural carbon sinks, thus playing a vital role in mitigating climate change impacts.
Volcanic Eruptions
While the contributions of human activity to atmospheric CO2 are undeniable, nature has its own, often dramatic methods of altering carbon levels. Volcanic eruptions contribute to CO2 emissions through the release of gases from magma. Although these events tend to be sporadic and localized, they can have significant short-term impacts on atmospheric composition. Key factors include:
- Magnitude of eruption: Larger eruptions can eject substantial CO2 quantities, influencing global climate temporarily.
- Frequency: While the average annual volcanic CO2 emissions range between 0.15 to 0.26 billion tons, irregularity makes it challenging to predict cumulative effects.
Despite these natural emissions, it's essential to note that volcanic activity is part of a larger carbon cycle that operates over geological time frames. Such natural influences remind us that Earth's systems are interconnected and ever-changing.
"Understanding the natural sources of CO2 is crucial to develop effective strategies for managing its levels and combating climate change."
Impacts of Elevated CO2 Levels
The topic of elevated atmospheric carbon dioxide levels holds significant weight in discussions about climate change and environmental sustainability. As we delve into the implications of increased CO2 levels, it's crucial to grasp the interconnections between atmospheric composition and various ecological systems. Understanding these impacts will inform policy decisions and scientific research that shape our collective response to the climate crisis.
Climate Change Effects
The rise in carbon dioxide concentrations in the atmosphere is a primary driver of climate change. As CO2 traps heat in the atmosphere, the Earth's surface temperature increases. This phenomenon is known as the greenhouse effect, and its ramifications are far-reaching. For instance, rising temperatures are linked to several adverse outcomes, such as extreme weather events, rising sea levels, and the alteration of natural habitats.
- Extreme Weather: Areas previously unaffected by severe storms may face hurricanes, tornadoes, and floods. These events can lead to loss of life and immense economic damage.
- Rising Sea Levels: As polar ice melts due to warming, oceans expand, threatening coastal communities with increased flooding.
- Altered Ecological Systems: Species that cannot adapt to rapidly changing climates are at risk of extinction, leading to a loss of biodiversity.
Recognizing these effects can motivate proactive measures to curb CO2 emissions and mitigate climate change.
Ocean Acidification
Elevated CO2 levels don’t only affect terrestrial systems but have a profound impact on oceanic ecosystems as well. When CO2 is absorbed by oceans, it reacts with seawater to form carbonic acid, thus lowering the water's pH. This process, termed ocean acidification, poses a significant threat to marine life. Coral reefs, which depend on stable pH levels to maintain their structure, are particularly vulnerable.
- Coral Bleaching: As ocean temperatures rise and acidity increases, coral reefs experience bleaching, a phenomenon that can lead to massive die-offs.
- Impact on Shellfish: Many marine organisms, including mollusks and some species of plankton, rely on carbonate ions to build their shells. When acidity increases, these ions become less available, hindering their growth and survival.
The repercussions of ocean acidification could destabilize entire marine food webs, impacting the fisheries that millions depend upon for their livelihoods.
Ecosystem Disruption
Beyond the direct effects on climate and oceans, elevated CO2 levels also bring about disruptions across ecosystems. For instance, changes in temperature and carbon availability can shift the balance of plant communities, with potential cascade effects throughout the food chain.
- Shifts in Vegetation Zones: Some species may thrive in higher CO2 environments, while others may decline, leading to altered habitats.
- Invasive Species: With warmer temperatures and changing precipitation regimes, invasive species may flourish, further jeopardizing native flora and fauna.
The health of ecosystems is crucial for services they provide—from clean air and water to carbon storage and habitat for diverse species. Protecting these systems is essential for maintaining ecological balance.
"The future of our planet is heavily dependent on the decisions we make today regarding carbon emissions and environmental protection."
In summary, the impacts of elevated CO2 levels extend far beyond mere temperature increases; they intertwine with complex environmental and societal challenges that must be tackled with urgency and innovation. Understanding these dynamics is crucial for developing effective policies and solutions that address this global challenge.
Public Policy and CO2 Mitigation Strategies
Public policy plays a crucial role in shaping the approaches necessary for mitigating atmospheric carbon dioxide levels. As society grapples with the realities of climate change, effective policy frameworks can facilitate transformative shifts in both individual behavior and industrial practices. Addressing CO2 emissions not only hinges on technological innovation but also requires solid commitments from governments and institutions that affect how resources are allocated and how strategies are developed.
From international agreements to national regulations, the policies laid down will directly impact the speed and effectiveness of our response to rising CO2 levels. So, understanding the strengths and weaknesses of these strategies is imperative.
International Agreements
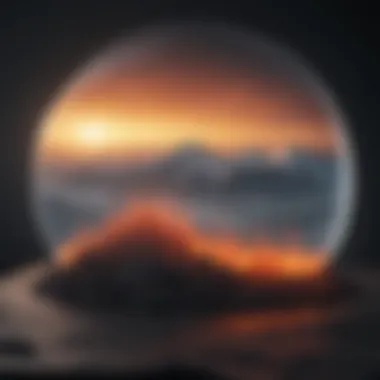
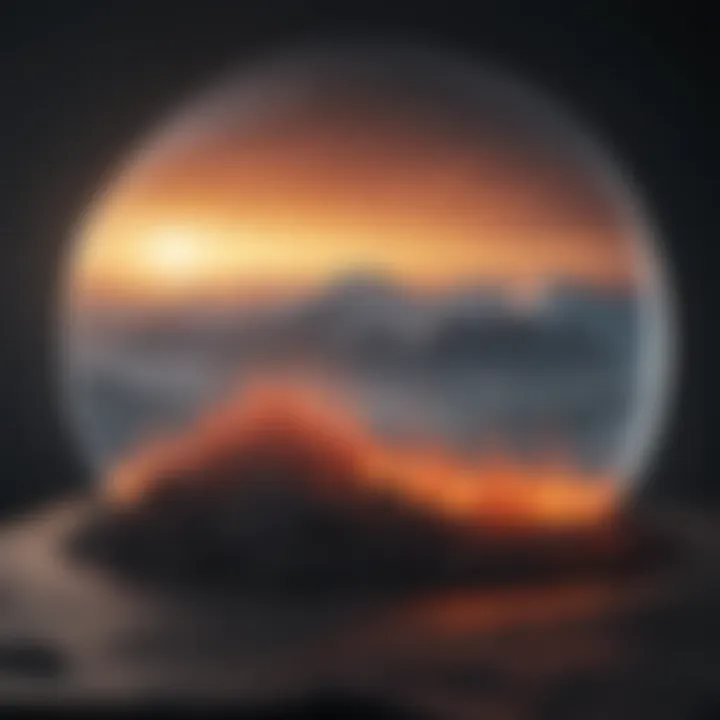
The Paris Agreement
The Paris Agreement represents a major leap towards collective efforts to combat climate change by limiting global warming to well below 2 degrees Celsius. One of its key characteristics is its emphasis on nationally determined contributions (NDCs). This approach empowers individual countries, allowing them to set their emissions reduction targets based on their specific circumstances. This feature makes the Agreement flexible, capturing the varied abilities of nations in addressing climate challenges.
A significant benefit of the Paris Agreement is its universal participation. Almost every nation on Earth is a signatory, showing a shared commitment to tackling climate change. This broad involvement fosters accountability among signatory countries. However, this inclusiveness could also lead to challenges in ensuring compliance and reaching consistent standards globally. Nonetheless, the Agreement promotes transparency, which could result in enhanced trust between countries as they share their progress.
Kyoto Protocol
The Kyoto Protocol, established earlier in 1997, marked a pioneering step in setting legally binding emissions targets for developed nations. Its key characteristic is that it focuses primarily on industrialized countries, holding them accountable for their historical contributions to greenhouse gas emissions. The crucial aspect of the Kyoto Protocol is its establishment of a cap-and-trade system, enabling nations to trade emissions permits. This provides financial incentives for countries to lower their emissions more efficiently.
The protocol’s unique feature of allowing emissions trading sets a precedent for market-based approaches to pollution reduction. While it was one of the first international agreements to address CO2 emissions legally, it also faced criticisms for its limited reach. Notably, many large developing nations were not bound by legally binding targets, which raised concerns about its overall efficacy in global greenhouse gas reduction efforts.
National Policies
Carbon Pricing
Carbon pricing is gaining traction as an effective strategy to reduce greenhouse gas emissions. By putting a price tag on carbon emissions, it aims to incentivize both individuals and businesses to opt for lower-carbon alternatives. The core characteristic of carbon pricing is its ability to internalize the environmental costs associated with CO2 emissions, thereby potentially altering consumer behavior and stimulating innovation in cleaner technologies.
One major benefit of carbon pricing is that it generates revenue, which can be reinvested in renewable energy projects or used to support communities that are heavily reliant on fossil fuels. However, this mechanism can also face significant resistance from businesses that fear it might hurt their bottom lines or lead to increased costs for consumers. The balance between incentivizing change and managing economic impact is delicate but necessary for sustainable progress.
Renewable Energy Initiatives
Renewable energy initiatives serve as a cornerstone of efforts to reduce reliance on fossil fuels and lower atmospheric CO2 levels. These initiatives are characterized by their focus on transitioning to clean, sustainable sources such as solar, wind, and hydropower. By encouraging investment in renewable technologies, these policies not only mitigate the release of CO2 but also create new job opportunities in emerging sectors.
A particular advantage of these initiatives is their potential to diversify energy sources and enhance national energy security. Countries investing in renewable energy can reduce their dependence on imported fuels and thus minimize vulnerability to fluctuating energy prices. On the downside, the initial investment in renewable infrastructure can be high, and the intermittency of certain energy sources, like solar and wind, requires supplementary strategies to ensure a reliable energy supply.
The path to effective policy and strategies against rising CO2 levels is as complex as it is urgent. A balanced approach incorporating international agreements and national policies is essential for fostering a sustainable future.
Future Projections for Atmospheric CO2
As we look ahead into the future, the significance of projecting atmospheric levels of carbon dioxide (CO2) cannot be overstated. Understanding these projections is vital for several reasons, including assessing the potential impacts of climate change, formulating effective policy, and paving the way for innovative technological developments. These projections offer insights not just into potential future scenarios, but also into the urgency of addressing the challenges presented by elevated CO2 levels.
One key aspect to consider is how climate models provide a framework for these projections. These models simulate various scenarios based on current data and trends, helping us foresee the potential trajectories of CO2 concentration under different conditions. This enables policymakers and scientists to identify the most critical areas for action and investment.
The implications of these projections stretch beyond mere numbers. They serve as a wake-up call, indicating the direction our planet is headed if current trends continue. Without intervention, rising CO2 levels could exacerbate environmental issues, intensify climate change impacts, and threaten global ecosystems. This makes understanding future projections an essential part of our strategy to mitigate adverse outcomes.
Climate Models and Scenarios
Climate models represent the backbone of projections regarding atmospheric CO2. These sophisticated models utilize a variety of data – including historical emissions, temperature patterns, and carbon sinks – to generate scenarios that outline possible futures.
There are several types of climate models, each contributing uniquely to our understanding:
- General Circulation Models: These models simulate atmospheric and oceanic movements, helping to predict climate behavior on a large scale.
- Integrated Assessment Models: They combine data from economic, social, and environmental disciplines, providing insights into the socio-economic factors that influence CO2 levels.
- Earth System Models: These are some of the most advanced, incorporating interactions among the atmosphere, oceans, land surface, and ice, refining our understanding of feedback mechanisms that impact CO2 levels.
Projections can vary significantly based on the inputs fed into these models, which often leads to multiple, sometimes conflicting scenarios. However, emerging consensus points to a few key outcomes:
The trajectory of atmospheric CO2 will depend crucially on global policies and technology advancements, alongside societal choices regarding energy production and consumption.
Technological Innovations in Carbon Capture
Technology plays a pivotal role in shaping future atmospheric CO2 levels. The development of carbon capture and storage (CCS) technologies stands at the forefront of these innovations. By actively removing CO2 from the atmosphere and securely storing it underground, these technologies can help mitigate the impact of emissions.
Several promising innovations within the field of carbon capture include:
- Direct Air Capture: This technology captures CO2 directly from atmospheric air, allowing for its removal regardless of the emission source.
- Bioenergy with Carbon Capture and Storage (BECCS): This approach combines biomass energy production with carbon capture, potentially leading to net-negative emissions.
- Advanced Materials for Capture: Research into novel materials, such as metal-organic frameworks, has the potential to enhance the efficiency and reduce the costs of carbon capture technologies.
Moreover, the integration of carbon capture solutions into existing industrial processes can significantly cut down emissions from sectors that are traditionally hard to decarbonize, such as cement and steel production. However, challenges in scalability, cost-effectiveness, and regulatory frameworks still loom large.
Thinking forward, as these technologies mature and become more widely adopted, they hold the promise of reshaping our climate trajectory. Ultimately, how we leverage these innovations will play a crucial role in determining our collective future amidst rising CO2 levels.
Research Gaps and Future Directions
Understanding the atmospheric levels of carbon dioxide (CO2) remains one of the most pressing scientific challenges today. The reasons for this urgency are manifold. First, the thickening blanket of CO2 in our atmosphere contributes significantly to climate change, thereby influencing weather patterns, sea levels, and biodiversity. Yet, while much has been studied, there is still a neec for continued investigation.
Need for Continued Monitoring
The first step toward addressing the issue is maintaining ongoing monitoring of atmospheric CO2 levels. This involves not just tracking the concentrations but also understanding the sources and sinks of CO2 in real time. A continuous stream of reliable data is essential for drawing responsive strategies against the escalating levels of this gas. Modern technology, such as remote sensing from satellites, has greatly enhanced the ability to monitor CO2. However, gaps still persist in localized measurements that can effectively inform policymakers and researchers about specific regional dynamics.
A comprehensive monitoring network could provide several benefits:
- Timely insights: Early detection of anomalies in CO2 levels can help in better forecasting future conditions.
- Informed decision-making: Policymakers rely on accurate data to craft effective legislation targeting emissions.
- Public awareness: Continuous data sharing can keep communities informed and engaged about the environmental health of their surroundings.
In essence, it’s about connecting the dots between observations and actions. But we can't simply rely on past models and assumptions; fresh approaches and novel techniques are desperately needed to fill in the blanks.
Interdisciplinary Research Opportunities
The complexities surrounding atmospheric CO2 levels require a multidisciplinary approach that bridges gaps across various fields. Geosciences, meteorology, oceanography, and social sciences must work collaboratively to fully understand how human activities and natural phenomena affect atmospheric compositions.
Further interdisciplinary angles can unlock myriad research opportunities. For instance:
- Integrating Technology with Ecology: Combining advanced monitoring technologies with ecosystem studies can unearth interactions between CO2 levels and biodiversity.
- Policy and Behavior Studies: Examining how public frameworks influence individual behavior towards emissions can shape both education and enforcement strategies.
- Climate Models and Human Factors: Understanding how social behaviors contribute to emissions opens a new frontier for climate modeling. If we can predict human responses to changes in CO2 levels, we can craft more adaptive climate policies.
"The need for interdisciplinary collaboration is clear; complex issues are seldom solved in silos, and atmospheric CO2 concentrations exemplify this intricacy."