Understanding Electrolytic Processes: Basics and Uses
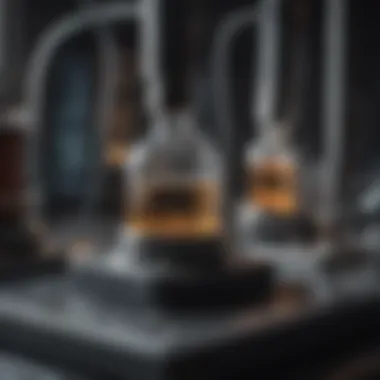
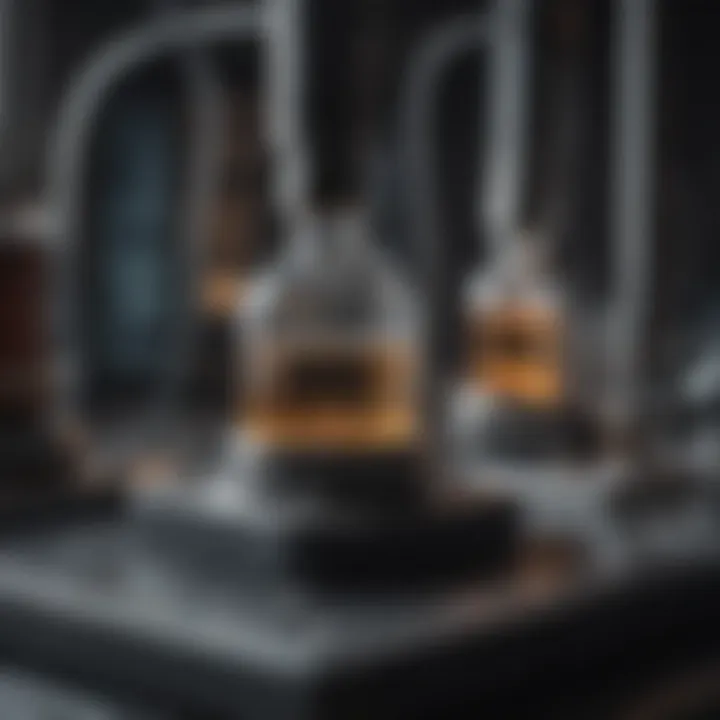
Intro
Electrolytic processes play a pivotal role in our understanding of numerous scientific principles, and their applications span across various sectors. From your car battery to industrial grade electroplating, the underlying mechanisms of electrolysis are intricately woven into the fabric of modern technology. This discourse intends to highlight the essentials of these processes and their significance in diverse fields like chemistry and materials science as well as environmental technology.
By dissecting the mechanisms that underpin electrolysis, we gain insight not only into how these processes work but also into why they matter. They're not just about splitting water into hydrogen and oxygen; they breathe life into advancements in clean energy technologies and waste management solutions. A comprehensive discussion will also address future trends that are shaping the landscape of electrolytic research and its practical implementations.
In exploring these aspects, it becomes clear that electrolytic processes are far more than mere curiosities. They offer exciting avenues for innovation and sustainability, making it crucial for scholars and industry experts to stay abreast of developments within this realm.
Preamble to Electrolytic Processes
Electrolytic processes are pivotal in understanding the intricate dynamics of chemical reactions that occur when an electric current is passed through a conducting solution. This section serves as a gateway into the realm of electrolysis, setting the stage for deeper exploration of its many facets in subsequent sections. The significance of electrolytic processes extends beyond mere theoretical concepts; they play a substantial role in various industrial applications, environmental remediation, and energy solutions.
In discussing electrolytic processes, we can highlight several key elements. Firstly, the ability to manipulate chemical reactions through electric currents opens doors for advancements in manufacturing and materials science. Industries rely on these processes for extracting metals and refining resources. Secondly, the environmental aspect cannot be overstated; electrolysis is a promising technique in water treatment and pollution reduction. Lastly, this area is an active field for research, indicating vast potential for future innovations and improvements.
Definition and Overview
At its core, electrolysis refers to the process of using direct electric current to drive a non-spontaneous chemical reaction. It is commonly used for the decomposition of compounds into their elements or simpler compounds. When a suitable electrolyte—usually a solution containing ions—is exposed to an electric current, chemical transformations occur at the electrodes, leading to the movement of ions and the production of various substances.
The basic setup includes an electrolytic cell, which contains two electrodes: an anode and a cathode. The anode is where oxidation occurs, and the cathode is the site of reduction. The flow of electricity through the electrolyte enables these reactions to take place, thus highlighting the fundamental relationship between electricity and chemical change.
Historical Context and Development
The historical roots of electrolytic processes trace back to the earliest experiments with electricity. In the late 18th century, scientists such as Alessandro Volta and Michael Faraday began to lay the groundwork for what we understand today as electrolysis. Faraday’s experiments in the early 19th century were particularly instrumental; he formulated principles that defined the relationship between electric charge and the mass of substances altered in electrochemical reactions.
Since its inception, electrolysis has seen numerous advancements. The initial applications were primarily focused on the production of metals like sodium and chlorine from their respective compounds. As technology evolved, the field expanded to include various applications ranging from electroplating in the manufacturing sector to more complex processes like water splitting, which has become central in hydrogen fuel research today. The journey of electrolytic processes is a testament to human ingenuity and our ongoing quest for efficient means to harness energy and transform materials.
Fundamentals of Electrolysis
Understanding the fundamentals of electrolysis is paramount for grasping its wide array of applications and implications in both industrial and environmental contexts. As we dive into this topic, we will uncover essential elements that influence the efficiency and effectiveness of electrolytic processes. Simply put, these basics serve as the foundation upon which more complex applications are built. Without a solid grasp of these principles, one might as well be sailing a ship in a storm without a compass.
Electrochemical Cell Configuration
An electrochemical cell is the nucleus of any electrolytic process—a structure designed to facilitate the necessary reactions for electrolysis to occur. Typically, this configuration includes two electrodes immersed in an electrolyte solution, permitting the flow of current and thereby enabling chemical transformations.
To put it plainly, picture two poles of a battery: one positive, often referred to as the anode, and the other negative, known as the cathode. Each serves distinct purposes during the electrolysis process. During operation, ions from the electrolyte move towards the respective electrodes, where they either gain or lose electrons, marking the foundation of electrochemical behavior. Without this dynamic interaction, the entire process would stall, akin to a car without wheels.
Key Components Involved
Electrolyte Solutions
Electrolyte solutions are critical for facilitating the movement of ions in electrolytic processes. They typically contain salts, acids, or bases, dissolved in water. One of the standout features of electrolyte solutions is their ability to conduct electricity when dissolved. This quality allows for optimal ion mobility, essential for efficient electrolysis.
In many instances, water serves as the solvent, providing a medium for ionic conduction. However, the type of electrolyte can significantly affect the overall efficiency and outcome of the process. For example, sodium chloride solutions can be popular due to their cost-effectiveness, yet other organic electrolytes might offer better conductivity under specific circumstances. It’s crucial to recognize that while many commonly available solutions exist, the choice of electrolyte can underline the success or failure of an electrolytic application.
Electrodes
Then come the electrodes themselves, the silent workhorses of the electrochemical cell. Generally crafted from conductive materials like platinum or graphite, electrodes play a vital role in the reactions that take place during electrolysis. Their key characteristic is their ability to either accept or donate electrons, dependent on the direction of the current flow.
Selecting the right electrode material is important; different materials can alter reaction kinetics. For instance, while platinum exhibits excellent conductivity, its cost makes it less feasible for larger-scale applications. On the flip side, while cheap and available, materials like copper may not provide the same level of performance and longevity. The nuances of choosing electrodes can be the difference between a successful project and one that fizzles out like a damp firework.
Power Supply
No electrolytic process would be complete without a robust power supply. It provides the energy necessary to drive the chemical reactions by maintaining the electric potential across the electrodes. Generally, the power supply must be adjustable, allowing fine-tuning based on the needs of the specific electrolysis method employed.
Key to consider here is the voltage and current specifications, which should align with the electrochemical process in use. A power supply that can provide variable power is advantageous since it facilitates different electrolysis methods as requirements change. If the power supply is inconsistent, it can disrupt the reactions happening at the electrodes, letting the whole operation drift off-course, much like a leaking boat on the water.
Theoretical Principles Governing Electrolysis
A thorough understanding of electrolysis would be incomplete without delving into the theoretical principles that govern these processes. Two primary laws define this domain—Faraday’s Laws of Electrolysis and electrode reactions.
Faraday’s Laws of Electrolysis
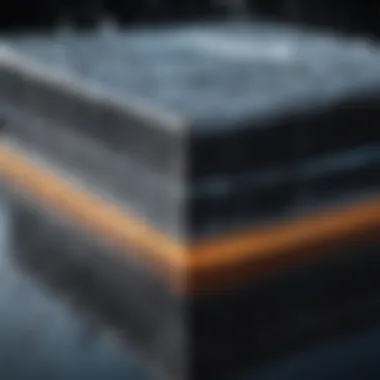
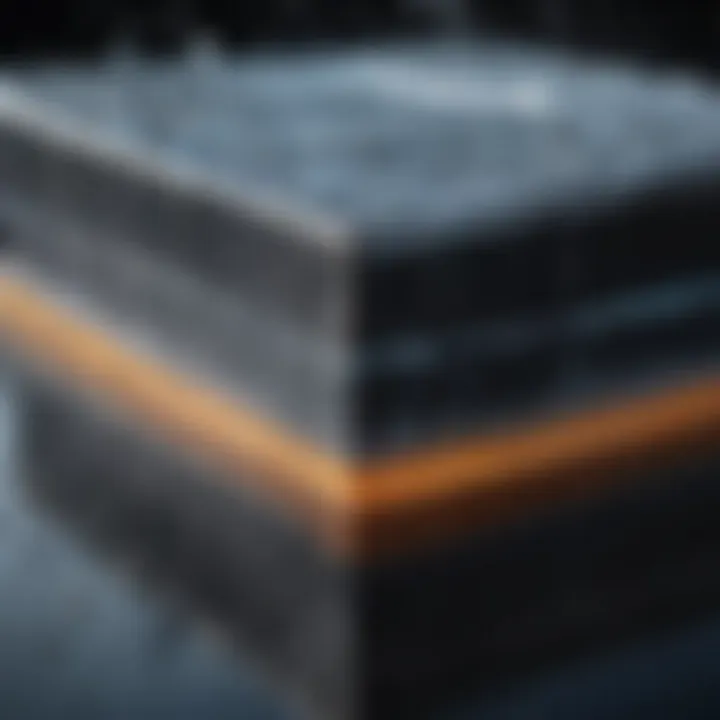
Faraday’s Laws form the backbone of electrolysis, articulating quantitative relationships between electric charge and the amount of substance transformed at an electrode. According to these laws, the mass of a substance altered at an electrode is directly proportional to the quantity of electricity passed through the electrolyte.
This principle has profound implications; it allows for precise calculations and predictions about the yield of products based on the supplied charge. When controlled properly, this leads to reliable and reproducible results, pivotal in applications ranging from water splitting to metal plating. Understanding these laws can empower researchers and engineers to optimize their approaches, enhancing efficiency beyond mere guesswork.
Electrode Reactions
Electrode reactions form the crux of what actually happens during electrolysis. At the anode, oxidation occurs, while reduction takes place at the cathode. Each reaction can be complex, influenced by various factors like temperature and concentration. What’s intriguing is that the specific reaction can determine the efficiency and yield of the desired products.
For instance, in the electrolysis of water, the goal is to generate hydrogen and oxygen gases. If conditions are mismanaged, it could lead to suboptimal yields or unintended products, bringing the whole operation to a standstill. This showcases the critical nature of understanding and controlling electrode reactions in achieving desired outcomes.
Types of Electrolytic Processes
Understanding the various types of electrolytic processes is crucial to grasp their importance and applications in multiple fields, including chemistry, materials science, and even environmental technology. Each process serves a unique purpose and brings its own advantages and challenges. This section aims to provide insights into these differences, further emphasizing why electrolytic techniques are favored in industrial and research applications.
Electrolytic Production of Metals
Aluminum Extraction
Aluminum extraction employs electrolysis predominantly through the Hall-Héroult process. This process involves dissolving aluminum oxide in molten cryolite. The primary aspect of aluminum extraction is its efficiency in separating aluminum from impurities. Not only is aluminum lightweight, but its electrical conductivity is also considerable, making it a favorite for various applications, including transportation and packaging.
One key characteristic of aluminum extraction is its ability to generate high-purity aluminum. This is particularly beneficial in industries that demand stringent material quality, such as aerospace. However, one should also consider the high energy requirements of this extraction method. The Hall-Héroult process is known for being energy-intensive, which can elevate production costs significantly. Despite this, the unique feature of aluminum's versatile use, coupled with its recyclability, makes aluminum extraction a leading process in the metallurgical sector.
Copper Refining
Copper refining, on the other hand, involves a different approach using electrolytic cells to purify impure copper. The specific aspect of this process centers around achieving high levels of purity, often exceeding 99.99%. This high purity is vital to meet the rigorous demands of industries that rely heavily on electrical conductivity, such as electronics and construction.
A notable characteristic of copper refining is its relatively lower energy consumption compared to aluminum extraction. That's a key reason why this method enjoys popularity. Additionally, copper can be recycled efficiently; hence, the refining process also offers an environmentally friendly option for creating high-quality metal. However, when considering long-term prospects, the fluctuation of copper prices on the market could pose economic challenges that should not be overlooked.
Electrolytic Electroplating
Principles of Plating
The principles of electroplating delve into the deposition of metal ions onto a surface through an electrolytic process. The importance of this topic lies in its application across various industries, especially in enhancing durability and aesthetics of products. This method allows metals to gain new characteristics, such as increased resistance to corrosion and enhanced visual appeal.
A prominent feature of electroplating is the precision with which layers can be applied, allowing for tailored finishes on various substrates. This specificity makes it an invaluable technique. However, issues such as environmental regulations concerning toxic metals (like cadmium or chromium) must be taken into account, affecting the choice of materials used in the process.
Industrial Applications
In industrial applications, electrolytic processes find their way into a multitude of uses, from automotive components to jewelry. The specific aspect of these applications includes enhancing the life span and performance of products. They play a significant role in meeting safety and durability standards in automotive parts and electronics.
One key characteristic is the increased market competitiveness for companies that use these processes effectively, due to the higher quality and more robust materials produced. A unique feature of industrial electrolytic processes is their adaptability to many different environments and needs, tailoring to sectors such as tool manufacturing, electronics production, and decorative applications. Despite the advantages, companies must remain vigilant against the cost implications of waste treatment and regulatory compliance related to the materials involved.
Water Splitting and Hydrogen Production
Water splitting stands as another vital electrolytic process with immense potential for sustainable energy production. The significance of this process lies in its ability to produce hydrogen fuel, which is increasingly viewed as a clean alternative energy source. This section will further explore the efficiency and mechanisms involved in the electrolytic splitting of water, including catalysts and energy requirements, offering readers insights into its revolutionary impacts on energy systems.
Applications of Electrolytic Processes
The realm of electrolytic processes extends far beyond mere academic interest. Their applications touch numerous sectors, serving as pivotal techniques that enable advancements in technology, manufacturing, and environmental management. This section delves into the varied applications of electrolysis, highlighting its significance in industries and the environment, thereby providing a holistic view of its multifaceted role.
Industrial Uses of Electrolysis
Chemical Manufacturing
Chemical manufacturing is one area where electrolytic processes really shine. By utilizing electrolysis, manufacturers can synthesize essential chemicals through efficient pathways. For instance, the production of chlorine and sodium hydroxide from brine demonstrates a straightforward yet effective electrochemical reaction whose implications are vast. These chemicals find usage in everything from pharmaceuticals to the production of plastics.
The key characteristic of this process is its ability to split complex compounds into useful simpler ones with precision. This tailored approach makes it a beneficial choice for industries aiming for both quality and output. Additionally, the unique feature of electrochemical processes is their relatively lower energy consumption compared to traditional thermal methods, positioning chemical manufacturing via electrolysis as a more sustainable option.
However, it’s not all smooth sailing. The initial setup costs tend to be on the higher end, which can deter smaller operations from adopting this method. Still, those who can navigate the upfront hurdles often find themselves at a competitive edge, benefitting from long-term savings and reduced environmental impact.
Metal Processing
The field of metal processing also harnesses the principles of electrolysis with remarkable effectiveness. This includes processes such as electrorefining and electrodeposition, which are capital in refining metals like copper and gold to high purities. By employing electrolysis, it facilitates the selective recovery of metals from ores and scrap materials. This benefits not only the economy through cost-effective production but also the environment by promoting recycling.
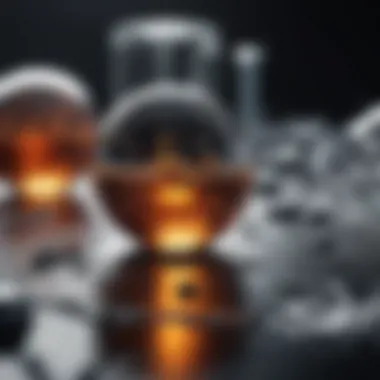
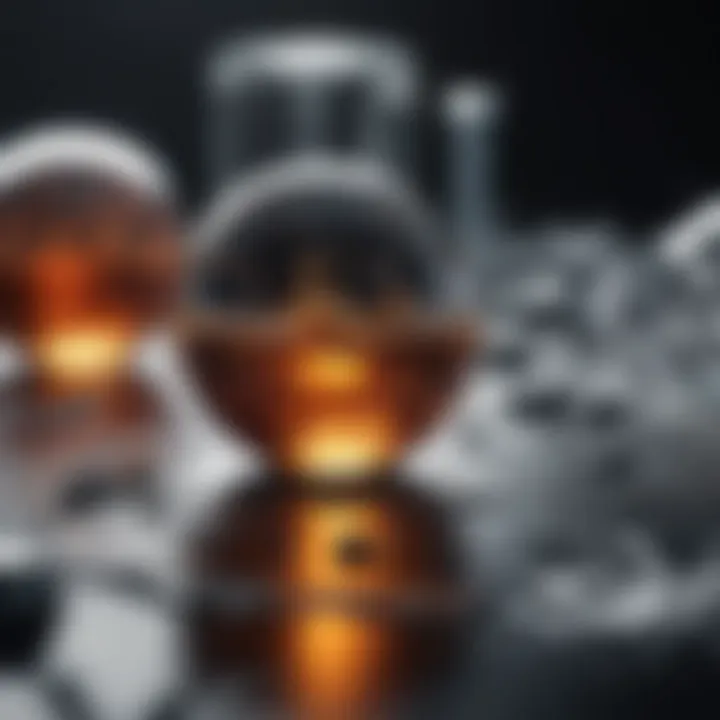
A major hallmark of metal processing through electrolysis is its ability to achieve refined metal quality, which often exceeds that of metals produced through conventional methods. The process suits large-scale operations and is widely favored in industries due to its scalability and efficiency.
On the downside, the challenge lies in the complexities of setting up and maintaining the systems, as well as managing toxic by-products. Although these challenges exist, the advantages of reduced impurities and material recovery make metal processing via electrolysis a wise choice for many industrial sectors.
Environmental Applications
Electroremediation
One of the key environmental applications of electrolytic processes is electroremediation. This technology utilizes electric currents to remediate contaminated soil or groundwater. Electrodes are strategically placed in contaminated sites, enabling the disintegration of pollutants into less harmful compounds.
Electroremediation stands out due to its targeted approach, which is less disruptive than traditional methods such as pumping and treating. It can be applied to a broad range of contaminants, making it a versatile choice for environmental cleanup. The beauty of this technique lies not only in its effectiveness but also in its capacity to minimize disturbance to surrounding ecosystems.
However, while electroremediation is resourceful, it comes with considerations related to energy usage and thorough site assessments prior to implementation. If not managed well, these aspects can lead to complications that negate the benefits.
Water Treatment
Water treatment is another critical area where electrolysis plays a vital role. Through processes such as water disinfection and desalinization, electrolytic methods can remove harmful pathogens and convert salty water into fresh drinking water. The importance of this application is magnified as global populations grow and clean water becomes increasingly scarce.
The key characteristic of electrolysis in water treatment is its potential for real-time treatment of contaminants, which is invaluable in emergencies or areas lacking infrastructure. Additionally, as a method, it can operate with minimal chemicals, enabling a more eco-friendly approach to water purification.
Yet, despite its clear advantages, the investment in electrolytic water treatment setups can be significant, alongside ongoing maintenance and the need for consistent power resources. Balancing these factors against the profound benefits of improved health outcomes and environmental sustainability is crucial.
Electrolysis in Energy Storage Technologies
Battery Technologies
Battery technologies have witnessed significant evolution thanks to advancements in electrolytic processes. From lithium-ion batteries to flow batteries, electrolysis integrates into the charging and discharging mechanisms, proving essential to energy storage and management. This has profound implications as society transitions towards renewable energy sources, making efficient and reliable storage solutions paramount.
One of the leading characteristics of battery technologies reliant on electrolysis is their ability to store energy effectively for later use, contributing to grid stability and renewable energy integration. Unlike typical electrochemical storage systems, batteries can offer efficient energy conversion rates while also providing a versatile range applicable in various sectors, including electric vehicles.
However, challenges remain in the performance efficiency of batteries, which can fluctuate under different operating conditions. Addressing these issues while striving for advancements in energy density and longevity is critical to the continued success of battery technologies.
Fuel Cells
Fuel cells are another remarkable application of electrolytic processes, turning chemical energy directly into electrical energy with exceptional efficiency. Utilizing hydrogen and oxygen, fuel cells generate electricity, water, and heat, marking a groundbreaking shift towards cleaner energy solutions.
One defining aspect of fuel cells is their minimal emissions, catering to environmental concerns while catering to energy demands. This makes them especially appealing in sectors like transportation, where they could replace traditional combustion engines. Their modular design offers flexibility, making them suitable for a wide range of applications, from portable electronics to power plants.
Nevertheless, the challenges in fuel cells lay in hydrogen production and storage. The infrastructure for hydrogen as a fuel is not nearly as established as that for fossil fuels, creating hurdles that must be jumped over. Despite these challenges, as technology advances and regulatory support grows, fuel cells are likely to hold significant promise for a sustainable energy future.
Innovations and Advances in Electrolysis
The realm of electrolytic processes has seen considerable innovation over the years. Understanding these advances is crucial as they set the stage for future developments in various applications including energy storage, environmental cleanup, and materials processing. The importance of exploring innovations and advances in electrolysis lies not just in the current usability but also in their potential to address pressing global challenges.
Research Trends in Electrolytic Processes
Recent research trends indicate an increasing interest in efficiency and sustainability within electrolytic processes. Scientists and engineers are exploring ways to reduce energy consumption and enhance the overall efficiency of electrolytic cells. This pivot towards sustainable practices is part of a broader effort to lessen environmental impact while meeting the demands of industrial and technological advancements. Researchers are also focused on optimizing the electrode materials, which play a pivotal role in determining the efficiency and rate of electrolysis. Collaborative efforts among academic and research institutions show promise in pushing the boundaries of what's currently achievable in this field.
Emerging Technologies
Nanomaterials
Nanomaterials have emerged as a significant aspect of recent advances in electrolysis. Their high surface area-to-volume ratio makes them particularly suitable for applications in electrochemical reactions. One of the key characteristics of nanomaterials is their ability to improve reaction kinetics, which translates to greater efficiency in electrolytic processes. For instance, using graphene-based materials can enhance conductivity and reduce overall energy input during electrolysis.
However, while they show much promise, the scalability and cost-effectiveness of sourcing nanomaterials still pose challenges. Practical applications must find a balance between performance and economic viability, particularly in large-scale manufacturing settings.
Advanced Electrolyte Solutions
Advanced electrolyte solutions are another promising avenue for innovation in electrolysis. By developing more efficient electrolytes, researchers can optimize the ionic conductivity crucial for effective electrolysis. A notable characteristic of advanced solutions is their ability to maintain stability under various operational conditions, offering enhanced control over the electrochemical reactions. Moreover, certain ionic liquids have emerged as a favorable option due to their wide electrochemical windows and low volatility.
Nevertheless, there are challenges associated with these solutions, notably their manufacturing costs and potential environmental impact. Selecting environmentally friendly options in creating advanced electrolyte solutions will be key in ensuring that progress is both effective and responsible.
"In pursuing innovation, balance is needed. Not all that glitters in technology is gold; sustainability matters as much as efficiency."
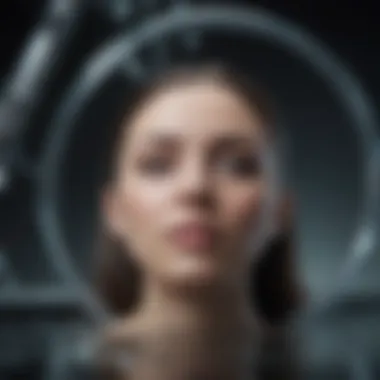
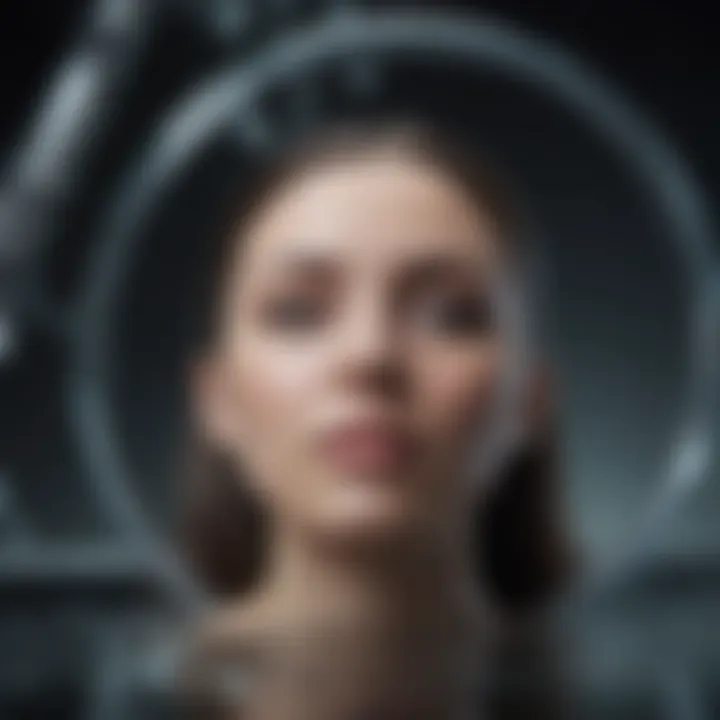
Ultimately, the path forward for electrolytic processes hinges on resolving these challenges while maximizing the unique benefits of emerging technologies. The dialogue between researchers and industry practitioners is vital for catalyzing advancements that meet contemporary needs and pave the way for a more sustainable future.
Challenges and Limitations of Electrolytic Methods
Electrolytic methods have carved out a significant space in modern technology, but they are far from flawless. Understanding their challenges and limitations is crucial for anyone venturing into this complex field. These challenges often dictate the pace of advancements in electrolysis technologies, influencing both economic outcomes and environmental sustainability. It's here that we need to peel back the layers to see how these limitations affect broader applications and how they may be addressed for future innovation.
Economic Viability
At the heart of the matter is the economic viability of electrolytic processes. These methods can often require substantial initial investments in both equipment and materials. For instance, setting up an electrolytic cell not only involves the purchase of electrodes and electrolyte solutions, but also the integrated systems managing energy input. When viewed through the lens of industrial applications, this initial capital outlay can be daunting, especially for small to medium enterprises. The high operational costs that accompany these processes can pose significant barriers. Moreover, the fluctuating prices of metals, electricity, and maintenance create a precarious situation for long-term planning.
Consider the aluminum production industry, where the Hall-Héroult process—a widely used electrolytic method—functions as a beacon of this dilemma. Even a minor increase in energy costs can dramatically affect profit margins. Consequently, organizations must weigh their options meticulously, balancing the projected benefits against the financial risks involved.
"When it comes to electrolytic methods, money talks louder than science at times."
Energy Efficiency
Energy efficiency is another roadblock in the electrolytic landscape. While advancements have been made, many electrolysis processes still suffer from low energy conversion rates. This means that a significant amount of the energy input doesn't translate into useful output, leading to high operational costs. For instance, in water splitting, the energy lost in heat can be quite substantial, reducing the overall efficiency of the process.
Efforts to improve these efficiency metrics are underway, but many of them rely on finding the right balance between performance and cost. The development of new electrode materials and improved cell designs is crucial. However, scientifically novel solutions can take time to materialize and face rigorous industrial testing before they are deemed viable. Sometimes, the benefits of higher efficiency come with a trade-off in complexity or cost.
Environmental Concerns
Then there’s the issue of environmental impact. Although electrolytic processes can contribute significantly to sustainability in sectors like hydrogen production, they are not devoid of ecological footprints. Waste products, whether chemical or thermal, can lead to soil and water contamination if not managed correctly. For example, during the electrolysis of brine for chlorine production, byproducts such as hypochlorite can pose environmental threats if released without adequate treatment.
Moreover, energy sources for electrolytic processes often rely heavily on fossil fuels, undermining the very purpose of seeking cleaner technologies. Transitioning these processes to utilize renewable energy sources is essential but also presents unique challenges of its own.
It’s apparent that while electrolytic processes offer a myriad of opportunities, they also come bundled with several challenges ranging from economic viability and energy efficiency to environmental concerns. Any movement forward will require a concerted effort from scientists, engineers, and policymakers to navigate these obstacles effectively.
Future Directions in Electrolytic Research
In the ever-evolving landscape of scientific inquiry, electrolytic research stands on the precipice of remarkable developments. The future of this field is not confined to its foundational principles but brims with potential, especially as it increasingly intersects with global challenges like sustainability and energy efficiency. A spotlight on future directions in electrolytic research illuminates not only how these processes can be adapted for newer applications but also how they can contribute to societal needs. Understanding these directions offers insights into addressing challenges that pervade industry sectors and environmental domains alike.
Potential for Sustainable Practices
The drive towards sustainable practices has never been more vital, and electrolytic methods are well-positioned to play a pivotal role. These processes, known for their efficiency in energy use and material conversion, are being reexamined through the lens of sustainability. Consider, for instance, the use of electrolysis in waste management. Employing electrolysis to break down pollutants transforms hazardous waste into harmless byproducts—an innovation that champions both health and environmental stewardship.
The potential of electrolytic processes also extends to the realm of sustainable materials. Research into biocompatible electrolytes leads to alternatives that minimize the environmental impact. For example, using organic compounds as electrolytes can pave the way for greener technologies, potentially reducing dependence on harmful substances and promoting a circular economy.
Moreover, as industries and communities adopt stricter regulations, the need for sustainable manufacturing processes will only grow. Electrolysis can contribute here, too, enabling cleaner production pathways that align with regulatory frameworks while satisfying consumer demand for environmentally friendly products. This shift not only adds value to the output but offers a much-needed rethinking of traditional practices that aren't as sustainable.
Integration with Renewable Energy Sources
The integration of electrolytic processes with renewable energy sources is a focal point for future research and technological advancement. As the world pivots to renewables, harnessing solar or wind energy to power electrolysis presents an alluring opportunity. Imagine using solar panels to transform water into hydrogen, a clean fuel, essentially becoming an energy storage solution. This concept could turn theoretical discussions into practical applications, effectively bridging the gap between energy production and storage.
In this light, integrating renewable energy with electrolysis not only bolsters energy security but also contributes to decarbonization efforts. An example could be coupling photovoltaic systems with hydrogen production; excess electricity generated during peak sunlight can drive electrolytic processes that create hydrogen, effectively storing energy until it is needed. This synergy mitigates the intermittency issues commonly associated with renewable energy sources.
"The combination of renewable energy technologies and electrolytic processes could redefine energy paradigms and create a more sustainable future."
Exploring new materials that enhance the efficiency of electrolytic cells also plays an integral role. Research is delving into catalyst development, aiming to lower energy input while maximizing output efficiency. Such advancements will make this dual approach not only feasible but financially strategic as well.
Finale
In wrapping up our exploration of electrolytic processes, it’s clear that understanding this field goes beyond simply knowing how to set up an electrochemical cell. The implications are vast, affecting a range of disciplines from material science to environmental technology. Recognizing the principles of electrolysis is not just academic; it is crucial for harnessing sustainable practices as industries pivot towards greener methods in production and resource management.
Summary of Key Points
To distill the essence of our discussion:
- Core Principles: Electrolysis hinges on the movement of ions within an electrolyte, driven by an external power source. Understanding Faraday's laws provides the foundation for predicting outcomes in electrolytic reactions.
- Diverse Applications: Industries rely on electrolytic processes for everything from aluminum extraction to electroplating. Each application demonstrates the versatility of these methods in real-world scenarios.
- Innovative Frontiers: Advances in materials like nanomaterials are shaping how we think about electrolytic processes. Research continues to explore more efficient ways to utilize energy and raw materials.
- Challenges Ahead: Economic viability and energy efficiency remain pressing concerns in the science of electrolysis. Addressing these issues is vital for making electrolytic processes sustainable and accessible.
- Future Directions: As the focus shifts to integrating renewable energy sources, the potential for electrolytic processes to contribute to a more sustainable future has never been more promising.
Final Thoughts on Future Implications
The future of electrolytic processes presents a mosaic of opportunities and challenges. From enhancing energy efficiency in battery technologies to deploying electrolytic methods for environmental remediation, the possibilities are expansive.
As industries continue to embrace sustainable practices, electrolytic innovations could play a pivotal role in reducing our carbon footprint. With growing interest in green technologies, researchers and practitioners alike must collaborate to push the boundaries of what is achievable through electrolysis.
"The only way to do great work is to love what you do." – Steve Jobs
In closing, the implications outlined within this article not only illuminate the significance of electrolytic processes but also emphasize the importance of ongoing research and innovation in this field. Every new discovery holds the potential to change the narrative surrounding manufacturing, energy consumption, and environmental stewardship. The next wave of breakthroughs could very well redefine our approach to chemistry and industry in fundamental ways.