Exploring Carbon Nanotube Conductivity and Its Applications
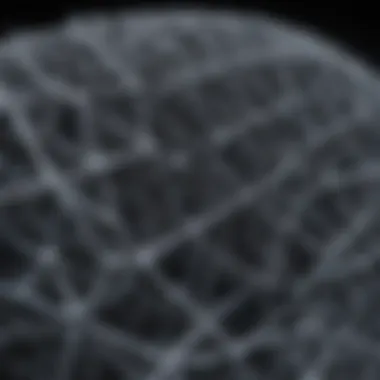
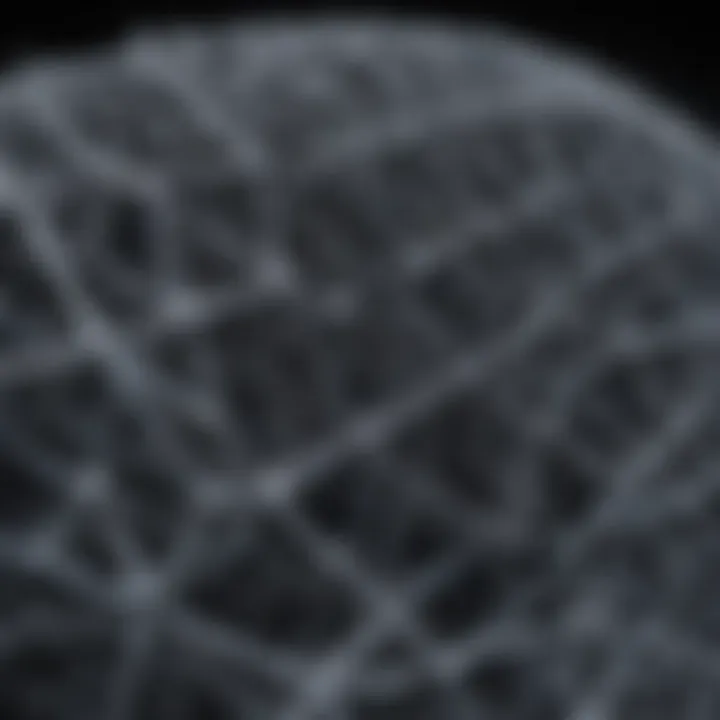
Intro
Carbon nanotubes (CNTs) have stirred up a whirlwind of interest in the scientific community and beyond, primarily due to their exceptional electrical conductivity and other unique properties. When you think of materials that challenge the norms of conductivity, CNTs sit right at the top of the list. This tiny structure offers a marvelous range of applications, from electronics to medicine, all the while providing a platform for researchers to push the boundaries of material science.
What sets CNTs apart? Their structure takes center stage. Each nanotube is essentially a rolled-up sheet of graphene—a one-atom-thick layer of carbon—forming cylindrical shapes exhibiting extraordinary mechanical, thermal, and electrical properties. The orientation and arrangement of carbon atoms dictate how well these nanotubes conduct electricity, showcasing a fascinating interplay of quantum mechanics and material science. Whether you're tinkering in a lab or studying from afar, understanding the mechanisms at play is crucial.
Diving deeper, we find that the conductivity of CNTS is not just a characteristic but a complex phenomenon influenced by myriad factors. From the chirality of the nanotubes to the surrounding environment, these elements interlace to create a tapestry that dictates how CNTs behave. With potential applications ranging from field-effect transistors to various sensors, the implications of harnessing their conductivity are profound. This article will take you on a journey through the various layers of CNT conductivity, exploring structures, mechanisms, and their wide-reaching applications.
By providing a comprehensive overview, we aim to untangle the intricate details surrounding this advanced material. Whether one is a student eager to learn or a researcher focused on the cutting-edge of materials science, this discussion seeks to foster a richer understanding of carbon nanotubes and their impressive conductive properties.
Prelude to Carbon Nanotubes
Understanding carbon nanotubes (CNTs) is essential as they represent a remarkable advancement in materials science. Their unique properties make them pivotal in various high-tech applications, such as nanoelectronics, energy storage, and composite materials. From their formation to their conductivity, every aspect holds significant implications for future technologies.
Definition and Structure
Carbon nanotubes are cylindrical structures formed solely from carbon atoms arranged in a hexagonal configuration. This arrangement gives rise to highly desirable physical properties. Each carbon atom bonds covalently with three neighboring carbon atoms, leading to the formation of strong covalent bonds that enhance the stability of the structure. Apart from their structural attributes, the ratio of length to diameter is critical, often reaching thousands:1, which composes their nanoscale identity.
The structure is fundamentally significant, as it allows CNTs to possess electrical properties that vary drastically based on their geometric configuration. Knowing the different arrangements leads us to better utilize them in applications ranging from efficient conductors to flexible materials.
Classification of Carbon Nanotubes
To grasp the vast implications of carbon nanotubes, it is crucial to classify them effectively. The primary distinction lies between single-walled and multi-walled carbon nanotubes, each possessing unique characteristics and advantages.
Single-Walled Carbon Nanotubes (SWCNTs)
Single-walled carbon nanotubes comprise a single layer of carbon atoms forming a seamless cylinder. A standout feature of SWCNTs is their exceptional electrical conductivity, which is vital for applications in electronics. Due to their uncomplicated structure, they exhibit high mechanical strength while retaining flexibility, making them popular for various nanotechnology applications.
Benefits of SWCNTs:
- High electrical conductivity: Because of their one-dimensional structure, they offer superlative conductivity.
- Lightweight and flexible: They maintain structural integrity while being adaptable.
However, their susceptibility to defects during production can hinder their performance. This can lead to inconsistency in application outcomes, making meticulous production methods crucial.
Multi-Walled Carbon Nanotubes (MWCNTs)
Multi-walled carbon nanotubes consist of several concentric cylinders of carbon atoms. This configuration enhances their overall robust mechanical properties while allowing for improved thermal and electrical conductivity. MWCNTs provide better shielding from external environmental influences due to their layered structures.
Characteristics of MWCNTs:
- Enhanced structural integrity: More walls mean they can handle greater stress, which is advantageous in composite materials.
- Versatile applications: Their robustness allows them to work well in energy storage systems.
One potential downside is their often lower electrical performance compared to SWCNTs, which can be a critical consideration in specific electronic applications. Understanding these classifications and their implications lays a solid groundwork for exploring the conductivity of carbon nanotubes in the following sections.
"Carbon nanotubes are not just materials; they are a gateway to revolutionary applications in technology and industry."
The journey through the properties, mechanisms, and practical usage of carbon nanotubes is just beginning. Each section of this article aims to delve deeper, providing clarity and insight into these fascinating structures.
Electronics and Conductivity of Carbon Nanotubes
The domain of electronics is inextricably linked to the conductivity characteristics of carbon nanotubes (CNTs). Understanding how these nanostructures conduct electricity is crucial for both technology development and scientific inquiry. The unique physical and chemical properties of CNTs, coupled with their high surface area, make them a game changer in the field of electronics. In this section, we will unravel the fundamentals of electrical conductivity, delve into the role of quantum mechanics, and ultimately highlight why carbon nanotubes deserve significant attention in contemporary research.
Basics of Electrical Conductivity
Electrical conductivity refers to the ability of a material to allow the flow of electric current. In the context of carbon nanotubes, conductivity is primarily influenced by the arrangement of carbon atoms and their electronic properties.
- Conductive Pathways: Carbon nanotubes create remarkably conductive pathways due to their cylindrical structure, ensuring electrons traverse with minimal resistance.
- High Aspect Ratio: Their high length-to-diameter ratio contributes to a low scattering rate, enabling efficient current flow.
- Comparison with Traditional Conductors: When you stack carbon nanotubes against traditional metallic conductors like copper, CNTs hold significant advantages, particularly in lightweight applications.
These elements collectively position carbon nanotubes as prime candidates for applications such as nanoelectronics, where miniaturization and efficiency are paramount.
Role of Quantum Mechanics in Conductivity
Quantum mechanics is pivotal in explaining the extraordinary electrical properties of carbon nanotubes. Unlike conventional materials, where a clear band gap delineates conductive and insulative behaviors, CNTs exhibit a more complex picture involving atomic-scale interactions. Understanding this is key to unlocking their full potential in futuristic applications.
Band Structure
- Key Characteristic: The band structure of carbon nanotubes is not only a fundamental property but also influences their conductive nature directly. Each type of carbon nanotube can be either metallic or semiconducting, depending on its chirality—the geometric arrangement of the carbon atoms.
- Benefits for Applications: This variability enables the design of specific nanotube types for different electronic applications, making CNTs highly versatile.
- Unique Feature: A remarkable aspect of their band structure is the absence of a traditional band gap in metallic nanotubes, which allows for extremely high conductivity.
In essence, their band structure is a beneficial cornerstone for utilizing carbon nanotubes in nanotechnology and electronics.
Electron Mobility
- Defining Characteristic: Electron mobility in carbon nanotubes is influenced by the quantum confinement effects arising from their nanostructured state.
- High Mobility Advantage: CNTs demonstrate extraordinarily high electron mobility, meaning electrons can shift from one atom to the next without encountering significant obstacles. This ensures swift current transportation, which is essential for high-speed electronic devices.
- Feature and Limitations: However, certain impurities or structural defects can lead to decreased mobility, introducing a factor to consider in CNT applications.
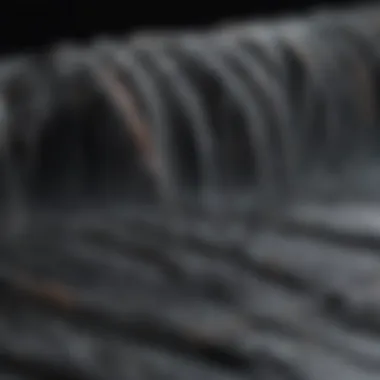
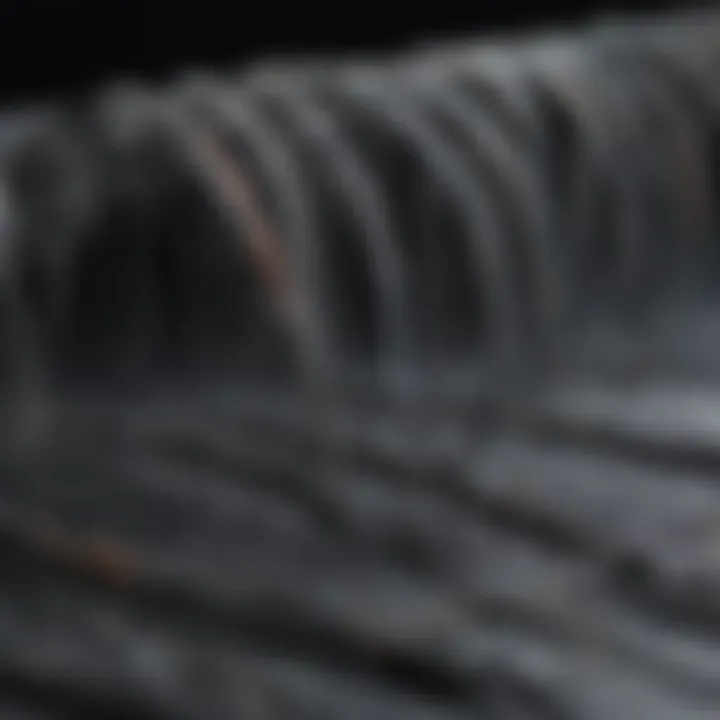
Overall, the interplay of quantum mechanics in band structure and electron mobility elucidates how carbon nanotubes can uniquely facilitate electrical conductivity. This foundational knowledge underlines their potential in creating superior electronic devices and innovative applications in technology.
Understanding these conductive properties of carbon nanotubes is not merely academic; they shape the future landscape of electronics, pushing boundaries that were once deemed impossible.
As we dig deeper, it's essential to consider the factors influencing the conductivity of these materials, paving the way for the synthesis and characterization we will explore in subsequent sections.
Factors Affecting Conductivity in CNTs
The conductivity of carbon nanotubes (CNTs) stands as a pivotal characteristic in determining their usefulness in various applications. As we peel back the layers of this complex subject, it becomes increasingly clear that several interplaying factors critically shape this property. Understanding these elements not only broadens our grasp of CNT behavior but also informs advances in technology ranging from nanoelectronics to energy storage systems.
Influence of Length and Diameter
The dimensions of carbon nanotubes, specifically their length and diameter, greatly influence their electrical conductivity. Generally, longer nanotubes tend to exhibit improved electrical properties. This stems from the idea that a longer path allows for greater chances of electron flow without scattering. When examining the diameter, it is apparent that narrower tubes can contribute to higher conductivity due to reduced electron density. However, it’s important to note that extremely thin nanotubes might introduce significant quantum confinement effects, which could potentially limit electron mobility.
In practical terms, achieving a balance between length and diameter is essential. Too broad a diameter incurs a penalty in terms of mechanical properties as well, while too narrow can lead to unexpected quantum behaviors. Researchers are constantly fine-tuning these parameters to optimize the conductivity of CNT-based materials for specific applications.
Impact of Defects and Impurities
Defects and impurities are the unsung villains in the drama of carbon nanotube conductivity. Much like a crack in the glass can hinder visibility, defects in the structure of CNTs can severely disrupt their electrical performance. These flaws can arise from various processes, such as during synthesis or from environmental exposure. Defects may introduce localized energy levels, scattering electrons and, hence, detracting from the conductivity.
Additionally, impurities — be it foreign atoms or structural irregularities — play a role in modifying the electronic properties of the CNTs. For instance, the presence of oxygen or other elements can create charge traps, which can impede free electron movement. Understanding these interactions is critical, as enhancing the purity of CNTs can lead to significant jumps in performance.
Temperature Effects
Temperature is another critical player affecting conductivity in carbon nanotubes. As temperature rises, the kinetic energy of electrons increases, usually leading to greater conductivity. However, this can come with a caveat. At elevated temperatures, increased lattice vibrations can cause scattering, thus reducing the overall electron mobility beyond a certain point. This temperature dependence can vary depending on the structural configuration of the CNTs.
Interestingly, carbon nanotubes can exhibit fascinating semiconductor characteristics at lower temperatures, where their conductivity may transition between metallic and insulating states. This phenomenon is further accentuated in systems that exploit the unique properties of some nanotubes; for instance, researchers are noting that individual CNTs display highly variable performance characteristics based on thermal conditions.
"The interplay of length, diameter, defects, and temperature illustrates not merely the nuances in conductivity but also the larger narrative of how we engage with advanced materials like carbon nanotubes."
Grasping these factors creates a composite understanding; we can appreciate that maximizing the effectiveness of CNTs isn't a mere technicality — it’s a science grounded in intricate relationships and a careful balancing act. The depth of knowledge on such matters is paramount for those working at the frontiers of materials science, as each advancement can ripple through industries and research fields, illuminating new paths for innovation.
Synthesis and Fabrication Techniques
When exploring the conductivity of carbon nanotubes (CNTs), it's crucial to understand the synthesis and fabrication techniques employed. These methods play a pivotal role in determining the quality, yield, and overall characteristics of the carbon nanotubes produced. How CNTs are synthesized not only impacts their physical structure but also their electrical and mechanical properties, ultimately dictating their applications across various fields. Hence, familiarity with these techniques enables researchers and professionals to tailor CNTs for specific uses, whether in electronics, energy storage, or composite materials.
Chemical Vapor Deposition ()
Chemical Vapor Deposition (CVD) is one of the most widely adopted methods for synthesizing carbon nanotubes. This process involves the deposition of carbon atoms onto a substrate from a gas phase, allowing for a controlled environment that promotes the growth of high-quality nanotubes. The versatility of CVD is one of its major strengths; variations like thermal CVD, plasma-enhanced CVD, and low-pressure CVD can be harnessed depending on the desired properties and configurations of the nanotubes.
The key benefits of using CVD for nanotube synthesis include:
- Scalability: CVD can be scaled up for industrial applications, making it feasible for mass production.
- Control over Morphology: Precise control of growth conditions can lead to specific morphological features, including length and diameter.
- High Purity: The process can result in high-purity products with minimal defects, essential for maintaining conductivity.
However, the technique also comes with its challenges. Achieving uniform growth across large areas can be difficult, and the costs associated with high-quality substrates may be prohibitive. Additionally, the control of parameters like temperature, pressure, and gas flow requires fine-tuning to produce CNTs with the desired characteristics.
Laser Ablation
Laser ablation presents another intriguing method for synthesizing carbon nanotubes and offers unique advantages. In this process, a high-energy laser pulse vaporizes solid carbon sources, often in the presence of a catalyst, producing a plume of carbon atoms that subsequently condense to form nanotubes. This technique is especially noted for producing multi-walled carbon nanotubes.
Some notable aspects of laser ablation include:
- Production Rate: The method can yield large quantities of CNTs within a short time frame, an appealing trait for research and industrial needs.
- High Quality: Nanotubes produced by laser ablation often exhibit fewer structural defects, which enhances their conductivity and strengthens their potential applications.
- Versatility: Different carbon sources and catalysts can be utilized, leading to variations in the properties of the resulting CNTs.
On the flip side, potential downsides include the initial investment in equipment, which can be significant. There's also the need for skilled personnel to operate and maintain the laser systems efficiently. Moreover, while the production can be fast, the actual integration of these materials into products might require additional processing and refinement.
"The synthesis method chosen has a direct correlation with the performance of carbon nanotubes. Each technique comes with its own set of advantages and limitations, demanding a careful evaluation based on the intended application."
In summary, understanding the myriad synthesis and fabrication techniques available expands the horizon for tailoring carbon nanotubes. Whether opting for CVD or laser ablation, researchers and industry professionals must consider the specific requirements of their applications to harness the full potential of CNTs.
Characterization of Carbon Nanotube Conductivity
Understanding the conductivity of carbon nanotubes (CNTs) is essential, as it paves the way for their applications in various fields. Characterization can be thought of as the fine-tuning of a musical instrument – it adjusts and enhances the qualities inherent in these materials. Accurate assessment of conductivity not only explains the functioning of CNTs but also aids in the development of new tech solutions. The uniqueness of CNTs arises from their structure which, in turn, determines their electrical properties. Delving deeper into how we characterize these materials is pivotal to unlocking their full potential.
Electrical Measurements
When it comes to gauging how well carbon nanotubes conduct electricity, electrical measurements come into play. These tests measure the ability of CNTs to transmit electrons efficiently. Some key methods used include:
- Four-Probe Measurement: A standard technique for accurate conductivity measurement. Here, four probes are arranged in a line. By applying a current through the outer probes and measuring the voltage between the inner probes, researchers can determine the resistivity of the material.
- Transmission Line Method: An innovative technique that allows for measuring the dielectric properties of CNTs by analyzing how they respond to electromagnetic waves.
- I-V Characteristics: Current-voltage (I-V) curves illustrate how current varies with voltage. This graphical representation helps in determining the semiconductor or metallic nature of nanotubes, guiding future applications.
Benefits of Electrical Measurements
Through these measurements, one can:
- Assess the quality of synthesized nanotubes.
- Optimize conditions for better conductivity.
- Compare different CNTs and align them with specific applications.
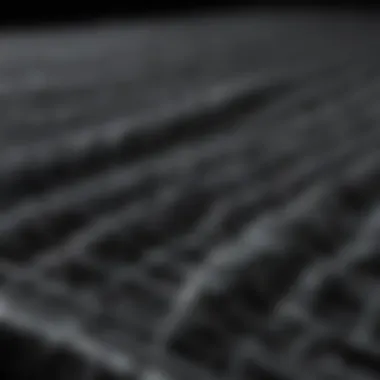
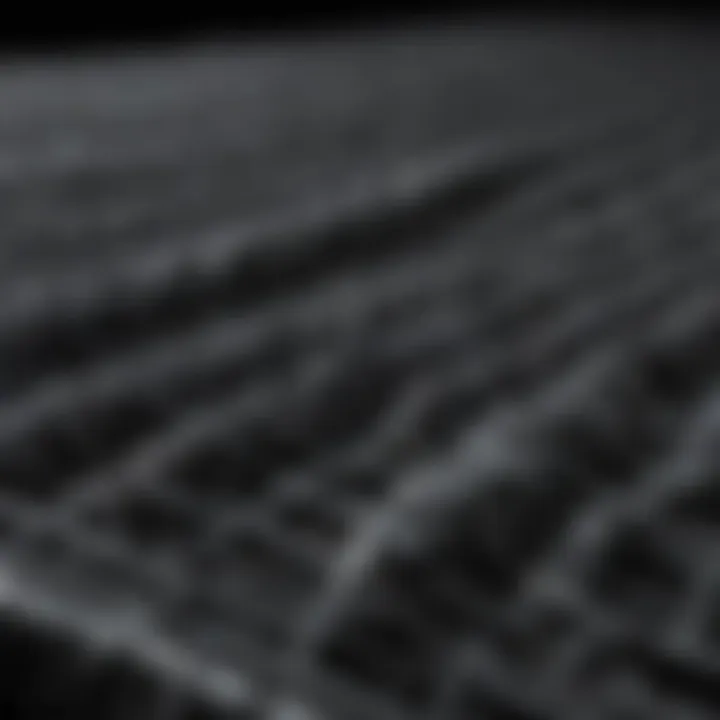
By thoroughly understanding how these measurements work, we can ensure that the properties of carbon nanotubes are effectively utilized.
Scanning Tunneling Microscopy (STM)
One of the most fascinating methods for exploring carbon nanotube conductivity is Scanning Tunneling Microscopy (STM). Imagine peering into a world where the atomic structure of a material reveals its secrets. STM allows researchers to examine surfaces at the atomic level. Using a sharp tip, it can detect electronic density variation within a carbon nanotube. This technique provides a direct insight into the electronic properties of these materials.
Key highlights of STM include:
- High Resolution: STM can achieve atomic resolution, meaning it can count the atoms on the surface of a carbon nanotube while simultaneously revealing the electronic structure.
- Conductivity Mapping: This technique allows for mapping of the local density of states, essentially showing where electrons are likely to be found.
- Material Characterization: By observing how the nanotubes interact with the scanning probe, scientists can assess defects or impurities that may impact conductivity.
As we consider these methods, it’s clear that both electrical measurements and Scanning Tunneling Microscopy offer rich insights into carbon nanotube conductivity. The combination of these techniques enables a comprehensive approach to understanding how structure and conductivity interplay in CNTs. This characterization process ultimately lays the groundwork for their various successful applications in technology and beyond.
"Characterization is not just a process; it's the key to unlocking potential in materials like carbon nanotubes."
Understanding these methodologies and the implications they have can potentially steer the direction of scientific research and application, offering a broad landscape for future advancements.
Applications in Technology
The realm of carbon nanotubes (CNTs) is increasingly intertwined with various technological innovations, reflecting their pivotal role in enhancing performance across multiple applications. This section dissects the significance of deploying CNTs in technology, focusing on their multifaceted benefits and the considerations that come alongside their utilization.
Carbon nanotubes are heralded for their exceptional conductivity and mechanical strength, marking them as game-changers in numerous fields. They serve as critical components in nanoelectronics, energy storage systems, and composite materials, catalyzing advancements that were once mere concepts. The integration of CNTs into these areas not only enhances functionality but also addresses the pressing demands for sustainability and efficiency in modern technology.
Nanoelectronics
In the fast-evolving field of electronics, carbon nanotubes have become indispensable due to their remarkable electrical properties. The capability of CNTs to conduct electricity efficiently makes them ideal for developing smaller, faster, and more efficient electronic components. Their unique one-dimensional structure allows for unparalleled electron mobility, resulting in devices that can operate at significantly higher speeds than traditional silicon-based components.
Furthermore, the flexibility and light weight of nanotubes facilitate innovative designs that push the boundaries of current technology. For instance, CNTs are being investigated for use in field-effect transistors (FETs), where their high performance could lead to quicker response times and lower power consumption.
Key Takeaways
- Enhanced Performance: CNTs increase the speed and efficiency of electronic devices.
- Innovative Design: Their lightweight and flexible nature allows for new electronic architectures.
- Future Potential: Continued research keeps pushing nanoelectronics closer to revolutionary breakthroughs.
Energy Storage Systems
The incorporation of carbon nanotubes into energy storage systems has garnered considerable attention thanks to their remarkable electrical characteristics. Their high surface area and unique morphology present several benefits in both batteries and supercapacitors.
Batteries
Within the battery sector, carbon nanotubes are being explored for their ability to improve the overall energy density and life cycle of rechargeable batteries. one key characteristic of CNTs is their excellent conductivity, which enhances the rate of charge and discharge without significant power loss. This quality lends to the production of batteries that not only charge faster but also deliver superior performance.
Additionally, using CNTs can result in lighter batteries, which is a significant advantage for portable electronic devices and electric vehicles, where every ounce matters. However, challenges such as the uniform distribution of nanotubes within the battery matrix remain a topic of ongoing research.
Advantages of CNT Batteries
- High Energy Density: Increased storage capacity.
- Quick Charge Times: Reduced waiting periods for energy replenishment.
Disadvantages of CNT Batteries
- Manufacturing Complexity: They can be difficult and expensive to produce at scale.
- Potential Stability Issues: Further research is needed to ensure long-term durability.
Supercapacitors
Conversely, supercapacitors benefit from carbon nanotube properties by offering rapid charge and discharge cycles— an essential trait for applications requiring sudden bursts of energy. The incorporation of CNTs in supercapacitors allows these devices to store significant amounts of energy while ensuring a longer lifespan compared to conventional capacitors.
A notable feature of CNT supercapacitors is their high power density, enabling devices to deliver energy more quickly. This makes them particularly useful in applications ranging from regenerative braking systems in electric vehicles to powering electronic devices that require quick energy boosts.
Advantages of CNT Supercapacitors
- Fast Energy Delivery: Ideal for applications needing immediate power.
- Longevity: Extended lifecycle compared to traditional batteries.
Disadvantages of CNT Supercapacitors
- Lower Energy Density: While they charge quickly, the total energy stored is less than in batteries.
- Cost Implications: Production and material costs can be a limiting factor.
Composite Materials
Carbon nanotubes also play a vital role in the development of advanced composite materials. Their unique properties allow for significant enhancements in strength-to-weight ratio, electrical conductivity, and thermal stability when integrated into various matrices.
Using CNTs in composites not only augments the strength of the material—making it ideal for applications in aviation and automotive sectors—but also maintains a lightweight profile, which is crucial for performance. These composites can be tailored for specific uses, such as in the construction of durable and lightweight sporting goods or electronic enclosures that can dissipate heat more effectively.
As research continues, the full potential of CNT composite materials is expected to unlock new avenues across a spectrum of industries, including healthcare, automotive, and aerospace, driving the evolution of more sophisticated and reliable products.
Environmental and Health Considerations
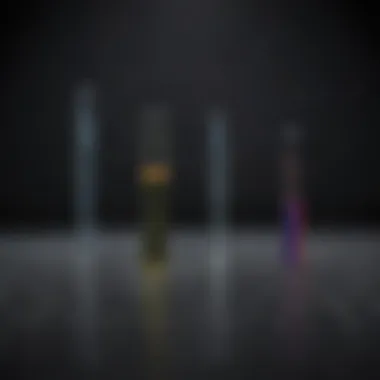
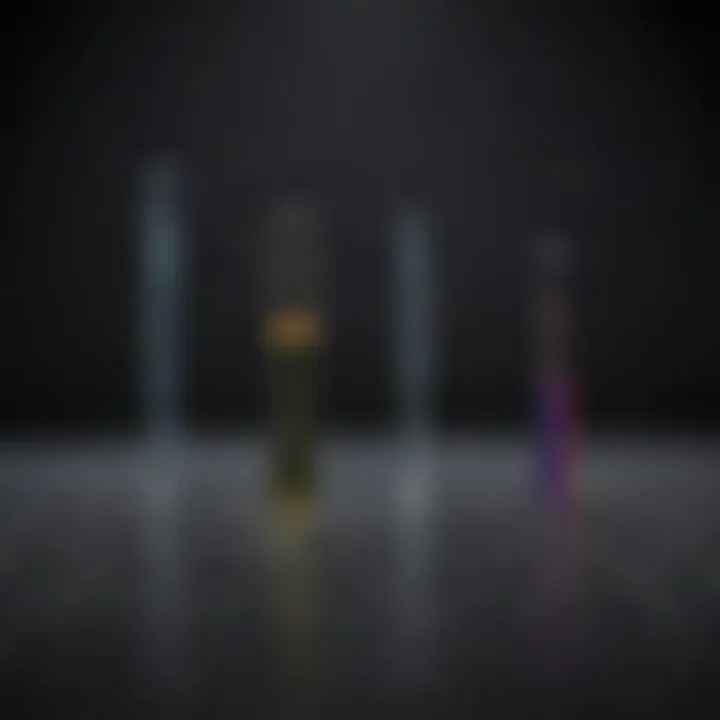
The exploration of carbon nanotubes (CNTs) is not solely a pursuit of their impressive mechanical and electrical properties. There lies an essential narrative regarding the implications of these unique structures on health and the environment. As CNTs find their way into a multitude of applications—from electronics to medicine—understanding their potential impacts is pivotal. Ignoring these facets could undermine the benefits achieved through technological advancement.
Toxicity and Biocompatibility
When it comes to toxicological assessments, the behavior of carbon nanotubes in biological systems should raise flags. Researchers have discovered that CNTs can display varying levels of toxicity depending on their structure, size, and functionalization.
- Size Matters: Smaller CNTs tend to penetrate biological barriers more effectively, which could lead to unforeseen health risks. Where the body’s immune system sees these nanotubes as invaders, adverse reactions may ensue.
- Functionalization Effects: The performance and behavior of CNTs alter significantly when functional groups are attached. Certain modifications may render them safer, while others can exacerbate toxicity, potentially leading to cell death or inflammation.
- In Vivo Studies: Investigations on animals have shown that CNTs can accumulate in organs like the liver, lungs, and spleen. These studies bring to light a pressing question: How do these accumulations translate to human health?
In regards to biocompatibility, it is crucial to consider how CNTs interact with cellular structures. Some paths show promise for targeted drug delivery, whereas others highlight severe inflammatory responses. Making strides in understanding these interactions will help shape the future application of CNTs in medical devices and therapies.
"Safety is the cornerstone of innovation with carbon nanotubes; as we forge the path ahead, we must tread carefully to ensure these materials do no harm."
Sustainability Issues
On a broader scale, sustainability challenges prevail in the context of CNT production and disposal. While the promise of harnessing carbon nanotubes is undeniable, several concerns have emerged that need addressing to align with global sustainability goals.
- Production Footprint: The manufacturing processes, particularly chemical vapor deposition (CVD) and laser ablation, can lead to substantial carbon emissions and chemical waste. Industry pressures must consider ways to optimize these methods, perhaps by transitioning to greener alternatives like bio-derived solutions.
- Lifecycle Analysis: Understanding the full lifecycle of carbon nanotubes—from extraction to end-of-life—is crucial. If these materials end up in landfills, they may contribute to environmental pollution. Planning for recycling or safe disposal methods is essential in decreasing their ecological footprint.
- Research on End-of-Life Management: Current innovations propose the re-utilization of CNTs in various sectors, from composite manufacturing to energy solutions. By closing the loop on their lifecycle, sustainability can be enhanced, reducing reliance on raw materials and minimizing waste.
In summary, as carbon nanotubes proliferate into different spheres of technology, ensuring their environmental and health impacts are comprehensively evaluated is a non-negotiable priority. The journey toward safe and sustainable applications of CNTs is complex but critical for our advancement, bridging innovation with responsibility.
Current Research Trends
Research in carbon nanotubes (CNTs) is rushing ahead like a freight train, with scientists uncovering innovative applications and synthesis techniques that could revolutionize various fields. Investigating the trends in this area not only informs us about the present capabilities of CNTs but also sheds light on where this fascinating material might lead us in the future.
Novel Applications in Medicine
The potential for carbon nanotubes in medicine is nothing short of staggering. Thanks to their unique properties, such as high surface area and electrical conductivity, researchers are exploring a multitude of medical applications that promise to change the way diseases are diagnosed and treated.
One particularly exciting avenue is drug delivery. CNTs can be engineered to carry therapeutic agents directly to target cells, minimizing side effects and maximizing effectiveness. For instance, anti-cancer drugs can be loaded into CNTs to facilitate their delivery, ensuring they reach malignancies with pinpoint precision.
Moreover, the conductive properties of CNTs make them suitable candidates for the development of advanced biosensors. These devices can detect biochemical substances in very low concentrations, paving the way for earlier diagnosis of conditions such as diabetes and infections. Imagine a tiny sensor embedded in a wearable device, providing real-time feedback on a patient’s health metrics.
- Benefits of CNTs in Medicine:
- Targeted drug delivery
- Highly sensitive biosensors
Despite all this promise, challenges remain. Toxicity and biocompatibility are significant concerns that need thorough investigation to ensure that these applications are safe for human use. The ongoing studies delve into these areas, aiming to build solid safety profiles for CNT-related technologies.
Innovations in Synthesis Approaches
As the demand for harnessing CNT capabilities grows, so does the push for innovative synthesis methods. Typically, techniques such as chemical vapor deposition (CVD) and laser ablation have been well-established, but new methods are emerging that show enhanced efficiency and versatility.
One recent development is the use of catalytic chemical vapor deposition (CCVD), which allows for a more controlled environment in which CNTs can be synthesized. This approach can yield higher quality nanotubes with fewer defects— a critical factor influencing electrical properties.
Another promising area is the hybridization of synthesis techniques, where researchers combine methods to create CNTs with tailored features. For example, combining CVD with electrospinning is being investigated, potentially allowing for the production of align CNTs on a large scale.
In summary, the field of carbon nanotube research is vibrant and rapidly evolving. Staying abreast of research trends is crucial, not only for the advancement of the technology but also to navigate the ensuing challenges and ethical considerations.
"The journey of understanding carbon nanotubes is just beginning, and their applications could unlock doors to solutions we haven't yet imagined."
Ongoing research in both novel applications and innovative synthesis methods will keep shaping the trajectory of carbon nanotube technology, promising a future rich with potential innovations.
Future Directions in Carbon Nanotube Research
As we gaze into the horizon of carbon nanotube research, it becomes increasingly clear that this material is not merely a fleeting trend in materials science. Instead, it stands as a pillar upon which many modern technologies could be built. The exploration of future directions in carbon nanotube research highlights not only the innovation potential of these structures but also their versatility across various domains. This section delves into specific challenges and opportunities, as well as interdisciplinary approaches that can catalyze further advancements in this exciting field.
Challenges and Opportunities
Carbon nanotubes present a myriad of both challenges and exciting opportunities. While their inherent properties make them promising candidates for a wide range of applications, their practical implementation often hits a few bumps along the road.
- Scalability of Production: Producing carbon nanotubes at a large scale while maintaining their quality is a daunting task. Current methods like chemical vapor deposition can be costly and time-consuming. Optimizing these processes to mass-produce high-quality CNTs could unlock their vast potential in commercialization.
- Material Integration: The integration of carbon nanotubes into existing materials poses another challenge. It requires overcoming issues related to compatibility with other substances, especially when considering composite materials. Developing techniques to effectively mix CNTs with polymers or metals could enhance the final products' performance significantly.
- Cost-Effectiveness: The production costs associated with carbon nanotube technology can be high. Identifying more economical synthesis methods or finding innovative approaches to reuse waste material can enhance the feasibility of CNT applications in everyday technology.
Despite these hurdles, the prospects are bright. Innovations in the field of electronics, energy storage, and nanomedicine rely heavily on advancements in carbon nanotube applications. The following key opportunities could steer future research:
- Development of flexible electronics that utilize carbon nanotubes for enhanced conductivity.
- Creation of energy-efficient batteries, drawing on CNT attributes to improve charge capabilities.
- Medical advancements, where CNTs can be utilized for targeted drug delivery systems.
Interdisciplinary Approaches
As carbon nanotube research progresses, collaborations across various disciplines will become more crucial. By synergizing insights from chemistry, physics, engineering, and biology, researchers can push the boundaries of what CNTs can achieve. Here are a few examples of such interdisciplinary approaches:
- Materials Science and Engineering: Merging materials science with engineering will pave the way for novel applications in composite materials. Understanding structural integrity while utilizing CNTs can enhance products from aerospace components to everyday electronics.
- Biology and Medicine: In the healthcare realm, chemistry and biology can unite to explore the biocompatibility of carbon nanotubes. This can forge new paths in regenerative medicine, where CNTs help repair or regenerate tissues.
- Environmental Science: Addressing sustainability challenges through carbon capture technology can depend on understanding CNTs. Collaborative efforts can create methods to utilize carbon nanotubes in filtering pollutants or aiding in clean energy solutions.
Culmination
The exploration of carbon nanotube (CNT) conductivity reveals pivotal insights not only into their unique structural features but also into the broad spectrum of their applications. As we draw the curtains on this comprehensive analysis, it's evident that this field is as rich as it is complex. The key findings highlight a number of critical factors that influence conductivity, such as dimensional characteristics and the presence of defects, while also shedding light on the quantum mechanical principles at play. These intricacies lay the groundwork for the future utility of CNTs in various domains.
"Understanding how carbon nanotubes conduct electricity is fundamentally important as it contributes to advancements in technology and materials science."
In our summary of key findings, we noted several significant aspects such as the distinction between single-walled and multi-walled CNTs and their respective electrical properties. These traits serve as the backbone for understanding how CNTs can versatility function in high-tech applications. The implications for both science and industry are profound. With the transition towards more sustainable technologies, the ability to harness the electrical conductivity of CNTs could lead to major breakthroughs in energy storage systems and miniaturized electronics. Furthermore, the ongoing research and innovations in synthesis techniques showcase a proactive stride towards sustainable practices, embedding the essence of carbon nanotubes into the fabric of emerging technologies.
As the landscape of carbon nanotube applications continues to expand, one must bear in mind the environmental and health considerations that accompany their use. While the benefits are numerous, addressing challenges surrounding toxicity and sustainability becomes paramount for long-term viability. It is through this conscientious approach that the field will not only advance technologically but will also establish a responsible framework for deploying these extraordinary materials into everyday life.