Chemical Engineering: Principles, Applications, and Impact
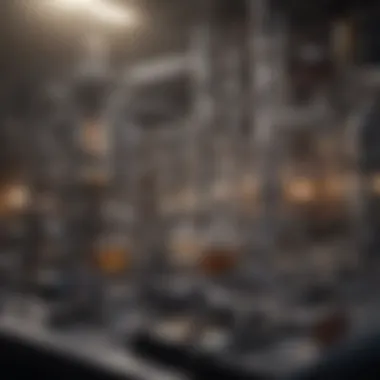
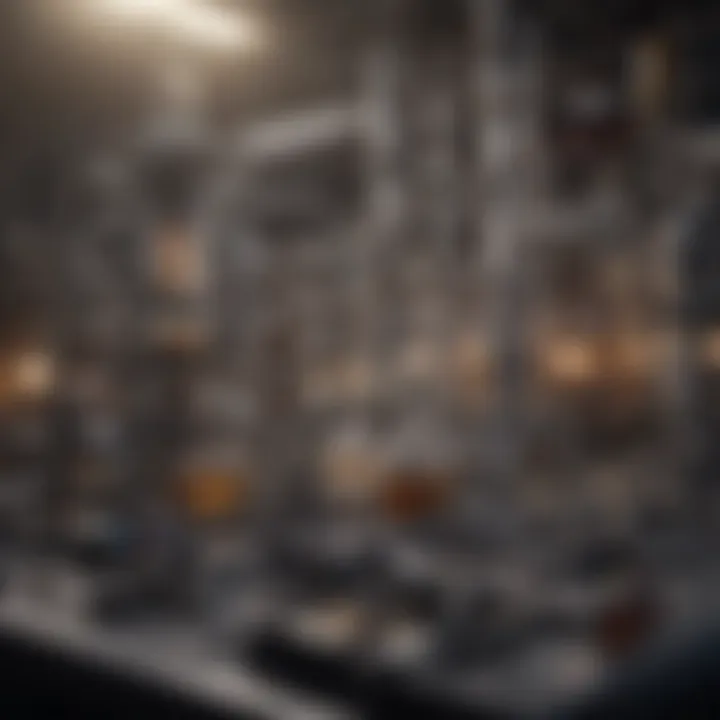
Intro
Chemical engineering is often seen as a crux where science and technology meet. It involves not merely the transformation of materials but also the design and optimization of processes that are integral to producing a vast array of products. From pharmaceuticals and food to fuels and plastics, the impact of chemical engineering reverberates through daily life.
To understand this field more thoroughly, we need to insert ourselves into its fabric and dissect its core principles. Such an understanding is crucial because chemical engineers play a pivotal role in addressing pressing issues like climate change, energy shortages, and waste management. Quite often, the situations they tackle require a multi-disciplinary approach, combining chemistry, biology, and physics. This article aims to act as a lens, bringing into focus the various aspects that shape the world of chemical engineering. We'll also take a peek into how educational pathways prepare aspiring engineers to hit the ground running in their careers, ensuring they are not only technically skillful but also ethically grounded.
"Brilliance is not solely in what you know; it's in how you use what you know to address real-world challenges."
As we journey through the intricate world of chemical engineering, a thoughtful exploration awaits that promises to unravel the complexities of this discipline.
Foreword to Chemical Engineering
Chemical engineering stands as a pivotal branch of engineering that intertwines chemistry, biology, physics, and mathematics to develop processes that transform raw materials into valuable products. Understanding this field is crucial, not just for aspiring students and professionals but also for policymakers and industry leaders who require insights into sustainable practices and technological advancements. Chemical engineers play an essential role in various industries including pharmaceuticals, energy, food processing, and materials science. Their work directly impacts our daily lives—from the medications we take to the gasoline we use in our cars.
Defining Chemical Engineering
Chemical engineering can be best described as the application of physical sciences along with life sciences together with mathematics to convert, produce, and use chemicals, materials, or energy. It involves creating efficient processes and systems for the large-scale manufacture of products, integrating diverse elements as varied as heat transfer and reaction kinetics. This discipline demands a strong foundation in related sciences as well as the ability to think analytically and innovatively.
Some of the key areas that chemical engineers focus on include:
- Process Design: Formulating the processes needed to produce materials efficiently and safely.
- Plant Operation: Ensuring production processes run smoothly and addressing any issues that arise.
- Research and Development: Innovating new ingredients and improving existing processes to enhance efficiency.
In essence, chemical engineering serves as a bridge between science and practical application, emphasizing the importance of turning theoretical knowledge into real-world solutions.
Historical Context
The roots of chemical engineering can be traced back to the ancient practices of metallurgy and alchemy; however, as society evolved, so too did the need for systematic approaches to chemical processes. During the Industrial Revolution, significant breakthroughs occurred, setting the stage for formalized chemical engineering. The establishment of the first chemical engineering degree programs in the early 20th century marked a significant milestone in merging theoretical foundations with practical applications.
In the mid-20th century, the profession began to gain recognition as a distinct engineering discipline. As global challenges grew, particularly with rapid industrialization, chemical engineers became crucial in addressing environmental concerns and societal needs. The shift from purely chemical manufacturing to ensuring safety and sustainable practices reflects the profession's evolution over the years. Nowadays, chemical engineers not only work to make production more efficient but also focus on minimizing environmental impacts, embodying the principles of sustainability and responsibility.
"Chemical engineering is about understanding the science and integrating it with practical application to create solutions for real-world problems."
In summary, a solid grasp of chemical engineering principles allows professionals to tackle some of the most pressing challenges today, from creating more efficient energy sources to developing biocompatible materials. This overview serves as a vital entry point into a complex field that continues to shape the world we live in.
Fundamental Principles
The realm of chemical engineering thrives on a foundation encompassing several fundamental principles. These principles not only serve as the bedrock of the discipline but also guide chemical engineers in the design and development of processes, materials, and systems. Understanding these core concepts is vital—just like a sturdy building needs a solid foundation, a chemical engineering project relies on these principles to ensure efficiency and effectiveness. By grasping the interconnections among mass and energy balances, thermodynamics, and transport phenomena, professionals can make informed decisions that propel innovation and address real-world challenges. Therefore, fundamental principles are not just academic theories; they are essential tools that empower engineers to transform ideas into tangible results, enhancing productivity and sustainability in our industries.
Mass and Energy Balances
At the heart of chemical engineering lies the principle of mass and energy balances. This concept emphasizes that matter and energy are neither created nor destroyed in a closed system. Consider a simple example: when making a chocolate cake, each ingredient has to be measured precisely. If you add too much flour, the cake won't rise properly; if you forget the eggs, it will lack structure. Similarly, in chemical processes, one must account for all inputs and outputs.
The applications of mass and energy balances are numerous:
- Process Design: Engineers utilize these balances to design reactors, distillation columns, and other equipment efficiently, ensuring all necessary materials are present.
- Environmental Impact: By monitoring material usage, engineers can minimize waste and optimize resource consumption, supporting sustainability initiatives.
- Quality Control: Keeping track of balance ensures that processes remain consistent, producing end products of reliable quality.
Maintaining a delicate equilibrium helps engineers troubleshoot issues in operational performance, leading to better productivity and lower costs.
Thermodynamics in Chemical Engineering
Thermodynamics plays a pivotal role in chemical engineering, laying the groundwork for understanding how energy is transferred within systems. The laws of thermodynamics dictate how energy transformations affect matter's physical and chemical properties.
Imagine trying to boil water over a fire. The heat energy flows from the flame to the pot, raising the water temperature until it reaches its boiling point. In chemical processes, similar fundamental principles apply, influencing phase changes, reaction kinetics, and energy efficiency. The critical aspects of thermodynamics include:
- Energy Conservation: Understanding how energy can be transformed to optimize processes.
- Equilibrium: Knowing when reactions reach a stable point helps in designing effective chemical reactions.
- Efficiency Calculation: It provides insights into optimizing the performance of equipment, reducing energy consumption, and minimizing environmental impacts, ultimately leading to cost-effective solutions.
As such, thermodynamics serves as the compass in navigating the complex chemical landscapes that engineers work within.
Transport Phenomena
Lastly, transport phenomena—the study of how material, energy, and momentum move through different media—completes the triad of fundamental principles in chemical engineering. These phenomena help engineers model, analyze, and control the movement of substances in various scenarios.
Transport phenomena can be observed in daily life. Think about how quickly ice melts in warm water compared to cold water. The heat flows from water to ice, enhancing its melting rate. This principle applies on a grander scale in industries, with applications such as:
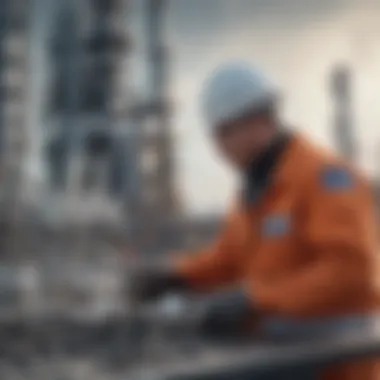
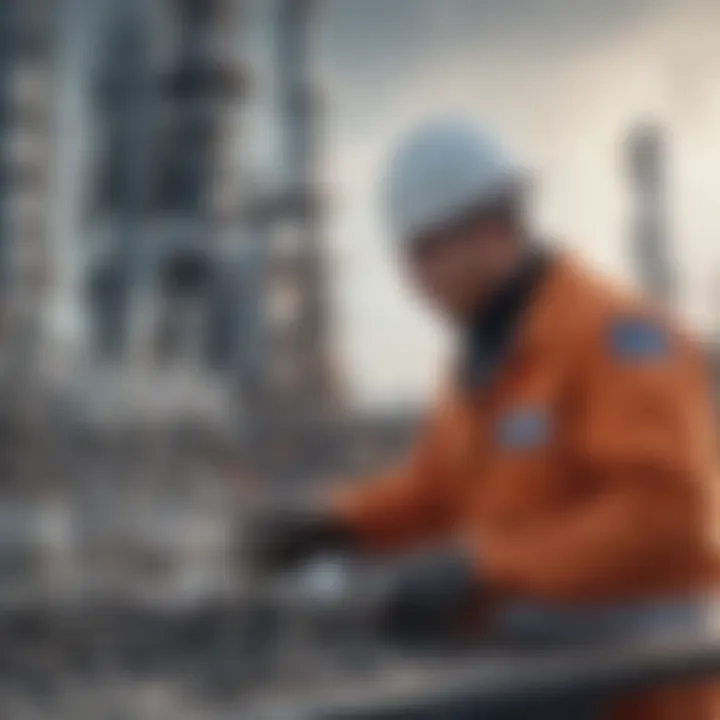
- Mass Transfer: Relevant in separation processes, such as extracting essential oils from plants.
- Heat Transfer: Crucial in designing heat exchangers, where efficiency translates directly to energy savings.
- Fluid Dynamics: Impacts every chemical process involving liquids and gases, affecting flow rates and mixing efficiency.
"Chemical engineering is not just about chemistry; it’s about transforming ideas into real solutions."
By mastering these fundamental principles, engineers can lead the charge toward innovations that meet modern-day challenges.
Key Areas of Application
When diving into chemical engineering, understanding the key areas of application is fundamental. This section unpacks the significance of these applications, which not only embody the nuts and bolts of chemical engineering but also reveal its expansive influence on various sectors. Chemical engineers wear many hats and operate across industries, enhancing processes and driving innovation. Their work plays a pivotal role in improving production efficiency, enhancing product quality, and ensuring environmental safety, thus addressing both industrial and societal challenges.
Process Engineering
Process engineering is arguably the backbone of chemical engineering applications. It focuses on designing, operating, and optimizing processes to transform raw materials into valuable products. The strength of this field lies in its systematic approach to creating processes that are not only cost-effective but also scalable. Understanding mass and energy balances plays a crucial role here. Engineers apply principles of thermodynamics to ensure that reactions are conducted under optimal conditions.
Some of the major components of process engineering include:
- Material Selection: Choosing the right type of materials for reactions is critical. The wrong material can lead to inefficient processes, wastage, or even catastrophic failures.
- Process Design: Engineers design processes with the intent of maximizing yield while minimizing energy and material consumption. They utilize advanced modeling techniques to simulate and predict process behavior.
- Quality Control: Maintaining product quality requires regular monitoring and adjustments in the process parameters. The concept of quality by design is essential here.
In summary, without robust process engineering, advancements in chemical manufacturing and other applications would stall. Occupying a central role in driving productivity, it also sets the stage for innovation.
Materials Science
Materials science in chemical engineering examines the relationship between the structure and properties of materials. This area is crucial in developing new materials that meet the specific demands of various applications. Engineers in this field study everything from traditional composites to modern nanomaterials.
Some notable aspects of materials science include:
- Polymer Engineering: This focuses on the synthesis and processing of polymers, leading to a diverse range of products from plastics to elastomers, each tailored for specific applications.
- Nanomaterials: With the rise of nanotechnology, the design and manipulation of materials at the nanoscale is leading to breakthroughs in electronics, medicine, and energy storage.
- Ceramics and Metals: Understanding how the molecular structure impacts the performance of these materials can lead to stronger, lighter, and more durable options for various industries.
Through the prism of materials science, engineers not only enhance existing materials but also pioneer novel solutions to complex challenges, contributing significantly to technological progress.
Biochemical Engineering
Biochemical engineering merges principles of biology with chemical engineering. This area focuses on the production of materials through biological processes, which often leads to more sustainable practices. It encompasses a wide range of applications, particularly in pharmaceuticals, agriculture, and energy, by utilizing microorganisms or enzymes to produce desired products.
Key elements in biochemical engineering include:
- Bioprocess Design: Developing processes that efficiently cultivate microorganisms or cells to produce bioproducts like enzymes, antibiotics, or biofuels.
- Metabolic Engineering: Manipulating an organism's metabolic pathways to enhance product yields or create new products is a growing area driven by the idea of sustainability.
- Fermentation Technology: Utilizing fermentation not only for food production but also for producing chemical feedstocks is a significant aspect, leveraging naturally occurring processes to create valuable products.
Biochemical engineering is imperative in our pursuit of greener and more sustainable technologies. It allows industries to tap into biological processes, thereby reducing reliance on fossil fuels and minimizing environmental impacts.
"Thus, key areas of application in chemical engineering are not just about efficiency and production; they resonate with the long-term vision of sustainability and innovation, paving the way for a better future."
In this exploration of key areas of application, it’s clear that chemical engineers hold the tools to address many of today’s most pressing challenges. Their work is intricately woven into the fabric of our societal needs and industrial demands, creating a profound impact that extends far beyond the laboratory.
Emerging Technologies
Emerging technologies in chemical engineering present new avenues for efficiency, sustainability, and innovation. Their significance lies in the potential to revamp largely entrenched processes, making them more compatible with today’s fast-paced world where environmental concerns weigh heavily. By tapping into advancements such as nanotechnology and sustainable chemical practices, engineers are better equipped to tackle pressing industry challenges. Understanding these technologies is crucial for anyone involved in the discipline, as they open doors to smarter and greener solutions.
Nanotechnology in Chemical Applications
Nanotechnology is nothing short of a game changer in chemical engineering. It involves manipulating matter at the atomic or molecular scale, typically in the range of 1 to 100 nanometers. This scale allows for dramatic enhancements in material properties and functionalities.
Some notable applications include:
- Catalysis: Nanoparticles can enhance the speed and efficiency of chemical reactions. For instance, platinum and palladium nanoparticles are widely used in catalysts, significantly reducing the energy required for reactions.
- Drug Delivery Systems: In biochemical engineering, nanotechnology plays a crucial role in creating targeted drug delivery systems that release medication precisely where needed, minimizing side effects.
- Environmentally Friendly Materials: From coatings that repel bacteria to membranes that efficiently filter water, nanotechnology paves the way for innovative materials that address health and environmental challenges.
Nanotechnology's potential isn't just theoretical; its actual implementations are already visible in various sectors. However, careful consideration of its implications on safety and ethical standards is paramount.
Sustainable Chemical Processes
The push for sustainability in chemical engineering is louder than ever. Sustainable chemical processes aim to minimize waste, optimize resource use, and reduce the carbon footprint associated with traditional methods. The shift towards sustainability is fueled by increasing awareness of environmental issues and a global commitment to combatting climate change.
Elements of sustainable chemical processes include:
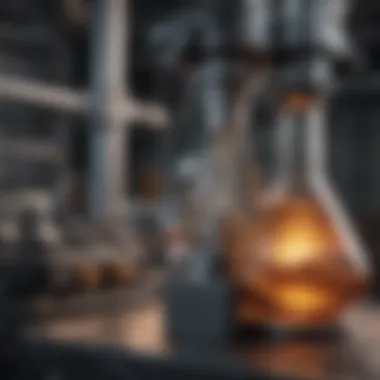
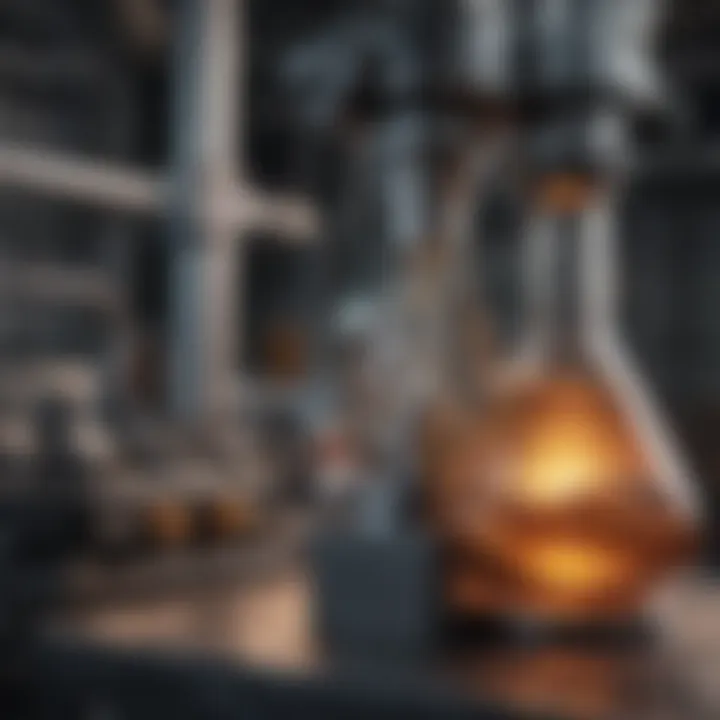
- Green Chemistry Principles: These guiding principles help in designing chemical products and processes that reduce or eliminate hazardous substances. This means working smarter, not harder, in order to achieve better results with less environmental impact.
- Waste Valorization: Instead of viewing waste as a liability, sustainable processes encourage converting waste into useful products, minimizing landfill use and leveraging resources that would otherwise go to waste.
- Renewable Energy Integration: Leveraging renewable energy sources in chemical manufacturing helps lower reliance on fossil fuels, thus contributing to a lower overall carbon footprint.
"The successful integration of sustainability into chemical engineering not only enhances efficiency but also secures the future of our planet—it's a win-win affair."
Educational Pathways
Understanding the various educational pathways in chemical engineering is crucial for anyone looking to delve into this expansive field. It involves more than just earning a degree; it's about grasping the full scope of opportunities available, the skills needed, and the significance of practical experiences. As the landscape of chemical engineering continually evolves, the educational framework must adapt to keep pace with technological advancements and industry demands. Therefore, it is pertinent to explore the existing educational structures, their benefits, and considerations.
Undergraduate Programs
Undergraduate programs in chemical engineering typically lay the foundational knowledge that future engineers need. These programs generally emphasize a blend of basic sciences, mathematics, and engineering principles, allowing students to develop essential problem-solving skills.
Programs often include courses in:
- Chemistry, Biology, and Physics
- Differential Equations and Calculus
- Fluid Mechanics and Thermodynamics
In addition to classroom instruction, practical lab experience plays an essential role in undergraduate education. Many universities provide opportunities for students to engage in hands-on projects, where they can apply theoretical concepts to real-world scenarios. This approach not only solidifies their understanding but also enhances employability after graduation. Furthermore, participating in internships or cooperative education programs allows students to gain industry experience while completing their degrees.
There are various universities with reputable undergraduate programs, such as:
- Massachusetts Institute of Technology (MIT)
- Stanford University
- University of California—Berkeley
These institutions often offer specialized tracks that can help students focus on areas like materials science, environmental engineering, or process design, aligning their education with specific career goals.
Graduate Studies and Research Opportunities
After completing an undergraduate degree, many chemical engineers choose to pursue graduate studies. A master’s or doctoral degree can open doors to more advanced positions within the industry or academia. Graduate programs typically encourage a more in-depth understanding of sophisticated concepts, such as:
- Advanced Thermodynamics
- Chemical Reaction Engineering
- Process Systems Engineering
An integral component of graduate education is research. Graduate students often engage in significant research projects, which can lead to innovations in chemicals, materials, and processes. This research experience is invaluable, particularly when applying for jobs or further academia. It's not uncommon for these students to present their findings at conferences or in academic journals, expanding their professional network and enhancing their resumes.
Moreover, involvement in interdisciplinary research programs can provide insights into how chemical engineering intersects with other fields, such as biotechnology or sustainable energy systems. Collaboration tends to yield groundbreaking ideas that can be pivotal in tackling global challenges. Institutions like California Institute of Technology (Caltech) or Georgia Institute of Technology stand out for their research-driven graduate programs that push the boundaries of traditional chemical engineering.
"An effective engineer must transform knowledge into actionable solutions, navigating real-world complexities through education and research."
Professional Landscape
The professional landscape of chemical engineering is a vibrant and dynamic realm that intertwines innovation with practical applications. Understanding this field is crucial, as it not only shapes the careers of individuals but also influences the very fabric of numerous industries. Chemical engineers are at the helm of advancements that address both societal needs and environmental challenges, emphasizing the importance of this topic in the broader narrative of engineering.
Role of Chemical Engineers in Industry
Chemical engineers play an essential role across multiple sectors. Their expertise spans from the design of chemical processes to the implementation of new technologies that enhance efficiency and sustainability. They often work in industries like pharmaceuticals, petrochemicals, food production, and environmental management. One key aspect of their role is the ability to apply principles of chemistry, physics, and biology to solve real-world problems.
Chemical engineers not only develop processes that manufacture products but also ensure that these processes are safe and environmentally friendly. For instance, in the pharmaceutical sector, they optimize drug production while maintaining rigorous safety standards. In the energy sector, they are increasingly turning their attention to renewable resources, crafting ways to harness solar and wind energy effectively.
Moreover, the collaboration between chemical engineers and other professionals, such as biologists and environmental scientists, leads to innovative solutions that push the boundaries of possibility. This interdisciplinary approach is vital in tackling challenges such as climate change and resource scarcity, proving that chemical engineering is much more than just an industrial discipline; it is a pivotal force for sustainable development.
Licensing and Certification
In the journey towards becoming a chemical engineer, obtaining the necessary licenses and certifications plays a significant role. In the United States, for example, after earning a degree in chemical engineering, candidates often pursue a Professional Engineer (PE) license. This credential not only boosts one's credibility but also opens doors to senior roles and responsibilities within organizations.
To obtain this license, candidates typically need to pass the Fundamentals of Engineering (FE) exam and then gain a requisite amount of work experience, usually four years, under a licensed engineer. Following this period, they can take the Principles and Practice of Engineering (PE) exam, specific to chemical engineering.
- Other notable certifications include:
- Certified Chemical Engineer (CCE)
- Lean Six Sigma Certification
- Project Management Professional (PMP)
These certifications can be a game changer for professionals in the field. They signify a commitment to ongoing education and professional excellence, which is essential in a field that evolves rapidly due to technological advancements and new scientific discoveries. The blend of formal qualifications and industry-recognized credentials contributes to a more competent workforce that is well-equipped to handle emerging challenges.
"The true measure of a professional is not just their technical knowledge but their ability to apply that knowledge in a meaningful way to address real-world problems."
Ethical Considerations
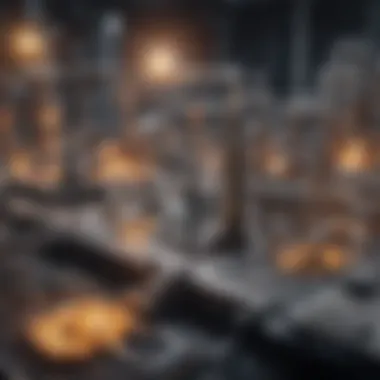
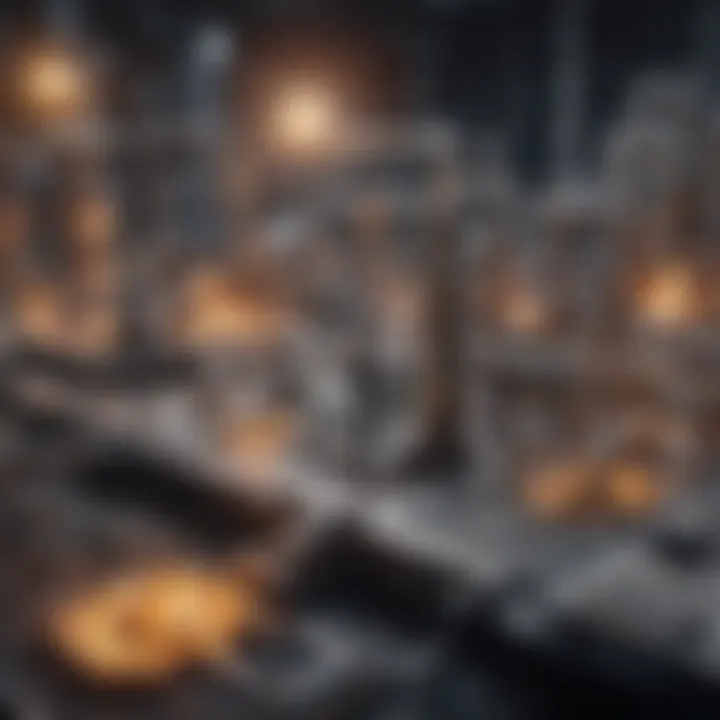
Ethical considerations in chemical engineering are not just a checklist item; they are the very bedrock upon which responsible engineering is built. As the world becomes increasingly interconnected, the need for chemical engineers to navigate complex ethical landscapes only intensifies. At the core, ethical considerations revolve around ensuring that chemical processes not only meet technical metrics but also respect societal norms and environmental limits.
One critical aspect of these considerations is environmental sustainability. The drive towards sustainable practices shapes how chemical engineers design processes that minimize waste, reduce emissions, and conserve resources. For example, when evaluating the lifecycle of a product, engineers must ponder not only the economic factors but also the long-term implications on the planet. This means incorporating renewable resources and energy-efficient technologies that align with sustainable development goals.
"Sustainability is not a choice, it's a responsibility."
Having a firm grip on environmental sustainability means being proactive rather than reactive. Chemical engineers are often in the hot seat when it comes to addressing climate change. As such, they must consider the environmental impact of their engineering choices right from the drawing board.
Environmental Sustainability
When diving into environmental sustainability, one must recognize its dual role in chemical engineering. On one hand, it serves to protect natural ecosystems and public health; on the other, it drives innovation and efficiency in designs. A chemical plant that integrates sustainable practices can not only enhance its reputation but also boost profitability through operational efficiencies.
Some key approaches to achieving better sustainability in chemical engineering include:
- Green Chemistry: Emphasizing the design of products and processes that minimize hazardous substances.
- Waste Reduction: Implementing methods like recycling and upcycling to ensure minimal waste generation.
- Energy Efficiency: Utilizing technologies that require less energy to operate, such as heat exchangers and alternative energy sources.
Moreover, engineers can explore closed-loop systems, where waste from one process becomes the raw material for another. Such circular economy principles are becoming crucial in shaping sustainable industrial practices.
Safety and Risk Management
Safety and risk management cannot be treated as mere formalities in the realm of chemical engineering. Instead, they are fundamental elements that affect the health and welfare of individuals, communities, and the environment. Every chemical engineer must grapple with the potential hazards associated with chemical processes and materials.
A robust risk management plan includes:
- Hazard Identification: Recognizing potential risks before they manifest. This could involve modeling scenarios where chemical leaks could occur and determining their consequences.
- Risk Assessment: Evaluating the likelihood and impact of identified hazards. Utilizing quantitative methods helps in gauging the severity of potential outcomes.
- Risk Control Measures: Implementing controls such as engineering solutions, administrative procedures, and personal protective equipment to mitigate identified risks.
Safety also extends beyond mere compliance with regulations. Engineers should foster a culture of safety within their organizations, encouraging open discussions about workplace hazards and empowering employees to report unsafe conditions without fear of backlash.
Future Trends in Chemical Engineering
In the rapidly evolving landscape of chemical engineering, it becomes imperative to stay abreast of future trends that could reshape the discipline. The integration of advanced technologies and collaborative efforts across borders are critical elements steering the future of chemical engineering. These trends not only enhance efficiency but also pave the way for innovative solutions to pressing global challenges.
Digital Transformation
Digital transformation encompasses the integration of digital technologies into all areas of chemical engineering, fundamentally changing how processes operate and how data is utilized.
It’s a game changer, allowing engineers to gather vast amounts of data in real time and analyze it with unprecedented precision. This shift helps in optimizing chemical processes, reducing waste, and improving safety measures by leveraging big data analytics and artificial intelligence. For instance, consider predictive maintenance — by employing sensors and data analytics, chemical plants can forewarn about potential failures before they occur, leading to significant cost reductions and minimized downtime.
Moreover, the adoption of cloud computing facilitates collaboration among teams spread across different geographical locations. Engineers can readily share data and insights, enhancing the collective intelligence of their organizations. Such transparency and accessibility are vital for complex problem-solving and advancing innovative research. Ultimately, this trend towards digitalization not only streamlines operations but also drives sustainable practices in the industry.
Globalization and Collaboration in Research
Globalization has opened up new avenues for collaboration among chemical engineers, researchers, and institutions worldwide. The modern challenges we face, like climate change and resource scarcity, require a concerted effort across national borders.
Working collaboratively fuels innovation. It brings diverse perspectives together, aiding the development of comprehensive solutions. For instance, a joint research project between institutions in the United States and Europe on reducing carbon emissions has resulted in groundbreaking carbon capture technologies. This kind of transnational partnership exemplifies the significant benefits of sharing knowledge and resources.
Furthermore, global research networks often emphasize inclusivity and cross-disciplinary approaches, linking chemical engineering with fields like biotechnology, materials science, and environmental science. These collaborative efforts amplify research capabilities and foster an environment rich in creativity.
Globalization in chemical engineering not only enhances the body of knowledge but also drives forward the imperative for sustainable industry practices.
The importance of future trends in chemical engineering cannot be understated. As we pivot towards a more interconnected and technologically advanced future, being proactive and adaptable will be central in navigating the complexities of modern engineering challenges. This journey will demand not just technical expertise, but also a willingness to work collectively and think critically about the innovations that shape our world.
Closure
The conclusion of an in-depth exploration into the field of chemical engineering is not just a wrap-up; it stands as a crucial juncture where all the threads presented throughout the article weave together. Understanding chemical engineering offers insights into how this discipline shapes the world around us, influences various industries, and contributes to addressing some of the most pressing challenges today, such as sustainability and safety.
Summarizing Key Points
The key points outlined throughout this article provide a roadmap of the expansive territory that chemical engineering covers. Here are some highlight points:
- Core Principles: Foundational concepts like mass and energy balances, thermodynamics, and transport phenomena are essential to every process in this discipline.
- Applications: The versatility of chemical engineering is illustrated through its key areas, such as process engineering, materials science, and biochemical engineering, touching various sectors from pharmaceuticals to food production.
- Emerging Technologies: Innovations like nanotechnology and sustainable practices show the pivotal role chemical engineers play in responding to modern needs while ensuring environmental preservation.
- Educational Pathways: The educational framework guiding future chemical engineers is vital. Programs at both the undergraduate and graduate levels shape the skilled professionals needed in the industry.
- Ethical Considerations: Issues surrounding safety, environmental impact, and social responsibility should not be taken lightly. The responsibility of chemical engineers extends beyond mere technical proficiency; it also involves ethical decision-making.
This synthesis encapsulates the importance of the discipline and its foundational theories, urging current and future professionals to engage deeply with the complexities of chemical engineering.
The Ongoing Evolution of Chemical Engineering
Chemical engineering is far from static; it evolves continuously in response to technological advancements, societal needs, and environmental concerns. One cannot help but notice the significant transformations that are shaping the path forward for this field. Some key developments include:
- Digital Transformation: The integration of digital tools in chemical engineering processes is making ways for precision and efficiency unheard of in the past. From simulation software to data analytics, these innovations foster improved decision-making.
- Collaboration in Research: In an increasingly interlinked world, collaboration across borders and disciplines is becoming vital. Chemical engineers find themselves working with biologists, environmental scientists, and even data analysts to develop holistic solutions.
Advancements in other areas have a profound impact on how chemical engineering is practiced. As challenges arise, the resilience and adaptability of this field shine through. As new generations of engineers take the stage, they carry with them not just technical skills but also a renewed commitment to tackling global issues that demand innovative and sustainable chemical engineering solutions.