Chemical Machines: Their Role and Impact in Science
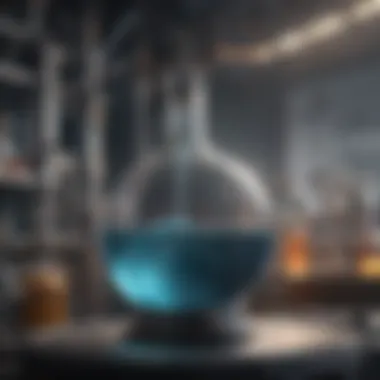
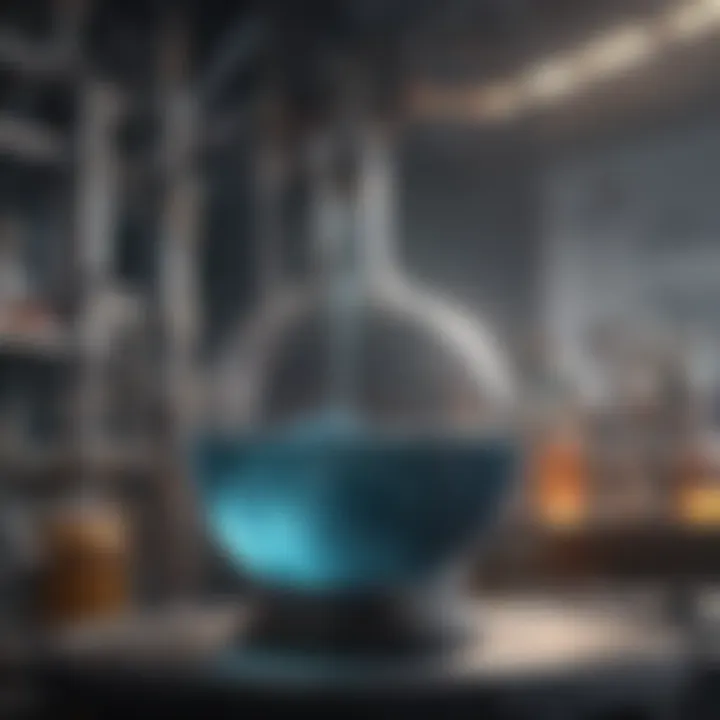
Intro
The field of chemistry has seen unprecedented advancements in recent years, and central to this evolution are chemical machines. These machines serve as fundamental tools in modern laboratories, reshaping the landscape of scientific inquiry. Their relevance spans across various domains, including chemical synthesis, material processing, and biological research. This article will provide a nuanced discourse on the principles, designs, and pressing applications of chemical machines in contemporary science.
By dissecting their operational mechanisms, we can appreciate their contributions to both theoretical and practical realms of science. Through a critical evaluation of existing literature and recent innovations, we will elucidate their role in enhancing efficiency and precision in chemical experiments. The appeal of chemical machines lies not only in their diverse applications but also in the profound impact they have on the methodology and practices within the scientific community.
Through this exploration, both established researchers and newcomers to the field can develop a comprehensive understanding of the importance of chemical machines in todayβs scientific landscape.
Research Methodology
Description of research design and approach
To understand the significance of chemical machines, a multi-faceted research methodology has been employed. The approach integrates qualitative analysis of scholarly articles, case studies, and various patent documents. This synthesis provides insights into the different designs and functionalities of chemical machines utilized across the scientific spectrum. Additionally, interviews with industry professionals and academics shed light on current challenges and opportunities within this field.
Materials and methods used in the study
Key materials analyzed include:
- Peer-reviewed journal articles focusing on chemical engineering and machine design.
- Technical specifications and operational manuals of notable chemical machines, such as those from Anton Paar and Shimadzu.
- Industry whitepapers that discuss innovations in automation and robotics in chemistry.
Data collection involved searching databases and extracting relevant information. A systematic review was conducted to ensure that the findings are comprehensive and grounded in reputable sources. By focusing on empirical evidence and theoretical frameworks, this study aims to present a well-rounded view of chemical machinesβ impact in modern science.
Discussion and Interpretation
Interpretation of results in the context of existing literature
The findings indicate a marked rise in the efficiency of chemical processes attributed to the integration of chemical machines. Several studies have documented reductions in cycle times and error rates due to automation, aligning with contemporary literature that emphasizes the shift towards mechanization in laboratories. Notably, the use of chemical machines in high-throughput screening has transformed drug development and materials science, enabling rapid experimentation that was previously unattainable.
Implications for future research or practical applications
As the role of chemical machines continues to expand, future research will focus on enhancing machine learning algorithms applied within these devices. There is also a growing interest in sustainable practices, prompting the design of machines that minimize chemical waste and energy consumption. The implications for education and industry are profound, suggesting a need for updated curricula that equip future scientists with the skills to operate advanced chemical machinery.
"The advent of chemical machines is not merely a technological advancement; it is a paradigm shift in how we perceive and conduct scientific research."
By continually adapting to the latest advancements, stakeholders can ensure that the impact of chemical machines is both far-reaching and enduring, paving the way for a more efficient and effective scientific inquiry.
Prelims to Chemical Machines
The concept of chemical machines has increasingly become vital in the realm of scientific exploration. These machines are not merely tools; they embody the evolution of chemistry through technology. Understanding chemical machines is essential for anyone involved in scientific research or industry applications. They serve a dual purpose: enhancing the efficiency of chemical processes and enabling complex reactions that might otherwise be difficult to execute manually. In this section, we will delve into the essence of chemical machines and why their study is crucial in contemporary science.
Definition of Chemical Machines
Chemical machines can be defined as devices designed to facilitate, automate, or enhance chemical reactions and processes. They can range from simple laboratory equipment, such as beakers and stirrers, to sophisticated systems like automated reactors or microfluidic devices. The main objective of these machines is to improve the precision, repeatability, and efficiency of chemical processes.
Chemical machines can be classified into several categories based on their application, functionality, and mode of operation. Each type plays a distinct role in enhancing the throughput of chemical reactions, ensuring consistency, and minimizing human error. Understanding these definitions lays the groundwork for appreciating their historical context and future implications in various scientific domains.
Historical Development
The historical development of chemical machines reflects the broader trends in scientific progress. In the early days of chemistry, particularly during the alchemical period, practitioners relied heavily on manual methods without mechanical assistance. The shift towards more systematic experimentation began in the 18th century, leading to the first basic mechanical devices for mixing and heating.
As chemistry matured into a formal science, so did the tools used by chemists. The industrial revolution in the 19th century marked a significant turning point. It ushered in the invention of machines capable of performing routine chemical tasks on a larger scale. This paved the way for innovations such as the continuous flow reactor, which allowed for more efficient processing of chemical materials.
In the late 20th century, advancements in computer technology and engineering further transformed chemical machines. With the introduction of automation and computer-controlled systems, researchers could perform complex chemical synthesis faster and with greater accuracy than ever before. Today, chemical machines continue to evolve, integrating cutting-edge technologies like artificial intelligence and machine learning to enhance their capabilities.
Understanding this historical trajectory is vital. It contextualizes the significance of the machines that scientists use today. As we explore principles, types, and applications in later sections, recognizing how these devices have progressed will aid in grasping their profound impact on modern science.
Principles of Operation
Understanding the principles of operation of chemical machines is fundamental to grasping their role in modern science. These machines are complex, yet their design and functionality are rooted in a few essential concepts that dictate their performance and efficiency. Recognizing these principles allows researchers and practitioners to manipulate variables to optimize processes and innovate new applications that push the boundaries of conventional practices.
Fundamental Concepts
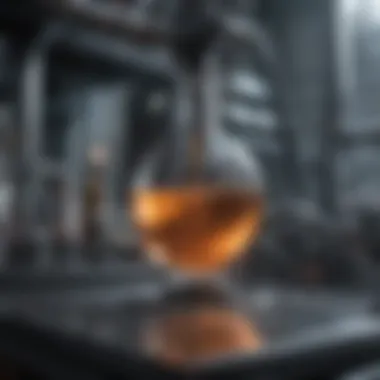
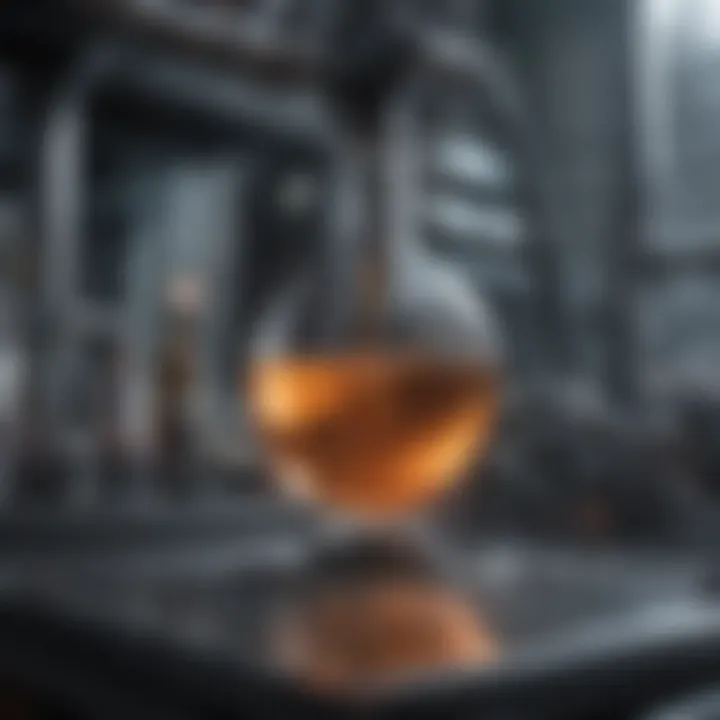
The operation of chemical machines relies on several fundamental concepts that form the foundation of chemical engineering. One key aspect is the reaction kinetics, which involves studying the rates at which chemical reactions occur. This aspect is crucial because knowing how fast reactions proceed allows engineers to design machines that maximize productivity.
Another important concept is thermodynamics, which governs energy changes during reactions. Understanding thermodynamic principles helps in assessing the feasibility and predicting the energy requirements of chemical processes.
In addition, mass transfer phenomena must be considered. This involves the movement of reactants and products within the machine, influencing the overall efficiency of the reactions. For example, in batch processing machines, ensuring efficient mixing and contact between reactants is vital for maximizing yield.
Lastly, process control is essential. Effective operation of chemical machines demands continuous monitoring and adjusting of variables like temperature, pressure, and flow rates. This ensures that the machine operates under optimal conditions, leading to consistent and desirable results.
Chemical Reaction Dynamics
Chemical reaction dynamics is a pivotal area within the study of chemical machines. It encompasses understanding how reactions occur at the molecular level and how these processes can be manipulated within an engineered system. The dynamics of chemical reactions are influenced by multiple factors, such as concentration, temperature, and catalysts.
In chemical machines, the design often integrates specific catalysts to accelerate reaction rates without being consumed in the process. This is particularly important in industrial applications where efficiency and cost-effectiveness are paramount.
Additionally, the spatial and temporal aspects of reaction dynamics must be optimized. For continuous flow reactors, ensuring that reactants are adequately mixed and come into contact throughout their transit time is critical for achieving desired conversion rates.
Moreover, advancements in computational models enable better predictions of reaction behavior in complex systems. By simulating different scenarios, researchers can evaluate various design configurations to enhance performance before actual implementation.
The integration of these elements leads to improved outcomes, ultimately enhancing the reliability and scalability of chemical machines across various applications, including pharmaceuticals, petrochemicals, and materials science.
Types of Chemical Machines
Understanding the different types of chemical machines is essential for grasping their wide application in various scientific fields. Chemical machines can be categorized based on their operational modes, designs, and functionalities. This section outlines three prominent types: batch processing machines, continuous flow reactors, and microfluidic devices. Each type plays a unique role in enhancing efficiency and precision in chemical processes, which is crucial for modern scientific advancements.
Batch Processing Machines
Batch processing machines are one of the earliest forms of chemical machines used in laboratories and industrial settings. These machines operate by processing a finite amount of material in one cycle. Their significant advantage is flexibility. Researchers can easily change parameters such as temperature and pressure for different reactions without major shifts in equipment.
Batch processing machines are particularly useful in producing small quantities of specialized chemicals, pharmaceuticals, or high-value compounds. For example, a batch reactor allows for meticulous control of reaction conditions, which can lead to improved yields.
However, the operation often involves longer processing times and may result in inconsistent quality. Despite these limitations, they remain integral for applications where precision is paramount. Thus, while batch processing is less efficient in terms of continuous output, they serve specific needs effectively.
Continuous Flow Reactors
Continuous flow reactors represent an evolution in chemical processing technology. These machines operate by keeping reactants flowing through the reactor without interruption. This leads to constant and uninterrupted production, drastically enhancing productivity compared to batch systems.
One of the primary benefits of continuous flow reactors is their ability to maintain uniform conditions throughout the reaction. Additionally, they often require less space and can improve safety through better heat management, reducing risks of thermal runaway reactions. They have gained popularity in the pharmaceutical industry, particularly for processes requiring high throughput and consistent product quality.
Yet, the initial setup and design of continuous flow systems can be complex and costly. Moreover, not all reactions can be adapted to continuous processes. Therefore, understanding the specific suitability of a reaction for continuous flow operation is essential for researchers.
Microfluidic Devices
Microfluidic devices are at the forefront of modern chemical machine technology. These small-scale systems manipulate fluids at the microliter level. The precision and control afforded by microfluidic devices opens new avenues in experiments and applications.
Microfluidics allow for rapid screening of chemical reactions, making them valuable in drug discovery and development. Their compact size promotes integration and automation in laboratories.
However, challenges exist, such as the need for specific designs tailored to particular applications. Furthermore, scaling these devices for larger production can also present difficulties. Nevertheless, the potential of microfluidics in enhancing efficiency and reducing waste is undeniable.
In summary, the various types of chemical machines showcase different operational approaches, each with its advantages and challenges. Understanding these types provides insight into their critical roles in boosting efficiency and innovation across diverse fields.
Applications of Chemical Machines
The role of chemical machines is pivotal in contemporary scientific practices. Their applications span various fields, significantly enhancing processes that involve chemical transformations, manufacturing, and biological experimentation. Understanding these applications can yield insights into efficiency gains, innovation, and future directions in research and development.
Chemical Synthesis
Chemical synthesis is a fundamental process in chemistry and related fields. Chemical machines streamline this process by automating reactions, allowing for precise control over conditions such as temperature and pressure. This increased efficiency translates into higher yield and purity of products. Machines like batch reactors and continuous flow reactors play crucial roles in industrial-scale chemical production. Their ability to operate under controlled environments minimizes risks associated with human error, ultimately leading to safer operations. Moreover, advancements like computer-aided synthesis have opened new avenues for optimizing reaction pathways.
"Automation in chemical synthesis not only accelerates production but also fosters innovative approaches to previously seen limitations in reaction conditions."
Key benefits of chemical synthesis applications include:
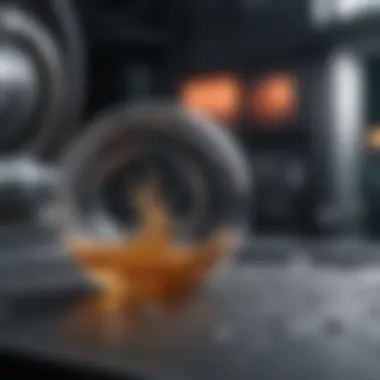
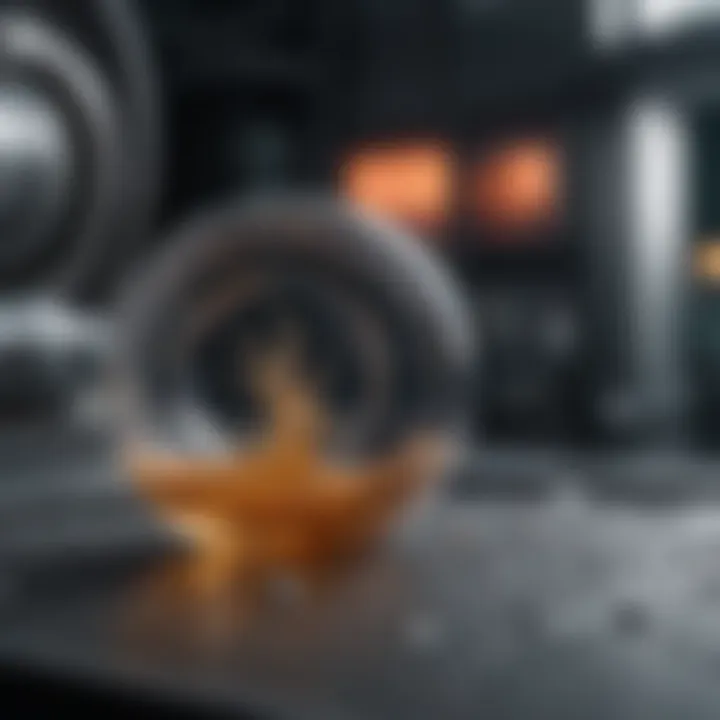
- Reduced Time: Automation speeds up reaction times.
- Enhanced Control: Machines offer refined control over parameters, improving outcomes.
- Increased Safety: Reduced human intervention decreases accident risks.
Material Fabrication
The fabrication of materials often hinges on chemical processes. Chemical machines are integral in producing polymers, nanomaterials, and other advanced substances. Depending on the desired properties of the final product, these machines can be programmed to adjust parameters dynamically. Processes such as chemical vapor deposition and spinning mills are facilitated by chemical machines, allowing for the manufacture of high-quality materials with specific characteristics. The importance of material fabrication extends into sectors such as electronics, construction, and automotive industries.
Unimaginably, this technology not only aids in creating new materials but also contributes to recycling existing ones, combining sustainability with innovation.
Biotechnological Uses
Biotechnology is an area where chemical machines show tremendous potential. They support a variety of applications, from pharmaceutical production to agricultural improvements. Devices like bioreactors allow for the cultivation of microorganisms under optimal conditions for the production of enzymes, vaccines, or antibiotics. With the rise of personalized medicine, chemical machines are adapting to manufacture specific formulations tailored to individual needs.
In agriculture, these machines help produce biofuels and biopesticides, minimizing reliance on synthetic chemicals that can harm ecosystems.
Overall, chemical machines exemplify the confluence of technology and biology, leading to innovative solutions for pressing scientific and environmental challenges.
Advantages of Chemical Machines
The landscape of modern science has greatly benefited from the implementation of chemical machines. Their advantages are multifaceted, influencing efficiency, innovation, and the overall progression of scientific research. In this section, we will explore the two critical elements that underscore their importance: efficiency improvements and innovation in research methodologies.
Efficiency Improvements
Chemical machines are designed to optimize various processes within scientific disciplines. These machines automate procedures, reducing human error and expediting workflows. With enhanced precision, they ensure consistent results, a factor crucial for experiments that require high reliability. For instance, the accuracy in dosage and timing during chemical reactions managed by machines like continuous flow reactors can lead to superior product yields.
- Time-saving: The automation of processes reduces the time required for experiments. Researchers can focus more on analysis and less on the repetitive tasks traditionally performed manually.
- Resource utilization: Chemical machines often employ resources more effectively. By minimizing waste and improving yield rates, they contribute to more sustainable practices within labs.
"The use of chemical machines streamlines research, significantly improving throughput and allowing scientists to explore new avenues previously thought impractical."
Furthermore, the adaptability of these machines allows them to handle varying scales of production, from small-scale laboratory syntheses to larger industrial processes. This scalability means that researchers have greater flexibility in their work, and they can test hypotheses more efficiently without needing extensive manual intervention.
Innovation in Research Methodologies
The advent of chemical machines has propelled innovation in research methodologies. These devices enable scientists to explore previously inaccessible realms of chemical analysis and synthesis. For instance, microfluidic devices give researchers the ability to conduct experiments on a microscale, enabling the study of reaction dynamics with unprecedented precision.
- Enhanced experimental design: Chemical machines facilitate complex experimental designs that were not feasible without automation. They allow researchers to perform high-throughput screening of compounds, which is vital for drug discovery and material science.
- Real-time data collection and analysis: Many chemical machines come equipped with sensors and data processing technology. This capability enables real-time monitoring of reactions, significantly enhancing the understanding of chemical processes.
In addition, the integration of artificial intelligence with chemical machines holds promise for future advancements. AI-driven analyses can lead to new insights and unexpected discoveries, reshaping traditional methodologies.
In summary, the advantages of chemical machines in modern science are not just practical; they are transformative. As efficiency improves and innovation flourishes, these machines have become essential tools in the ever-evolving landscape of research.
Challenges in Chemical Machine Design
The design of chemical machines presents multifaceted challenges that can significantly affect their performance and application. Understanding these challenges is essential for advancing research and improving the effectiveness of chemical machines. The field demands not only extensive technical knowledge but also an understanding of various operational considerations. By addressing the difficulties in design, it is possible to align these machines with the ever-evolving needs in science and industry.
Technical Limitations
Chemical machines must operate under a variety of conditions, which can impose strict limitations. One major aspect is the scalability of designs from laboratory prototypes to industrial applications. Technologies that work well in controlled environments may face unpredictable behaviors when functioning at larger scales. These discrepancies can lead to inefficiencies or even failures.
Moreover, the materials used in constructing these machines often have limits in terms of temperature and pressure resistance. For instance, certain reactions may require extreme conditions that standard materials cannot endure. This limitation necessitates constant innovation in material science to find more resilient options.
Additionally, the automation systems that control these machines must be adept at managing complex chemical reactions. This involves sophisticated sensor technologies and feedback mechanisms. If these systems are not precisely designed and calibrated, they can cause serious disruptions in the chemical production process.
Cost-Benefit Analysis
Cost considerations are pivotal when designing chemical machines. A thorough cost-benefit analysis helps in determining the viability of a project. The initial investment in advanced machinery can be substantial, but it is crucial to compare this against the potential long-term benefits. For example, more efficient machines may have higher upfront costs but can drastically reduce operational expenses through lower energy consumption or minimized waste production over time.
When analyzing costs, it is also essential to factor in the lifecycle of the machine. Maintenance costs can accrue quickly, requiring a thoughtful approach to both design and material selection. Cheaper materials may save money initially but can lead to increased maintenance or even early machine failures.
Furthermore, market demands must be evaluated. The success of a chemical machine is also contingent upon its ability to deliver products that meet or exceed current market requirements. A machine that fails to be adaptable and efficient may end up being a financial burden rather than an asset.
A thorough understanding of both technical limitations and cost-benefit dynamics is necessary to improve the design and effectiveness of chemical machines in various applications.
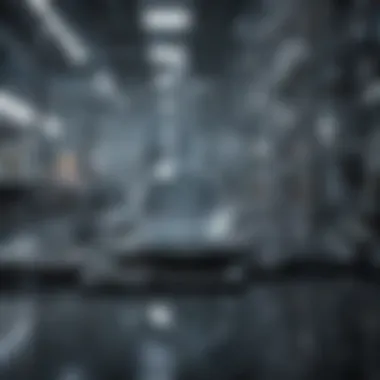
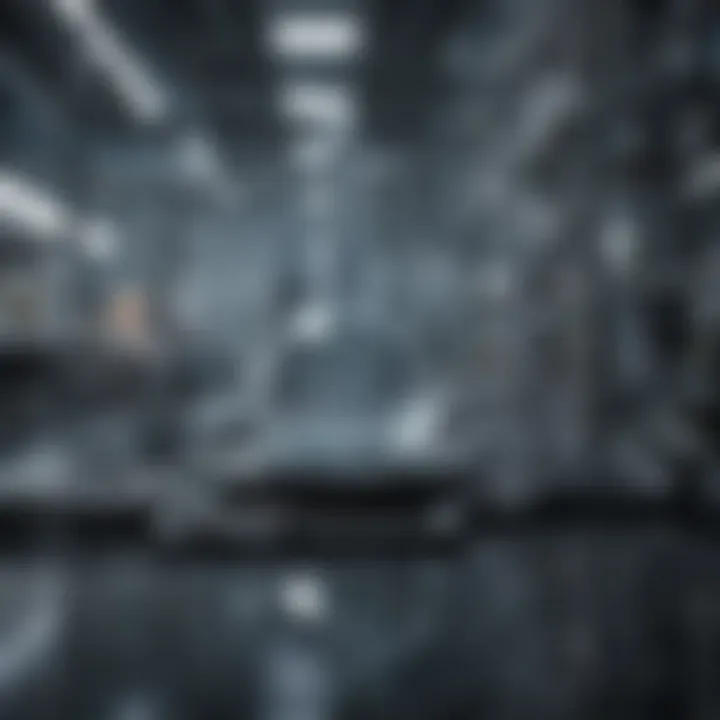
Evaluating these challenges allows for a progression in design methodologies that can lead to significant advancements in chemical engineering fields.
Future Perspectives
The exploration of future perspectives in the realm of chemical machines is essential for understanding their evolving role in science. As we look ahead, we can anticipate significant advancements shaped by technological progress and heightened environmental consciousness. These innovations not only enhance the capabilities of chemical machines but also emphasize sustainable practices in their operations.
Technological Innovations
Technological innovations are at the forefront of the evolution of chemical machines. The continuous integration of artificial intelligence and machine learning into chemical engineering processes streamlines operations and improves accuracy. For instance, algorithms can optimize reaction conditions in real-time, leading to better yield and reduced waste.
Moreover, advancements in sensors allow for more precise monitoring of chemical reactions. This precision reduces the risk of unsafe reactions and enhances overall efficiency. Microfluidic technology also plays a crucial role by enabling small-scale, high-throughput experimentation. This allows for rapid testing of multiple conditions which can drastically shorten development times.
Additive manufacturing or 3D printing has also emerged as a disruptive technology in chemical machine design. This method allows for the fabrication of complex geometries that can improve flow dynamics and reaction efficiencies. In summary, technological innovations are integral to making chemical machines more effective and versatile, driving both the scientific community and the industry toward new heights.
Sustainability Considerations
Sustainability considerations in the design and operation of chemical machines have become increasingly important. The global push for environmentally friendly practices means that chemical processes must minimize their ecological footprint. This encompasses everything from resource usage to waste production.
One key aspect is the development of greener solvents and reagents. Chemical machines can be designed to use these sustainable materials, thus reducing harmful emissions.
The life-cycle perspective also plays a pivotal role. By examining the entire lifespan of chemical machines, from production to end-of-life, researchers can identify ways to reduce energy consumption and material waste.
"Sustainable practices are essential not just for compliance but for the very survival of industries as we know them."
Moreover, recycling and reusing materials within chemical processes can significantly lower the impact on natural resources. Companies are now leveraging circular economy principles to extend the lifecycle of chemical machines and their components. Integrating sustainability into the core of chemical machine operations is not merely an ethical consideration; it is a strategic advantage that can lead to innovation and market leadership.
Case Studies
Case studies serve as a pivotal element in understanding the practical applications and implications of chemical machines in real-world scenarios. They not only illustrate the effectiveness of these devices but also highlight the intricate processes involved in their functioning. By reviewing specific instances where chemical machines have been successfully integrated into various fields, researchers and practitioners can gain insights into best practices, operational efficiencies, and innovation pathways.
Successful Implementations
Successful implementations of chemical machines in industries such as pharmaceuticals, energy production, and environmental science showcase their versatility and impact. For example, the creation of efficient reactors for drug synthesis has streamlined production processes, significantly reducing time and costs.
- Pharmaceutical Manufacturing: Companies like Pfizer have successfully utilized continuous flow reactors. These systems allow for more consistent reaction conditions. This results in better yield and control over chemical processes.
- Renewable Energy Sector: In biofuel production, chemical machines aid in the conversion of biomass into energy. Facilities using integrated bioreactors have seen improved output and minimized waste, contributing to sustainability goals.
- Environmental Remediation: Chemical machines play a role in soil and water treatment technologies. Specific case studies show their effectiveness in breaking down pollutants, making them valuable for environmental protection efforts.
Through these successful cases, we understand how chemical machines can drastically improve both quality and efficiency in processes that are vital to modern-day demands.
Lessons Learned
When examining the case studies, several lessons become evident, emphasizing the importance of adaptability and continuous improvement.
- Customization is Key: Different applications require tailored designs. The interaction between chemical machines and materials can be unique. Case studies highlight that a one-size-fits-all approach often leads to suboptimal results.
- Monitoring and Control Systems: The need for advanced monitoring technologies is evident. Successful implementations showed that integrating smart sensors can provide real-time data. This enhances control, reduces errors, and ensures safety in chemical processes.
- Collaboration Across Disciplines: Many effective projects arose from collaboration between chemists, engineers, and environmental scientists. There is a clear advantage in pooling expertise from diverse fields to address complex challenges.
- Sustainability Considerations: Recent studies emphasize the importance of eco-friendly practices. Implementations that prioritized sustainability not only benefitted the environment but also gained positive market reception, highlighting a growing consumer preference for green solutions.
Through careful consideration of thses lessons, future developments in chemical machines can continue to progress, aligning with industry needs and global sustainability goals.
Closure
Summative Insights
From the beginning, we explored the myriad functionalities of chemical machines. Their capability to enhance productivity and precision is evident in applications ranging from chemical synthesis to material fabrication. Moreover, they offer a bridge between theoretical research and practical implementation. This relationship demonstrates their significance in not just advancing individual studies but in the broader context of scientific progress.
A summary of key points includes:
- Efficiency in chemical processes, leading to reduced waste and increased yield.
- Technological Innovations that allow for fine-tuned reactions and real-time monitoring.
- Versatility across various applications, such as in biotechnology and pharmaceuticals.
These insights reveal that chemical machines are not mere tools; they are essential enablers for modern scientific inquiry and development.
Call for Further Research
Despite the substantial advances made, there remains a pressing need for further research in the domain of chemical machines. Scientific challenges continue to evolve, and there is a constant push toward sustainability and efficiency. Future investigations could focus on:
- Enhancing the integration of artificial intelligence in machine operation to enable smarter process management.
- Developing more sustainable chemical machines that minimize environmental impact.
- Exploring novel materials for machine construction that could expand capabilities and reduce costs.
"Research in chemical machines is not just about improvement; itβs about revolutionary changes that could redefine entire fields of study."
It is therefore crucial for researchers, educators, and industry professionals to collaborate in this area. As sharp minds innovate and ideate, the potential benefits for society, the economy, and the environment can be significant. Encouraging this discourse will help to unveil solutions to the various complexities faced in modern chemistry and related fields.