Cell Number Determination for 8-Well Chamber Slides
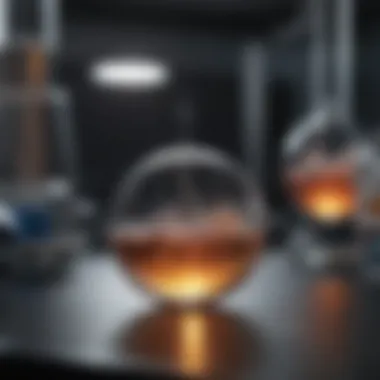
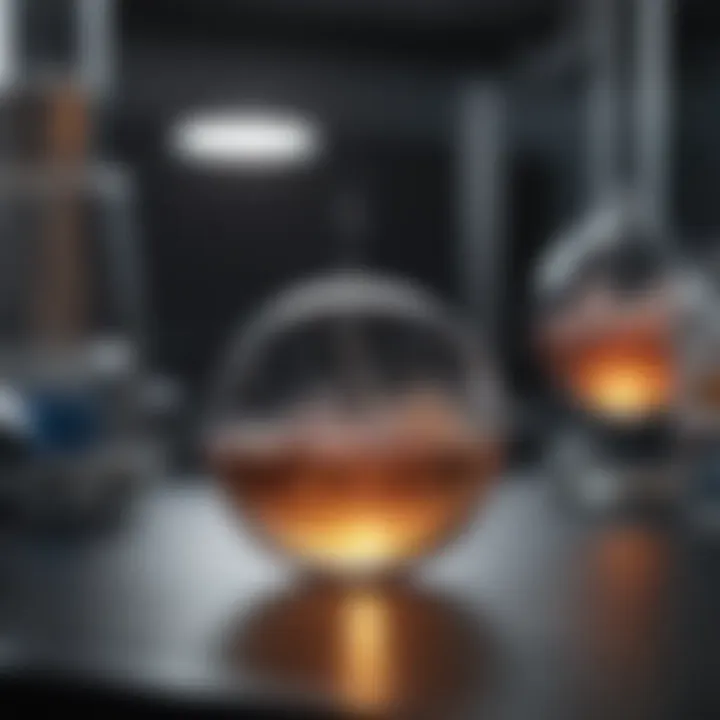
Intro
A key aspect of this discussion revolves around the cell plating density, a crucial parameter that can make or break an experiment. Too few cells might lead to inconclusive data, while an overabundance can induce competition for resources, skewing results. This balancing act requires a nuanced understanding of factors such as cell type, growth rate, and environmental conditions, ensuring that oneās experimental setup is both effective and reproducible.
As we proceed, letās not forget the variety of cell types that a researcher might encounter. Different cells exhibit unique behaviors and growth patterns, influencing how many you can plate. This variability can substantially impact the outcomes of your assays, leading to the need for tailored strategies based on specific objectives. For instance, adherent cells versus suspension cells require different considerations when determining cell numbers, fundamentally shaping growth dynamics.
In essence, this journey through the process of determining correct cell numbers culminates in a broader goal: achieving reliable and reproducible experimental results. With that in mind, letās turn our focus to the Research Methodology, where the underlying principles and technicalities begin to unfold, providing a roadmap for effective experimentation.
Prelims to 8-Well Chamber Slides
The 8-well chamber slide is a staple in numerous laboratory settings. Understanding its role is crucial, particularly when it comes to determining the right number of cells to plate in these wells. These chamber slides provide a controlled environment where various cellular experiments can be conducted efficiently. In essence, they act as miniature Petri dishes, allowing for localized study of cells, whether thatās for imaging, drug testing, or any number of high-throughput assays.
Why does this topic matter? Quite simply, itās about maximizing reliability and reproducibility in experiments. When cell density isnāt adequately calculated and adjusted, results can spiral into chaos, compounding errors that often lead to misleading conclusions. Thus, the 8-well chamber slide serves not just as a tool but as a bridge to valid scientific insight.
Purpose and Applications
The purpose of an 8-well chamber slide is multifaceted. Primarily it functions as a platform for culturing cells under conditions that can mimic physiological environments. This addresses various applications such as:
- Cell Viability Assessments: This is crucial for determining how cells respond to drugs or other treatments.
- Immunofluorescence Staining: Useful for visualizing cellular components or specific markers.
- Cell Proliferation Studies: For tracking how cell numbers change over time in response to particular stimuli.
- High-Throughput Screening: Offering standardized conditions across multiple samples for efficiency.
By utilizing this setup, researchers can gain valuable insights across diverse fields from cancer research to vaccine development.
Construction and Design Features
Diving deeper, the construction and design of 8-well chamber slides encapsulate functionality and practicality. Typically made from polystyrene or glass, the slides are designed with the following features:
- Individual Wells: Each chamber can be treated independently, facilitating multiple experiments simultaneously.
- Chamber Dimensions: Standard dimensions allow consistent cell plating across different experiments.
- Optically Clear: Enables precise imaging, whether through standard microscopy or fluorescence techniques.
- Sterilization Compatibility: Many slides can withstand high temperatures or other sterilization processes, ensuring minimal contamination.
Incorporating these features into experimental workflows can significantly enhance data quality, making the 8-well chamber slide not just effective but essential.
"Consistency is the mother of scientific enterprise; without it, findings may be more fiction than fact."
Equipped with this information, researchers can now appreciate how integral the 8-well chamber slide is in cellular biology, making informed decisions on how to handle cell densities and ensuring that their experimental designs yield consistent, actionable results.
Understanding Cell Density
Understanding cell density is pivotal for successful experiments utilizing 8-well chamber slides. This concept revolves around the concentration of cells in a given volume, directly influencing experimental outcomes. Whether one is conducting drug testing, growth assays, or any form of cellular manipulation, getting this density just right is the secret sauce for reproducible and reliable results.
Defining Cell Density
Cell density can be simplified as the quantity of cells present per unit volume, typically expressed in cells per milliliter (cells/mL). This metric is crucial in cell culture, as it dictates how many cells will migrate, proliferate, and eventually interact with one another in experiments. Scientists often utilize devices such as hemocytometers for accurate cell counting, allowing them to quantify their suspensions and adjust concentrations accordingly.
When calculating cell density, consider the following:
- Total Cell Count: Measure how many cells are in the suspension.
- Total Volume of Suspension: Take note of the exact volume in milliliters that your cells are in.
For instance, if your suspension contains 2 million cells in 20 mL, the density would be calculated as 100,000 cells/mL. This straightforward calculation can significantly impact your results if not addressed correctly.
Importance of Cell Density in Experiments
The significance of maintaining the right cell density cannot be understated. The density affects not only the growth conditions but also the interactions between cells, which can ultimately skew experimental results.
- Impact on Cell Behavior: Too high or too low cell density can lead to unforeseen behaviors. For example, overcrowding may trigger stress responses in cells, or too few cells might not generate enough data for biological relevance.
- Reproducibility: Consistent cell density across experiments is key to reproducibility. Minor variations in cell numbers can lead to significant differences in outcomes and interpretations.
- Optimization of Assays: Different assays require specific densities to function optimally. Understanding this allows for tailored applications, ensuring experiments yield the most pertinent results.
- Statistical Proficiency: Accurate cell density helps in achieving statistically significant results. With precise measurements, researchers can analyze data with more confidence.
"In cell culture, it's not just about having cells in a dish; it's about having the right number of cells at the right time and in the right conditions."
In summary, understanding cell density is foundational in both designing and executing experiments involving 8-well chamber slides. The nuances involved are not merely academic; they represent critical aspects of experimental design and interpretation that can lead to meaningful and impactful scientific findings.
Factors Influencing Cell Plating Numbers
In the realm of cell culture, especially when utilizing 8-well chamber slides, determining the correct number of cells to plate is of paramount importance. This decision affects not only the feasibility of the experiments but also their interpretability and reproducibility. Understanding the factors influencing cell plating numbers helps researchers optimize conditions tailored to their specific experimental goals. When researchers ignore these elements, outcomes can be unpredictable, leading to wasted resources and potentially flawed conclusions.
Type of Cell Line
The type of cell line being used plays a significant role in determining the optimal cell number for plating. Different cell lines exhibit unique growth characteristics, metabolic rates, and sensitivities. For instance, adherent cell lines like HeLa may require more initial plating cells compared to suspension cell lines like Jurkat due to their anchorage-dependent growth nature.
- Adherence: Cell lines that prefer to adhere to surfaces often require a higher cell density at the beginning to ensure adequate attachment and expansion.
- Growth Phase: Cells in exponential growth phase should be used for plating, as these cells are generally more viable than those that are quiescent or senescent.
- Variability: Individual cell lines can exhibit variance in growth rates; researchers need to conduct preliminary trials to establish baseline cell number requirements for their specific strain and culture conditions.
This variance indicates why relying solely on general recommendations could lead to miscalculations in the required cell numbers.
Growth Conditions
Growth conditions the cells are subjected to substantially affect how many to plate. Factors such as temperature, media composition, and exposure to gases like CO2 can alter both proliferation rates and cell viability.
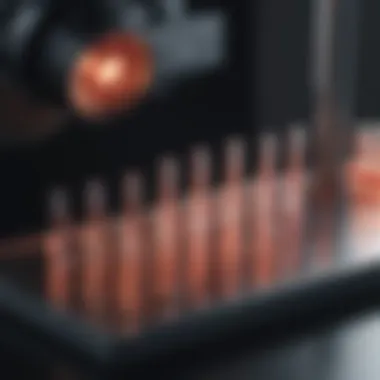
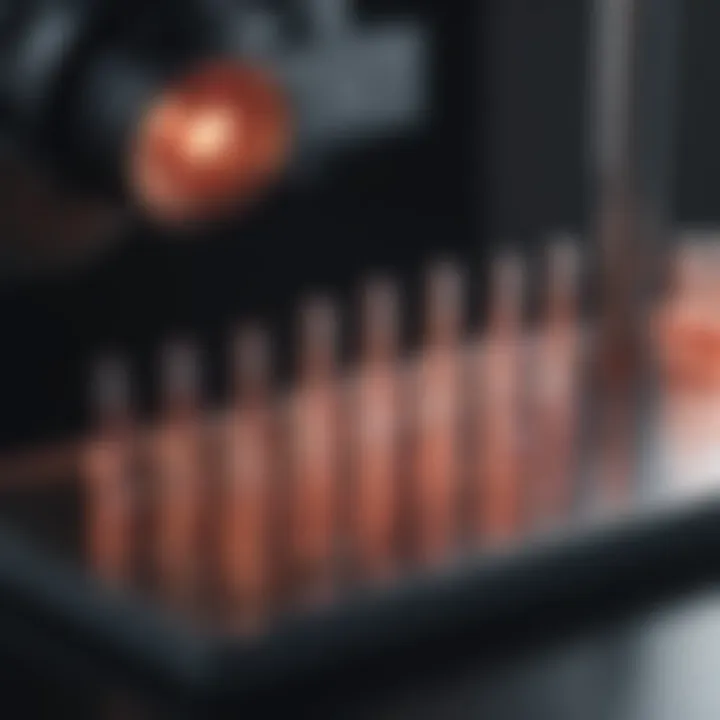
- Media Type: Different media compositions, like DMEM, RPMI, or F-10, contain varying nutrients, growth factors, and hormones, influencing how many cells can survive and proliferate.
- Temperature and pH: Cells derived from mammalian sources typically thrive at physiological temperatures (around 37°C) and neutral pH, which optimally supports their growth. Deviations can hinder or even impede cell growth.
- Incubation Time: The time cells are allowed to acclimate before the actual experiment can also affect assuming a steady state, maintaining consistent cell numbers becomes crucial in this setup.
By aligning growth conditions with the specific needs of the cell line, researchers can better gauge the appropriate cell density.
Assay Requirements
The requirements of the specific assay also dictate the number of cells to plate. Each experimental design needs a tailored approachābe it toxicity testing, proliferation assays, or differentiation studies. Considerations include:
- Sensitivity of the Assay: Some assays require denser populations to generate detectable signals, especially when measuring fluorescence or luminescence. For example, in cytotoxicity assays, a higher cell count can ensure accuracy in results.
- Statistical Significance: Placing an insufficient number of cells can result in variability, compromising the dataās validity. Usually, to achieve statistically significant data, researchers set a threshold number of replicates based on the variability known for the particular assay.
- End Point Measurements: Depending on whether the study requires real-time analysis or endpoint measurement, the plated cell density will alter based on the kinetics observed; assays that capture dynamic responses may need different initial cell densities.
Ultimately, the chosen assay must be critically assessed for its demands on cell density, and adjustments must be incorporated to guarantee reliable outcomes.
"Choosing the right cell number isnāt a one-size-fits-all scenario; itās a dance of conditions and requirements that can change with each experiment."
Being mindful of these influencing factors can guide researchersā decisions on cell plating numbers, ensuring experiments yield meaningful data and successful results.
Establishing Baselines for Cell Numbers
Determining the right number of cells to plate is more than a routine task; it is a foundational aspect of achieving reliable and reproducible results in any experimental setup. Establishing baselines for cell numbers not only informs researchers about the expected behavior of their cells but also ensures that they can minimize variability in their assays. This attention to detail becomes critical when facing various external factors that could influence cell performance.
When we talk about cell baselines, we are essentially speaking of creating a reference point for future experiments. Accurate baselines can help in several ways:
- Improved Experimentation: With a well-defined baseline, variations in data can be attributed more confidently to experimental changes rather than discrepancies in cell preparations.
- Enhanced Reproducibility: Establishing consistent cell densities allows other researchers to replicate results more effectively, advancing collective knowledge in the field.
- Resource Optimization: Knowing the optimal number of cells reduces the wastage of valuable reagents and samples, making experiments less costly and more efficient.
However, itās not just about pulling numbers out of thin air. Researchers need to consider multiple facets while establishing these baselines. The type of cell line, growth conditions, and specific assay requirements can all play pivotal roles in what those baselines should be. This chapter will delve into various strategies researchers can employ when trying to nail down these critical numbers.
Literature Review and Data Gathering
A literature review is often the first step to understanding the context of any experimental question. Here, we discuss the significance of exploring existing studies to find baseline cell numbers.
Gathering data from previous research allows you to:
- Identify Trends: Reviewing literature can highlight trends in cell density usage across similar experiments, offering valuable insights into what has worked well in the past.
- Categorize Cell Types: Various studies often focus on specific cell lines, and knowing which had successful outcomes can help tailor your baseline for your own research.
- Adapt Best Practices: Many protocols undergo peer review, which means that established studies often provide trustworthy recommendations for cell plating numbers.
Different sourcesāsuch as peer-reviewed journals, institutional protocols, and experimental textbooksāshould be consulted for robust data collection. Consider reaching out to online resources or forums, such as reddit.com, where many professionals share their experiences and insights. The nuances of these conversations might just give you the edge you need in deciding upon those critical cell numbers.
Consulting Protocols and Guidelines
Once a comprehensive literature review has been conducted, itās time to put it into action. Consulting standard protocols and guidelines can greatly enhance the reliability of your base numbers. These documents often stem from extensive peer-reviewed research and are composed by experts in the field.
- Standardized Protocols: Many institutions publish protocols that include cell plating densities relevant to specific cell types and experimental designs, such as those from wikipedia.org.
- Guidelines from Professional Organizations: Organizations such as the American Society for Microbiology often develop guidelines that address cell culture specifics, providing insights into best practices for cell culture and the relevant plating densities.
- Adaptability Consideration: While guidelines serve as solid starting points, adaptability for specific situations is crucial. Adjusting the recommended numbers based on your specific experimental conditions is something every experienced researcher should be comfortable doing.
By following these steps, researchers will be armed with the necessary information to establish effective baselines, paving the way for a streamlined protocol that can vastly enhance the success rates of their experiments.
Calculating Optimal Plating Density
When it comes to cell culture, getting the correct number of cells to plate in an 8-well chamber slide can feel a bit like threading a needle in a haystack. Itās a balancing act between maximizing experiment efficacy and ensuring the health of the cells. Calculating the optimal plating density stands at the core of achieving reproducible results in any cellular assay. The number of cells you begin with can influence everything from cellular interactions to the response measured in your experiments.
Why is this important? Optimal plating density helps in maintaining healthy cell growth, achieving the right confluence, and providing appropriate conditions for your cells to thrive. Too few cells can lead to skewed data, whereas too many might create overcrowded conditions, leading to stressed cells and non-ideal responses.
The result of inaccurate calculations can have ripple effects through your study, skewing data and wasting valuable research time. Understanding the intricacies around correct calculations allows you to pave a smoother road to success.
Mathematical Formulation
Calculating optimal plating density doesn't have to be rocket science, but it does require a bit of math. The general formula to determine the plating density can be expressed as:
Hereās how to break it down:
- Total Number of Cells: This is the number of cells you plan to start with based on your experimentation needs. Always remember to consider cell viability.
- Total Surface Area: For an 8-well chamber slide, the surface area for each well typically measures around 1 cm². Multiply this by 8 to derive the total area for all wells, which stands at 8 cm².
For instance, if you decide to plate 500,000 cells across 8 wells, you'd calculate it as follows:
[ PD = \frac500,0008 = 62,500 \text cells/cm^2 ]\
This calculation sets up a solid baseline before any cell viability adjustments occur. Sounds simple, right? But there's more to the picture when accounting for cell viability.
Adjusting for Cell Viability
Having a solid cell count is one thing, but making sure those cells can actually survive and thrive is another. Adjusting for cell viability gives you a better picture of how many cells you should initially plan to plate. This is crucial for ensuring that the effective density corresponds to healthy cell adherence and growth.
Key Factors to Consider:
- Viability Percentage: If your cell suspension tests show that only 85% of your cells are viable, this is a crucial number. Multiply your desired counts by the viability percentage to ensure a good starting point.
- Time Sensitivity: Cells have a tendency to drift towards lower viability if theyāve been left hanging too long post-harvest. Quick processing will get your cells in optimal shape.
- Cell Line Characteristics: Different cell lines have unique growth rates and adherence properties. Some might flourish in dense cultures, while others prefer a more spread-out arrangement.
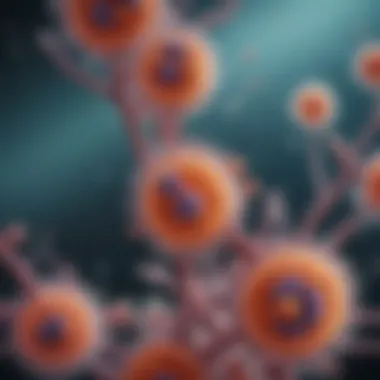
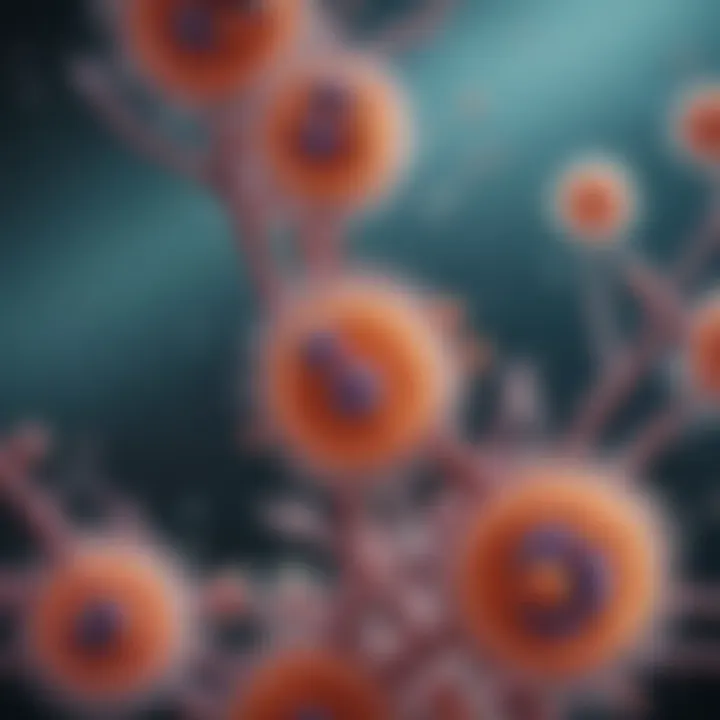
For instance, recalculating using the 85% viability observed above:
[ \textAdjusted Cells = \frac500,0000.85 \approx 588,235 \text cells ]\
Youād need about 588,235 cells before plating to ensure optimal growth within your 8-well plate. Integrative planning of these calculations can ensure a smoother experimental journey and richer, more reliable metrics when you reach the end of your study.
Remember: Kicking off with the right numbers can significantly minimize downstream troubleshooting efforts and bolster the integrity of your scientific findings.
In summary, calculating optimal plating density while adjusting for cell viability is a meticulous but absolutely critical part of cell culture in an 8-well chamber slide. Itās the kind of foundational steps that, while they might seem minor on their own, add up to make a world of difference in experimental results.
Practical Considerations
Understanding practical considerations when plating cells in an 8-well chamber slide is critical for achieving consistent and reproducible results. These elements are not just ancillary thoughts but fundamental aspects that lay the groundwork for successful experiments. The nature of the cell suspension, methodologies for accurate cell counting, and adherence to protocols can drastically influence outcomes. Thus, careful attention to these details can mean the difference between a fruitful assay and one hindered by variability or unforeseen issues.
Preparation of Cell Suspension
The first step in this process is preparing the cell suspension. It's not as simple as tossing a clump of cells into a flask and calling it a day. The preparation requires meticulous attention to detail. Ideally, the cell suspension should be homogeneous, ensuring that each well of the chamber receives a uniform distribution of cells. This uniformity is key in reducing variability in assay results.
When preparing your suspension, consider the following:
- Cell density: Always start by diluting your cells to the desired concentration to avoid overcrowding. This will help facilitate accurate counting and improve cell viability post-plating.
- Medium Choice: The growth medium used should be relevant to the cell line. It matters because cells react differently when nourished with various formulations. A medium well-suited for one cell line could very well deny the appropriate growth factors for another.
- Pipetting Techniques: Gentle pipetting is non-negotiable to prevent shear stress that could lead to cell death. Aiming for a gentle mix is crucial to keep the suspension even throughout.
Techniques for Accurate Cell Counting
For accurate cell counting, you want to rely on techniques that are both reliable and reproducible. A myriad of methods exists, but here are a few practical techniques to consider:
- Hemocytometer Use: This age-old tool allows you to count cells directly under a microscope. With a little practice, you can achieve high accuracy, but it does have a learning curve.
- Automated Cell Counters: If youāre part of a lab that has the luxury of modern devices, automated counters provide efficiency and precision. Besides, they reduce human error and can process samples rapidly.
- Trypan Blue Exclusion Test: This method helps to distinguish between live and dead cells. Keeping track of viability ensures that only healthy cells are counted for plating.
Plating in the 8-Well Chamber Slide
Plating cells in an 8-well chamber slide serves as a foundational step in cell culture experiments, pivotal for both quantitative and qualitative analyses. The well-controlled environment offered by these slides makes them an ideal choice for researchers requiring precision. Understanding the correct way to plate cells not only enhances assay reliability but also affects the overall consistency of experimental results. Well-plated cells can lead to more reproducible experiments, making it easier to draw valid conclusions from the data generated.
In the realm of cellular biology, numerous applications can be expedited thanks to proper plating techniques. Applications include drug screening, cytotoxicity testing, and immunocytochemistry, among others. Each application demands a specific density and uniformity in plating to achieve reliable outcomes. Consequently, this underscores the need for thoughtful consideration of factors influencing the general plate procedure, such as the specific type of cell line in use and the intended measurement outcomes.
Step-by-Step Plating Protocol
- Preparation of Materials: Gather your 8-well chamber slide, culture medium, and the cells you plan to plate. Make sure everything is sterile to avoid contamination.
- Cell Counting: Use a hemocytometer or automated cell counter to establish your desired cell density. Knowing how many cells to plate is critical, as it significantly influences how the cells will behave and interact.
- Dilution of Cell Suspension: If needed, dilute the cell suspension to achieve the optimal concentration per well. It is crucial to mix the suspension thoroughly to ensure an even distribution before plating.
- Plating Cells: Gently pipette the cell suspension into the wells of the chamber slide. Avoid introducing bubbles as they can disturb cell adherence. It is advisable to add the medium slowly down the wall of the well instead of directly into the center to maintain cell health.
- Incubation: After plating, incubate the chamber slide at suitable conditions (typically 37°C with 5% COā) for the necessary duration before proceeding with observations or assays.
- Medium Change (if necessary): After a period of incubation, assess cell attachment and viability. Changing the medium might be necessary to refresh nutrients and remove any non-attached cells.
Tips for Consistent Technique
To achieve a uniform cell distribution, there are several practices that can help maintain consistency:
- Pipetting Technique: Always use consistent pipetting techniques. Change tips between wells to prevent carryover and ensure there are no excess cells from the previous well.
- Use of a Controlled Environment: Maintain a stable environmental condition such as temperature and humidity within the incubator to minimize stress on the plated cells.
- Cell Handling: Minimize the time cells spend out of their culture conditions during the plating process.
It's important to remember that establishing a successful plating routine can save significant time and resources in future experiments. Consistency leads to reliable results, paving the path for further advancements in research.
Monitoring Cell Growth
Monitoring cell growth is not just a technical step in the process; it's a vital element that can determine the success or failure of an experiment. Without proper gauge of cell growth, the aim of achieving desired outcomes in assays hangs on a delicate balance, susceptible to variables beyond control. Keeping a close eye on cell growth enables researchers to optimize conditions, refine techniques, and anticipate possible pitfalls down the line.
Aside from just counting cells, monitoring cell growth encompasses observing various factors including physiological changes, response to treatment, and overall health of cell cultures. These factors are crucial as they can significantly impact experimental results, often dictating the timing for further procedures like drug exposure or analysis.
Through effective monitoring, one can glean insights on:
- Cell proliferation rates - understanding how quickly cells multiply can provide clues about their health and metabolic state.
- Response to interventions - whether thatās a new drug or a shift in environmental conditions, knowing how cells react can inform the next steps in experimentation.
- Detection of abnormalities - early detection of unusual growth patterns may prevent wasting resources on flawed experiments.
In sum, not just a box to tick off, monitoring cell growth asks for diligence and thoughtfulness. Implementing this practice will place researchers on a firmer footing towards achieving reliable and reproducible results.
Tools for Monitoring
Equipping oneself with the right tools is half the battle won when it comes to monitoring cell growth. A variety of methodologies exist, each providing unique advantages depending on the experimental design and specific goals at hand. These tools range from high-tech instruments to fairly straightforward techniques:
- Cell Counters - Automated cell counters leverage technology to provide quick and accurate cell counts, saving researchers valuable time.
- Hemocytometers - Though more traditional, these are still widely used for manual counting and can be invaluable when combined with staining methods to assess viability.
- Microscopy - Optical or fluorescent microscopy allows researchers to visualize cell morphology and count, analyze their health, and even detect contamination.
- Real-Time Monitoring Systems - Some advanced systems enable continuous monitoring of cell behavior, providing real-time data on various parameters without disturbing the culture.
- Optical Density Measurements - Utilizing a spectrophotometer to measure light absorbance can gauge cell growth indirectly, particularly useful for bacteria and yeast.
Each of these tools has its strengths and weaknesses, thus the choice often boils down to the specifics of the study being conducted as well as budget considerations.
Assessing Confluence Levels
Assessing confluence levels is an essential aspect of monitoring cell cultures. Confluence refers to the percentage of the culture surface covered by cells, providing a visual cue of their growth and readiness for subsequent experiments. This parameter is not just a number; it holds critical significance in cell biology experiments, offering a snapshot of the cellular environment at any point in time.
Maintaining the appropriate confluence level is crucial for several reasons:
- Cell Behavior: Cells behave differently at various confluence levels. For instance, they may alter their gene expression profiles, a factor that can heavily influence experimental results.
- Subculturing: Knowing the confluence allows researchers to determine the right time for subculturing. Waiting too long can lead to stress or differentiation, impacting the consistency of the cell line.
- Assay Requirements: Many assays have specific confluence requirements to ensure reliability, and failing to meet these can yield misleading results.
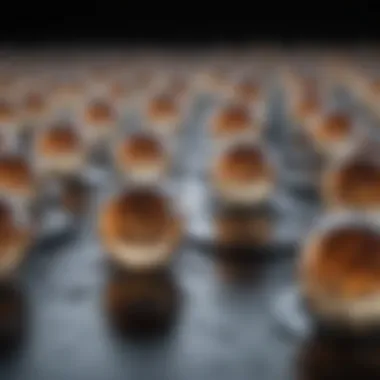
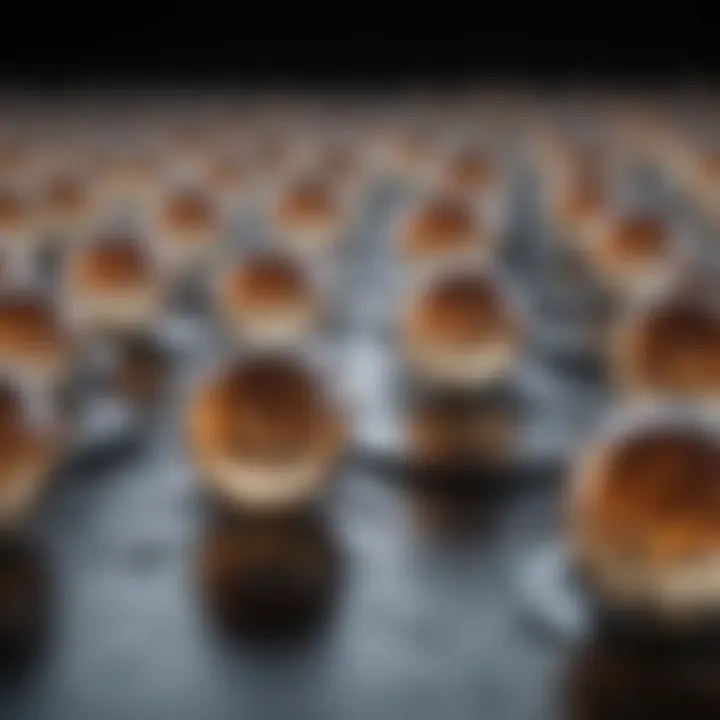
To accurately assess confluence levels, researchers often employ:
- Visual Inspection: Simply looking at the cells under a microscope can give a good estimation.
- Image Analysis Software: Tools can quantitatively analyze images, generating precise values for confluence and reducing human error.
- Cell Counting: Utilizing cell counters or hemocytometers in conjunction with observed confluence can give a well-rounded metric.
In summary, effectively assessing confluence levels is an indispensable step in maintaining optimal conditions for cell growth and experimental integrity. By combining visual assessment with analytical tools, researchers can ensure their cell cultures remain robust and productive.
Troubleshooting Common Issues
In any experimental setup, especially in cellular experiments, encountering issues is not uncommon. For researchers working with the 8-well chamber slide, understanding how to troubleshoot common issues will offer a smoother path to reliable results. Addressing challenges promptly can save time and resources, ensuring the integrity of the experiment. Moreover, being equipped to tackle problems such as contamination or unexpected cell death is invaluable. This section breaks down these issues into manageable components with practical guidance for overcoming them.
Identifying Contamination
Contamination can derail even the most carefully designed experiments. It often manifests as unexpected changes in cell morphology, unusual growth patterns, or colors in the culture as the substrate starts to show unwanted turbidity. It is vital to recognize contamination early to mitigate its effects and prevent faulty data from skewing results.
Some common sources of contamination include:
- Airborne bacteria or fungi
- Contaminated reagents
- Inadequate sterilization of equipment
- Use of culture media that are past the expiration date
To identify contamination, here are some practical steps:
- Visual Inspection: Look for any morphological changes in the cells. Healthy cells should appear uniform and exhibit normal growth patterns.
- Culture Medium Change: If media appears cloudy, it might indicate microbial growth. Replace media as a precaution and observe changes.
- Using Indicators: Implementing specific stains can help detect certain bacteria or fungi, differentiating between live and dead cells.
Mitigating the risk of contamination often requires the implementation of strict protocols. Regular cleaning of the workspace, using sterile techniques, and validating all reagents are essential for maintaining a contamination-free environment. Always remember, a little vigilance can go a long way in preserving the quality of your work.
Addressing Cell Death
Cell death can occur for several reasons, whether from improper handling, unsuitable growth conditions, or even the parameters of the experiment itself. Understanding how to address cell death will help in refining experimental design and achieving replicable results.
Signs of cell death include:
- Loss of cell membrane integrity
- Detachment from the substrate
- Visible necrosis or lysis in culture
Here are some strategies to minimize and address cell death:
- Monitor Growth Conditions: Ensure temperature, pH, and humidity are optimized for the specific cell line being used. For example, some cells might prefer a different concentration of CO2 in an incubator.
- Evaluate Cell Handling Techniques: Avoid harsh handling during plating. Use gentle pipetting methods to minimize shear stress.
- Regular Viability Testing: Employ trypan blue exclusion or flow cytometry to assess cell viability regularly during experiments. This can help identify issues before they become significant.
Resolving issues related to cell death can often lead to improved cell lines and better overall experimental outcomes. Always keep detailed records of conditions and changes in cell behavior for future reference.
"Prevention is better than cure." This adage rings particularly true in cell culture work, where proactive measures can avert contamination and cell mortality.
Case Studies and Examples
In the realm of cell culture, practical examples are invaluable. Delving into case studies can shed light on best practices and help avoid common pitfalls. They serve as a bridge connecting theory with real-world application, offering insights that might not be apparent from standard textbooks. Examining successful experiments showcases effective plating strategies while failures provide lessons that can inform future endeavors.
Successful Plating Strategies
A noteworthy case study involved a team focusing on human epithelial cells. They aimed to understand the effects of a specific drug on cell growth. Initially, they calculated the plating density by following a general guideline suggesting around 50,000 cells per well for the 8-well chamber. However, upon reviewing previous literature, they realized the need to adjust for confluence levels and specific confluence targets to be achieved for relevant experimental outcomes.
As a result, they slightly increased the plating density to 65,000 cells per well. Not only did this adjustment lead to more consistent and replicable results, but it also uncovered new insights into the drugās mode of action. Their successful plating from the outset ensured that they had robust data to analyze, highlighting how critical it is to adapt plating densities to the cellular context being investigated.
This experience reinforces a key element in cell cultureāhaving a tailored approach rather than a one-size-fits-all can lead to better outcomes. Researchers should always consider cellular behaviors, growth rates, and division times specific to their chosen cell line before finalizing the plating number.
Lessons Learned from Failed Experiments
Contrastingly, consider a research group that took on colorectal cancer cells for their experimentation. They were determined to follow a specifically detailed protocol found in a high-impact journal but neglected to adjust the settings for their lab-specific conditions. They had inadvertently adopted a cell density cited as optimal for broader cancer cell lines but failed to recognize that their particular cell type lacked the same growth characteristics.
After multiple tries, the team evaluated their approaches only to find that the initial plating density they adopted was too high for their colorectal cancer cells. As expected, they faced issues with cell death and nutrient depletion, ultimately derailing their experiments. The lesson? Adhering to prescribed methods without consideration for biological nuances can lead to significant challenges.
Itās crucial to integrate personal experience and review past experiments within a particular laboratory to tweak protocols to suit individual needs. Experimentation often demonstrates that even established methodologies need fine-tuning when applied to specific conditions. To avoid such setbacks, researchers should routinely document their findings. Establishing a detailed log can help in deriving insights that might lead to revisions in strategies used initially.
In sum, case studies amalgamate insights on both the triumphs and tribulations in cell culture, providing a compelling narrative that can be extremely beneficial for navigating experimental designs.
Whether from successes or mistakes, the experiences captured in these examples can provide a much clearer pathway toward successful plating and experimental fidelity.
End
The conclusion is the crowning moment of any scholarly piece, acting as the final word on the complexities of determining appropriate cell numbers for plating in an 8-well chamber slide. It's not just a mere recapitulation; rather, it serves to cement the significance of the myriad factors discussed throughout the article. The intricate interplay of cell density, type of cells, and the specific goals of an experiment culminate here, offering a pathway for researchers to navigate their experimental designs with confidence.
Through this discussion, readers can grasp how variations in techniques and conditions can significantly influence cell behavior and assay results. Understanding these fundamentals ensures that experiments not only achieve their aims but also contribute valuable data for future research endeavors. The importance of accurate cell counting and optimal plating cannot be overstated, as these factors lay the groundwork for reliable and reproducible results in cellular and molecular biology.
Summary of Key Points
- Cell Density Matters: Finding the right cell density is crucial for experiment success. Too few cells might lead to inconclusive results, while too many could skew data.
- Cell Types Influence Outcomes: The type of cells being plated affects their growth patterns and interactions, making it essential to consider this in the planning stages.
- Experimental Design is Key: The objectives and design of the experiment dictate necessary cell numbers. A well-thought-out approach yields fruitful data.
- Consult Protocols: Following established protocols can streamline the preparatory phase, ensuring researchers stay aligned with best practices.
Future Directions in Cell Culture Techniques
As science pushes forward, the future of cell culture techniques appears promising and dynamic. Here are several potential avenues to explore:
- Automation of Cell Counting: Advances in technology may lead to fully automated systems for cell counting, increasing precision and efficiency in experimental setups.
- Personalized Cell Lines: As our understanding of genetic variations grows, the development of personalized cell lines may pave the way for tailored experiments that consider individual differences in response to treatments.
- Integration of AI: Artificial intelligence could revolutionize how we analyze cell growth patterns and behaviors, allowing for predictive modeling that improves experimental outcomes.
- Exploration of 3D Cultures: Moving beyond traditional 2D cultures to 3D systems may provide more accurate representations of tissue behavior, which could lead to breakthroughs in drug testing and disease modeling.
In summary, considering the basics of cell plating might seem simplistic, yet it profoundly impacts the trajectory of research. The knowledge gained from accurately determining cell numbers will continuously shape scientific inquiry and innovation.