A Comprehensive Look at Electric Car Batteries
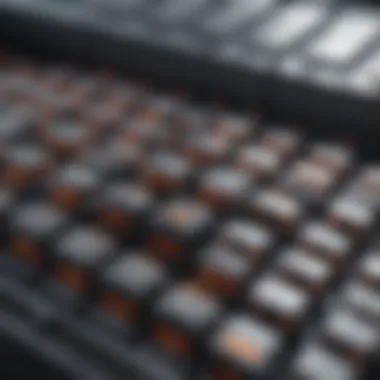
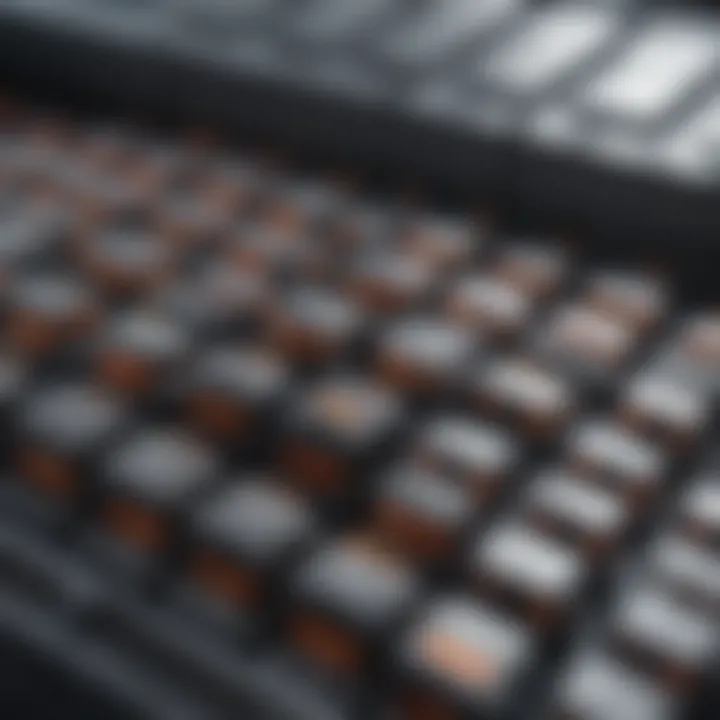
Intro
Electric vehicles (EVs) have transformed the way we think about transportation. The engine behind this revolution is not analog, but rather a sophisticated system composed of various components. Electric car batteries are at the heart of this engineering marvel, playing a pivotal role in powering vehicles that promise sustainability and efficiency. In this exploration, we will delve into the intricate world of electric car batteries, shedding light on their physical characteristics, construction, and technological innovations.
Understanding these batteries goes beyond mere technical specifications; it encompasses the very essence of future mobility. This journey will clarify which types of batteries are prevalent, the performance metrics that define them, and the raw materials integral to their creation. The interplay of chemical reactions and electrical principles will also be examined. Moreover, as the field races towards greener solutions, we’ll discuss emerging trends that aim to boost efficiency while considering the ecological footprints of these innovations.
The aim here is to cultivate a well-rounded perception of electric car batteries—not just as a component, but as a dynamic system that is set to evolve in ways we may not yet fully comprehend. Buckle up, as we embark on this insightful expedition!
Prelims to Electric Car Batteries
The significance of electric car batteries cannot be overstated. As we steer towards a more sustainable future, these batteries are at the forefront of innovation in the automotive industry. Understanding their anatomy is fundamental for grasping how electric vehicles function. The intricate design, coupled with emerging technologies, ensures that as electric cars evolve, so do their batteries. This portion of the article aims to illuminate the role and significance of these power sources in the exciting world of electric mobility.
The Rise of Electric Vehicles
In recent years, electric vehicles (EVs) have transitioned from niche products to mainstream automotive options. Environmental concerns, soaring fuel prices, and technological advancements all contribute to this shift. According to various reports, the global electric vehicle market has seen an expansive growth trajectory, with projections indicating that sales could reach into the millions within the next decade.
Consumers are increasingly aware of their carbon footprints, and many are considering electric alternatives. Countries around the world are also implementing stricter emission regulations, which further bolsters the demand for EVs. The increased production and purchase incentives from governments also play a crucial role in this rise. All these factors together shape a new landscape in which electric vehicles are now vying for a sizable share of the automotive market.
The Role of Batteries in Electric Cars
Batteries lie at the heart of electric cars, serving as the energy storage systems that power them. The performance, longevity, and efficiency of these vehicles hinge significantly on their batteries. Without advancements in battery technology, electric vehicles would struggle to compete with traditional gasoline-powered cars.
Electric car batteries manage several key functionalities:
- Energy Storage: They store energy generated from external sources and release it to power the vehicle. This process is vital for achieving adequate range and performance.
- Power Delivery: High discharge rates are mandatory to deliver power to the electric motor to ensure a responsive and dynamic driving experience.
- Cycle Life and Durability: The lifespan of a battery affects the total cost of ownership. Technologies that enhance cycle life are critical for consumer satisfaction.
"Batteries are the lifeblood of electric mobility; without them, we wouldn't move forward toward cleaner skies."
As we proceed through this article, we'll dive deeper into various aspects of electric car batteries, exploring their physical structures, performance characteristics, and even the materials used in their production. With a clearer picture of their anatomy, readers will better appreciate the technological marvel that is the modern electric vehicle.
Physical Structure of Electric Car Batteries
Understanding the physical structure of electric car batteries is crucial for comprehending how these power sources function and their long-term performance. The design and configuration dictate both efficiency and reliability, which ultimately impacts the overall usability of electric vehicles. This section peels back the layers, so to speak, on how these batteries are assembled, the types of components involved, and why such intricacies matter.
Battery Pack Configurations
When it comes to battery packs, configuration is a critical concept. Essentially, battery packs are comprised of multiple cells assembled in series or parallel to achieve desired voltage and capacity. Common configurations include:
- Series Configuration: Here, cells are connected end to end, which increases voltage but keeps capacity the same.
- Parallel Configuration: This arrangement connects cells side by side, raising capacity without altering voltage.
The decision on configuration impacts several factors. For instance, series packs are beneficial for reaching high voltage levels, essential for efficient energy transfer. However, they might be more susceptible to issues if even one cell fails, which can lead to a domino effect. In contrast, parallel configurations can provide redundancies that improve reliability, but may require more space, which could be a consideration for compact electric vehicles.
Another point to mention is thermal management, especially in high-performance setups. Batteries generate heat during operation, and their arrangement can either mitigate or exacerbate overheating. Therefore, manufacturers often implement cooling systems that circulate liquids or air, taking into account how the banks are laid out.
Battery architecture can significantly influence performance attributes like weight, volume, and charging capability.
Cell Types and Sizes
The cells themselves are the heart of the battery, and their types directly determine performance metrics like energy density and lifespan. There are primarily three types of cells seen in electric vehicles:
- Cylindrical Cells: These often resemble a battery you might put in a flashlight. Their shape allows for greater packing density and better cooling, making them popular in performance-oriented applications.
- Prismatic Cells: These flat, rectangular cells often require more sophisticated battery management systems, but they can be tailored to fit snugly into a vehicle's design, optimizing space utilization.
- ** pouch Cells:** Flexible and lightweight, pouch cells are particularly useful in design-specified situations. However, they may pose challenges during thermal management due to limited structure integrity.
The size of these cells varies, and while larger cells can generally hold more energy, they may take longer to charge or discharge. The ratio of size to energy retention is a balancing act that engineers must navigate. For example, Tesla has leveraged cylindrical cells for their high-efficiency models, while manufacturers like Nissan primarily utilize prismatic cells for easier integration.
In summary, understanding the physical structure of electric car batteries—such as their configurations and cell types—illuminates the complexity of electric vehicle design. It isn’t just about making a car go; it's about enabling a comprehensive experience that meets consumer demands while integrating cutting-edge technology.
Components of Electric Car Batteries
Electric car batteries are far more than just a power source; they form the very backbone of the electric vehicle (EV) ecosystem. Each component plays a critical role, influencing the overall performance, efficiency, and longevity of the battery system. Understanding these components helps to grasp how they interconnect, ultimately shaping the user experience and the vehicle's functionality.
Electrolytes
Electrolytes are pivotal in facilitating the movement of ions between the anode and cathode during charge and discharge cycles. The most common types used in lithium-ion batteries are lithium salts dissolved in organic solvents. However, researchers are exploring alternatives that offer higher efficiency and safety.

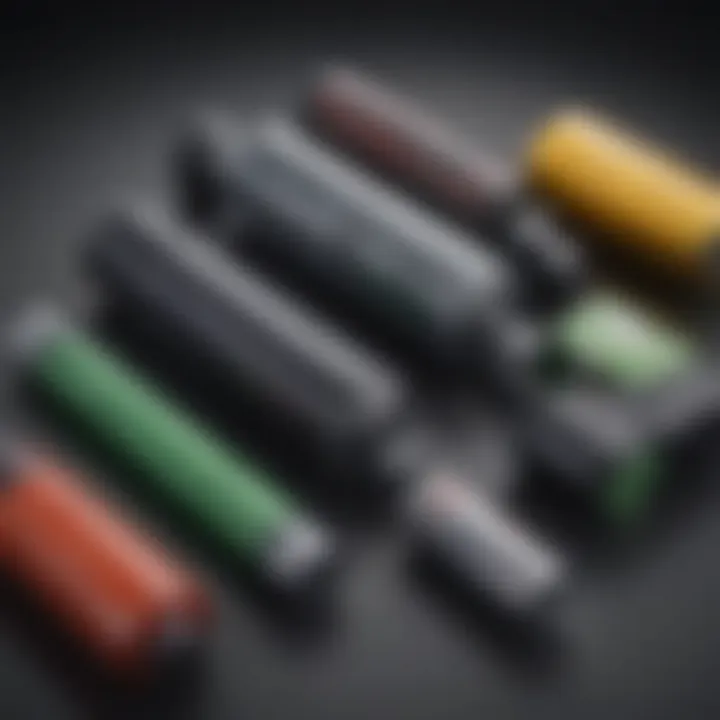
The right electrolyte can significantly affect energy density and temperature stability. For instance, solid electrolytes promise to enhance safety compared to traditional liquids, as they are less flammable.
Moreover, the viscosity of the electrolyte influences internal resistance. Higher viscosity might slow down ion movement, thus reducing performance under high-drain conditions. Understanding the trade-offs in electrolyte selection can lead to performance improvements and longer battery life.
Anodes and Cathodes
Anodes and cathodes are the heroes of the battery story, essentially acting as the negative and positive ends, respectively. The materials used in these electrodes are fundamental to how well the battery performs.
For anodes, graphite has been the go-to choice for many years, but there's been a growing interest in silicon-based materials. Silicon can theoretically store nearly ten times more lithium ions, leading to higher energy densities. However, it also undergoes significant expansion during charging, which can lead to structural failure over time. This creates a need for innovative designs that can harness silicon’s advantages while mitigating its drawbacks.
On the cathode side, lithium-cobalt oxide is common in consumer electronics, while lithium iron phosphate is becoming popular in electric vehicles due to its thermal stability and long lifespan. Each material choice comes with its unique set of performance metrics, including energy output, longevity, and cost considerations.
Example of Anode and Cathode Materials:
- Lithium Cobalt Oxide: High energy density, but limited thermal stability.
- Lithium Iron Phosphate: Lower energy density, but greater safety and longevity.
- Silicon-based Anodes: Higher capacity but prone to degradation.
Battery Management Systems
Battery Management Systems (BMS) are the unsung heroes of electric car batteries, managing the battery’s operation to ensure optimal performance. These systems monitor voltage, current, temperature, and state of charge. A BMS essentially acts like the brain of the battery, making decisions that can prolong its life and enhance safety.
A well-designed BMS prevents overheating, overcharging, or discharging too much, aiming to maintain a balanced state among the cells. With the increasing adoption of battery technologies like parallel and series configurations, the role of BMS has become even more critical to facilitate effective management. Furthermore, advanced BMS can enhance the overall efficiency by employing predictive algorithms to improve charging rates or cycle times.
The benefits of an effective BMS can be summarized below:
- Increased Safety: Reduces risks of fires or explosions.
- Extended Life Span: Helps in maintaining optimal operating conditions for the battery.
- Performance Optimization: Adaptively manages energy usage based on driving patterns.
"The effectiveness of a Battery Management System can determine the success of electric car batteries."
Types of Batteries Used in Electric Vehicles
Electric vehicles (EVs) significantly hinge upon the types of batteries used. This section dives into the three primary categories that dominate the market today: lithium-ion, nickel-metal hydride, and solid-state batteries. Each type has its unique characteristics, advantages, and challenges. Understanding these distinctions will help in comprehending how they influence both performance and sustainability of electric cars.
Lithium-ion Batteries
Lithium-ion batteries have arguably become the star of the show in electric vehicles. Their ability to pack a punch in terms of energy density — the amount of energy stored in a given volume or weight — stands out. These batteries offer lightweight solutions, which significantly enhance vehicle efficiency.
One could look at it like finding a perfect pair of shoes. You don't just want them to look good; they must also be comfortable for long walks. Similarly, lithium-ion batteries provide that balance between functionality and performance. They are used in models of Tesla, Nissan Leaf, and many more.
However, it’s not all smooth sailing. These batteries have a few quirks. Over time, lithium-ion batteries do face degradation, which leads to reduced capacities. Factors such as temperature and depth of discharge can impact their lifespan. Care for them has to be smart — it’s not a set-it-and-forget-it deal. Keeping them cool under the hood, literally, makes all the difference.
Nickel-Metal Hydride Batteries
Then, we have nickel-metal hydride batteries — once the go-to choice for hybrids, now often overshadowed by their lithium-ion counterparts. They tend to be more forgiving to environmental influences. They can handle a wide temperature range that makes them suitable for various climates. This reliability makes them favorable for users who live where extreme weather can take a toll on battery function.
While NMH batteries are not as energy-dense as lithium-ion varieties, they offer a longer lifespan and are less prone to the thermal runaway phenomena that can plague lithium-ion designs. For instance, Toyota Prius relies on these batteries and has built a loyal following by delivering dependability along with fuel efficiency.
That said, the trade-off is weight. Nickel-metal hydride batteries tend to weigh more, which can influence the overall efficiency of the vehicle. So while they might not be the flashiest, they're like an old friend — dependable, but perhaps lacking a bit in the agility department.
Solid-State Batteries
Lastly, solid-state batteries are like the new kids on the block, with the potential to redefine how we think about energy storage. These batteries use a solid electrolyte instead of liquid ones, improving safety and performance. Think of it as upgrading from a bike to a car — increased speed and reliability.
The key advantages are significant: higher energy density, faster charging times, and improved safety with a decreased risk of fire. Companies like QuantumScape are working hard to bring these batteries to the forefront of industry innovation.
An important note: even with all this promise, solid-state technology is still in the experimental phases for widespread adoption. They might take a few years before fully entering the mainstream market. Yet, if they can deliver on their promises, they could become the driving force behind the next generation of electric vehicles.
In summary, understanding the different types of electric vehicle batteries is crucial in realizing how they affect vehicle efficiency, range, and safety features. Choosing the right battery type can lead to a profound impact on the overall consumer experience and contribute to the ongoing evolution of electric transportation.
Performance Characteristics
The performance characteristics of electric car batteries play a vital role in determining how effectively these vehicles operate. Understanding these elements not only helps in evaluating existing battery technologies but also sets the stage for future innovations. Performance aspects like energy density, charge and discharge rates, and lifespan can significantly influence user experience, range anxiety, and the overall sustainability of electric vehicles.
Energy Density and Capacity
Energy density refers to the amount of energy a battery can store relative to its weight or volume. Higher energy density means that a battery can deliver more energy for a given space, contributing to extended driving ranges for electric vehicles without increasing vehicle size or weight unnecessarily.
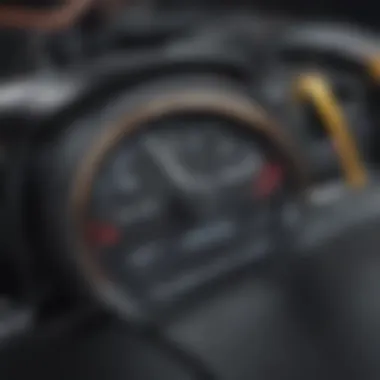
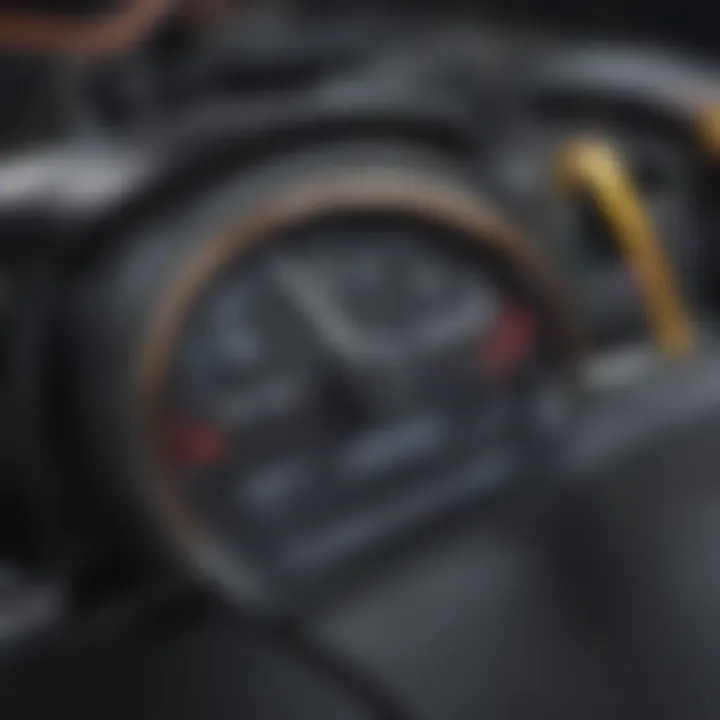
A key element of energy density is the chemistry used within the battery. For instance, lithium-ion batteries currently dominate the market due to their relatively high energy density compared to nickel-metal hydride batteries. As a result, electric cars powered by lithium-ion batteries are typically lighter and can travel longer distances per charge, making them more appealing to consumers.
However, energy capacity is not the only consideration. Quality of energy delivery and efficiency during usage are crucial. Electric car batteries must not only store energy but also do so effectively while minimizing losses during both charging and discharging cycles.
Charge and Discharge Rates
The rate at which an electric car battery can be charged or discharged significantly impacts its usability. Charge rates are typically measured in terms of how quickly a battery can be replenished, while discharge rates refer to how quickly energy can be used by the vehicle.
Charging stations vary dramatically; some can replenish a battery to full capacity in just a few hours, whereas others might take the whole day. Fast charging technology is therefore a vital area of innovation in the EV world. Users are typically looking for a balance where they can quickly plug in without suffering from overheating or reduced battery life due to rapid cycling.
Discharge rates also play a significant role in performance, especially when it comes to acceleration and the ability to maintain speed on inclines. High discharge rates are necessary for instant power to the motors. Upgrading battery technology can improve both charge and discharge rates, making the vehicle more efficient in a variety of driving conditions.
Lifespan and Degradation
Battery lifespan refers to the total number of charge and discharge cycles before a battery's capacity significantly diminishes. As batteries undergo repeated cycles, they naturally degrade—a process influenced by factors such as temperature, charge cycles, and even the depth of discharge.
Understanding how long a battery lasts before requiring replacement is essential for consumers. Lithium-ion batteries, for example, can typically last for 8-15 years, depending on their use and care. Yet, it’s crucial to also focus on ways to slow down this degradation process. Research into battery management systems allows for monitoring and optimizing conditions to enhance longevity.
"The effectiveness and longevity of electric car batteries are not just technical issues; they define our transition to sustainable transportation."
As electric vehicle adoption increases, ongoing research into improving energy density, optimizing charge and discharge rates, and extending lifespan will be pivotal. The quest for high-performing batteries remains at the forefront of sustainable automotive solutions. This ongoing evaluation and innovation will undoubtedly shape the future of electric vehicles.
Manufacturing Processes and Materials
The manufacturing processes and materials involved in electric car batteries sit at the heart of their performance and sustainability. As the electric vehicle sector expands, understanding the nuances of battery production becomes crucial. The industry is constantly evolving, aiming for higher efficiency, durability, and lower environmental impact. Focused efforts on refining manufacturing techniques can lead to batteries that not only last longer but also utilize fewer resources.
Raw Materials Sourcing
Sourcing raw materials is a critical first step in battery production. The backbone of electric car batteries lies in the materials like lithium, cobalt, nickel, and manganese. Each of these minerals brings unique properties that affect the overall battery performance. For example, lithium is prized for its lightweight and high energy density, making it a prime candidate for modern electric vehicles.
However, the quest for these materials can sometimes lead to ethical and environmental dilemmas. Many lithium mines, notably in regions like Bolivia and Chile, have faced scrutiny regarding their impact on local communities and ecosystems. When selecting suppliers, manufacturers must consider the mining practices and labor conditions.
Incorporating sustainability in raw materials sourcing not only builds a strong brand image but also aids in regulatory compliance. As governments worldwide amp up environmental regulations, sourcing materials responsibly ensures companies stay ahead of the game.
Production Techniques
Once the materials are sourced, how they're processed into actual batteries is paramount. The production techniques can greatly influence both the efficiency and reliability of the battery packs. Most electric car batteries today are assembled in highly automated factories to maintain stringent quality controls and minimize human error.
A common method employed is the dry coating process, which uses less binder and solvent compared to traditional methods. This helps in achieving higher energy density while reducing production costs. Additionally, advances in manufacturing technologies like additive manufacturing, also known as 3D printing, offer the potential for customization and on-demand components.
Moreover, innovations such as the incorporation of novel chemistries, like sodium-ion batteries, are being explored to complement or replace lithium-ion options. These new extraction processes can have a promising impact on reducing dependency on scarce materials.
Quality Control Measures
Quality control is the final line of defense in ensuring battery reliability. When it comes to electric vehicles, the stakes are high. Every battery must pass through a thorough quality control system to detect defects, which could lead to battery failures or safety hazards.
Manufacturers employ various testing methods, such as temperature cycling and electrochemical impedance spectroscopy, throughout the battery's lifecycle. For instance, the temperature cycling test subject batteries to extreme temperature fluctuations, ensuring they can withstand real-world conditions.
Furthermore, certifications from organizations like UL or ISO enable manufacturers to validate their products against standardized benchmarks. This not only reassures customers about the safety of their vehicles but also strengthens the manufacturer's market position.
"As the landscape of electric vehicle technology continues to evolve, investing in robust manufacturing processes is key to unlocking better battery performance and sustainability."
These quality control measures serve as a commitment to excellence, a promise that any battery powering an electric car meets the highest standards of performance and safety.
Innovations in Battery Technology
The significant strides in battery technology over recent years cannot be overstated. This topic is crucial as it encompasses the continual enhancement of electric vehicle performance, efficiency, and sustainability. Innovations are not just matters of convenience; they directly impact the operational costs and environmental footprint of electric vehicles. In times when the world is grappling with climate change, reevaluating energy consumption through advanced battery tech becomes indispensable.
Advancements in Energy Storage
The progress in energy storage solutions has witnessed innovative breakthroughs aimed at enhancing battery capacities while reducing charging time. One notable development is the introduction of lithium-silicon batteries, which promise a far higher energy density compared to conventional lithium-ion counterparts. This higher density translates into longer range for electric vehicles, something every driver seeks.
Furthermore, companies are exploring the use of ultra-capacitors, which store energy much quicker than traditional batteries. This could mean a future where electric cars not only recharge faster but also utilize less energy overall. Research into flow batteries also holds potential, providing a different method for storing energy using liquid electrolyte solutions, which could be scaled for larger applications.
"The future of energy storage is all about maximizing efficiency and sustainability," says a leading researcher in the field.
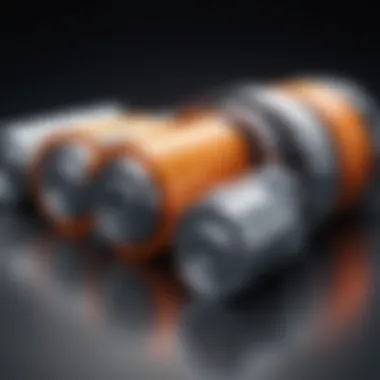
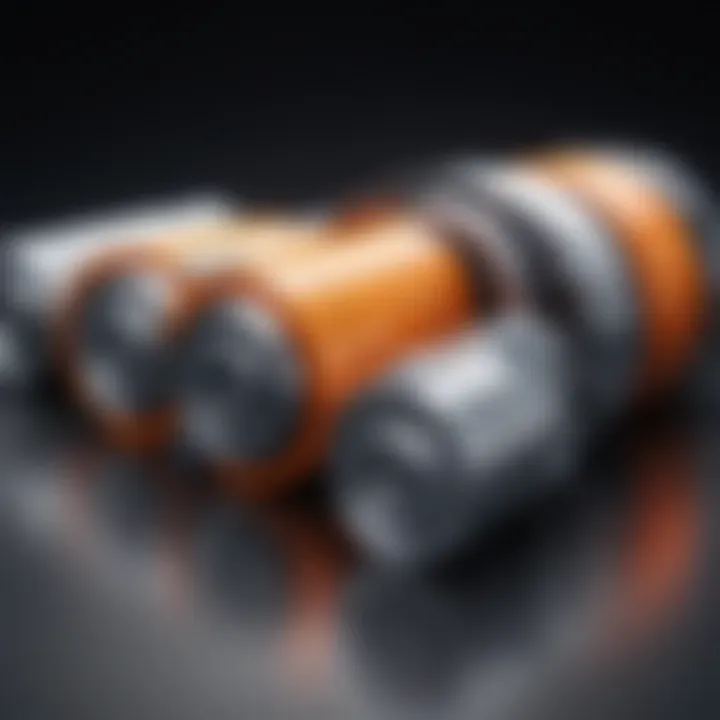
Recycling and Sustainable Practices
As the landscape of electric mobility expands, recycling practices for used batteries gain prominence. The environmental implications of battery disposal have sparked innovations in recycling methods, reducing the overall ecological footprint of electric car batteries.
Manufacturers are increasingly focussing on closed-loop systems, wherein the materials from old batteries are reused to create new ones. Not only does this conserve raw materials, but it also mitigates the mining impacts on the environment. Companies such as Redwood Materials are pioneering in battery recycling technologies, reclaiming precious metals like lithium, cobalt, and nickel from spent battery cells to reintroduce into the production cycle.
Another notable aspect is the movement towards biodegradable batteries. Research is ongoing into materials that are less harmful to the environment while still providing effective energy storage. These developments illustrate a gradual shift in the industry towards greener battery solutions, aligning with broader environmental objectives.
The importance of recycling and sustainable practices in battery technology cannot be overstated; they promise a future where electric mobility grows while minimizing its carbon footprint, proving essential for the industry as it continues to evolve.
Challenges and Limitations
Electric car batteries are at the heart of modern electric vehicles, driving their performance and efficiency. However, they aren't without their challenges. Understanding the limitations and downsides is crucial for anyone delving into this realm. These challenges not only affect the technology itself but also the broader adoption of electric vehicles on the roads today.
Safety Concerns
When dealing with electric car batteries, safety is a significant topic. Lithium-ion batteries, primarily used in electric vehicles, have been scrutinized for their risk of overheating and, in extreme cases, catching fire. The key factors influencing safety include:
- Thermal Runaway: This occurs when the battery cells overheat, possibly resulting in a fire or explosion. Factors such as poor battery design or defects during manufacturing can lead to this condition.
- Battery Management Systems: These systems are designed to monitor battery conditions, protecting against overcharging and overheating. However, the effectiveness of these systems varies between different manufacturers.
- Real-World Accidents: High-profile incidents involving electric vehicles can lead to public fear and skepticism about their safety. The media often focuses on battery failures, overshadowing the fact that gasoline engines also have safety concerns.
"While the technology has advanced significantly, safety standards must continually be reassessed to avoid incidents that could tarnish the reputation of electric vehicles."
Environmental Impact
The environmental footprint of electric car batteries is another focal point of concern. While electric vehicles are seen as cleaner alternatives to traditional combustion engines, the lifecycle of the batteries poses challenges. Key points to consider include:
- Raw Materials Extraction: The mining of lithium, cobalt, and nickel—the primary materials used in batteries—can lead to significant ecological damage. These mining processes often result in habitat destruction and water pollution.
- Manufacturing Footprint: Producing batteries is resource-intensive, generating substantial carbon emissions. The methods utilized can negate some of the environmental advantages of electric vehicles.
- Disposal and Recycling Issues: Battery disposal remains a growing concern as old batteries might leach harmful substances into the soil and water if not handled correctly. Although recycling programs are emerging, they are not yet widespread or efficient enough. Many recycled materials are still shipped overseas, raising questions about transparency and environmental ethics.
Future Trends in Electric Battery Development
The landscape of electric battery development is continuously evolving, spurred by the increasing demand for electric vehicles and the need for sustainable solutions. Recent advancements hold the potential to not only enhance battery performance but also to minimize environmental impact. Understanding these trends is vital for students, researchers, educators, and professionals who wish to stay ahead in the rapidly changing field of electric vehicle technology.
Competitive Innovations from Startups
A wave of startups is making its mark in the electric battery arena, often challenging established players with fresh ideas and disruptive technologies. These nimble firms can pivot quickly, focusing on niche areas that might be overlooked by larger corporations. For instance, companies like Sila Nanotechnologies are innovating by using silicon-based anodes which can increase energy density significantly compared to traditional lithium-ion batteries. This means electric vehicles could potentially travel much further on a single charge, which is a significant concern for users.
Moreover, startups such as QuantumScape are dabbling in solid-state battery technologies, which offer increased safety and longer lifespans. Solid-state batteries replace liquid electrolytes with solid materials, drastically lowering the risk of fires. As these technologies mature, we could see a paradigm shift in how electric vehicles are powered, allowing even greater performance without compromising safety.
Innovations in battery technology are often driven by a desire to solve foundational issues, like range anxiety and charging times, leading to a more compelling consumer offering.
Government Regulations and Incentives
Government support plays a pivotal role in steering the future of electric battery technology. Various regulations and incentives are being put in place worldwide, pushing companies toward greener practices and the development of more efficient batteries. For example, in the United States, the Inflation Reduction Act has allocated billions to clean energy projects, fostering advancements in battery research and production.
In Europe, stringent emissions regulations pressure automakers to expedite the transition towards electric vehicles. Countries like Norway aim to sell only zero-emission vehicles by 2025, creating an environment ripe for battery innovation. This results in attractive subsidies for consumers who purchase electric vehicles, which indirectly stimulates the demand for robust battery technologies.
With the introduction of these regulations, companies are incentivized to invest in research and development for next-generation battery technologies.
- Tax credits for battery production can reduce operational costs for manufacturers.
- Grants may be available for research initiatives focusing on recycling and sustainable practices.
- Governments may establish partnerships with companies to share knowledge and foster innovation through collaborative research ventures.
Harnessing the dual forces of startup innovation and regulatory support is crucial for the sustainable advancement of electric car batteries. As the spotlight continues to shine on this field, the interplay between technology and policy will shape the electric vehicle landscape for years to come.
End
The discussion surrounding electric car batteries isn't just technical jargon, it's a pivotal area that shapes the future of transportation and our approach to energy consumption. In this article, we've navigated through the complex landscape of electric car batteries, bringing to light essential aspects that contribute not only to vehicle performance but also to broader environmental considerations.
Summary of Key Points
As we delve into the summary, it's crucial to stress that:
- Physical Structure and Components: The battery's design plays a vital role in its effectiveness and energy efficiency. Each component, from electrolytes to battery management systems, is intricately tailored for optimal performance.
- Types of Batteries: The landscape is dominated by lithium-ion batteries, but alternative chemistries like nickel-metal hydride and prospects for solid-state technologies promise to revolutionize the sector.
- Performance Metrics: Energy density, charging capabilities, and lifespan are critical performance indicators that dictate how electric cars meet consumer expectations.
- Manufacturing and Sustainability: The methods by which these batteries are produced and the materials used impact overall sustainability and the environmental footprint of electric vehicles.
- Innovations and Trends: A forward-looking perspective reveals emerging technologies that aim to enhance battery life, improve efficiency, and adopt sustainable practices in battery recycling.
The Road Ahead for Electric Batteries
The future of electric car batteries poses intriguing questions and opportunities. Governments are ramping up regulations to ensure that the industry transitions smoothly towards greener technologies. As these policies unfold, startups and established companies alike are racing to innovate. Some are enhancing battery chemistry while others focus on improving manufacturing efficiency. Furthermore, the push for recycling programs is gaining momentum, reflecting a shift towards circular economies.
Ultimately, the horizon is rich with potential—these advancements not only have the power to redefine our energy reliance but also to create a more sustainable future. As we chart this course, staying informed is essential, and this article serves as a foundational guide to understanding the intricate world of electric car batteries.
"The only way to make sense out of change is to plunge into it, move with it, and join the dance."
— Alan Watts
With this detailed overview, we've embarked on an enlightening journey through the anatomy of electric car batteries, laying the groundwork for ongoing exploration and innovation in this dynamic field.