Exploring 16s Gene Sequencing: Techniques and Applications
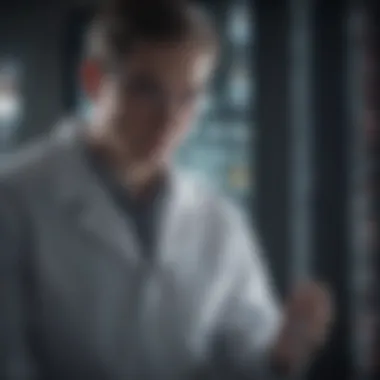
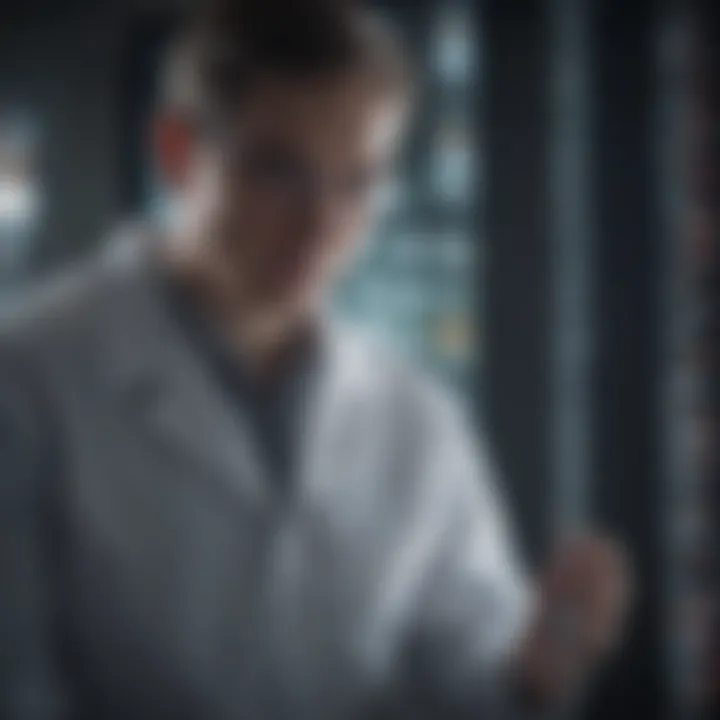
Intro
Understanding the intricacies of microbial life has been a cornerstone in advancing fields such as medicine, ecology, and biotechnology. Among the essential tools in molecular biology, 16s gene sequencing stands out. It serves as a linchpin for identifying and classifying microorganisms, offering a glimpse into the hidden biosphere that surrounds us. The significance of the 16s ribosomal RNA gene lies not just in its ability to pinpoint bacterial taxa, but also in how it facilitates insights into phylogenetic relationships, thus reshaping our comprehension of microbial diversity.
This exploration of 16s gene sequencing unfolds in three distinct yet interlinked layers. First, it provides a comprehensive grasp of the fundamental principles behind gene sequencing itself, emphasizing the role of the 16s rRNA gene. Following that, the article will delve into various methodologies employed in this sequencing technique, analyzing their respective pros and cons. Lastly, the implications of these methods will be scrutinized in several contexts, whether it be in microbiology lab settings, clinical diagnostics, or even environmental assessments. Each section is intended to reveal not just what is known, but also pave the way for future investigations and innovations.
As we embark on this journey into the world of microorganisms, let us first root our understanding in the Research Methodology, where we will elucidate the designs and approaches that underpin 16s sequencing.
Prelude to Gene Sequencing
Understanding gene sequencing is akin to deciphering the very code of life. It’s crucial to comprehend not only the basic principles but also how various techniques have emerged over time to analyze genetic material effectively. In this article, we will explore the intricate world of 16s gene sequencing, which is pivotal for identifying microorganisms, understanding their relationships, and studying their roles in diverse ecosystems.
With the rapid advancements in technology, gene sequencing has come a long way. The advent of high-throughput methods has made it feasible for scientists to sequence entire genomes in a fraction of the time it took a few decades ago. The significance of the 16s ribosomal RNA gene is at the forefront, as it's a universally conserved marker across bacteria and archaea, making it invaluable for microbial classification and phylogenetic studies.
The exploration of gene sequencing can lead to innovations across various sectors such as healthcare, environmental science, and agriculture. In medical diagnostics, for instance, it aids in the identification of pathogens, enabling tailored therapies and public health strategies. From a research perspective, understanding these sequences opens doors to new discoveries about microbial diversity and functionality in the planet’s ecosystems.
Understanding the Basics of Gene Sequencing
Gene sequencing involves determining the precise order of nucleotides within a DNA molecule. Each nucleotide consists of a sugar, a phosphate group, and a nitrogenous base, which ultimately dictates the genetic instructions for developing organisms. The basic step in sequencing is extracting DNA from an organism, followed by amplification, cloning, and finally, sequencing.
There are various methods available, each with its strengths and weaknesses. The simplest method employs chain-termination techniques, allowing scientists to read the sequence in small fragments. More advanced techniques, such as next-generation sequencing, can sequence millions of fragments simultaneously, providing a complete picture in drastically lesser time.
It's also important to highlight the biochemical significance of the 16s rRNA gene, primarily its structural properties that facilitate interactions with ribosomal proteins. Its conserved nature across species makes it an ideal target for probes when identifying unknown bacteria in a sample.
Historical Context of Sequencing Technologies
Gene sequencing has not always been as sophisticated as it is today. The field took its first steps back in the mid-1970s, thanks to the ingenuity of researchers like Frederick Sanger. Sanger's method, famously known as Sanger sequencing, laid the groundwork for many sequencing strategies we depend on today. This technique involved using chain-terminating inhibitors to produce DNA fragments of various lengths that could then be analyzed to determine the base sequences.
As challenges persisted, new technologies emerged. The 1990s saw the development of automated sequencers, which sped up the process significantly. The completion of the Human Genome Project in the early 2000s marked a turning point; it aimed to map the entire human genome and demonstrated the value of sequencing in scientific research.
Today, the landscape of gene sequencing has evolved into a competitive field, with the rise of next-generation sequencing (NGS) technologies that allow scientists to perform bulk sequencing and explore complex genetic variations. This drastic change not only enhanced the speed and efficiency of sequencing but also paved the way for wide-ranging applications in health, environmental sciences, and beyond.
Gene sequencing's past informs its present; understanding the history enhances the perspective on where this technology might lead us.
The 16s Ribosomal RNA Gene
The 16s ribosomal RNA gene plays a crucial role in gene sequencing, especially concerning our understanding of microbial life. This gene is relatively conserved among various organisms, making it an essential tool for comparing evolutionary relationships and understanding microbial diversity. It's like having a common language among different species; despite their differences, they all share this gene, allowing scientists to decipher the complex tapestry of life that exists, particularly within ecosystems that might often be overlooked.
Structure and Function of 16s rRNA
The structure of 16s rRNA is fascinating and complex. It consists of approximately 1500 nucleotides and has a 3D architecture that is vital for its function. The gene encodes a segment of the ribosome, the cellular machinery that facilitates protein synthesis. This ribosomal component is not only responsible for decoding the mRNA sequence but also helps maintain the integrity and positioning of the ribosomal subunits during translation.
The 16s rRNA molecule binds with ribosomal proteins, forming the small subunit of the ribosome which then interacts with the larger subunit to ensure accurate translation of the genetic code. Without this specific architecture and function, cells wouldn’t be able to produce the proteins necessary for life. Moreover, the structure of 16s rRNA features regions that are highly conserved, while others are variable; these variabilities are what help scientists distinguish between different bacterial species.
"The preservation of certain regions of the 16s rRNA gene through evolution highlights the fundamental role it plays in cellular processes."
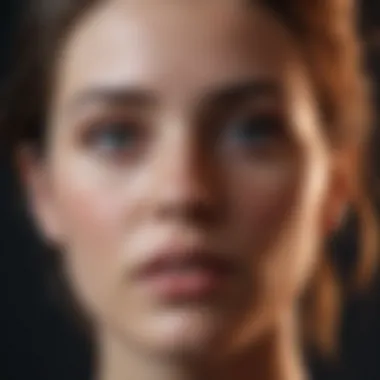
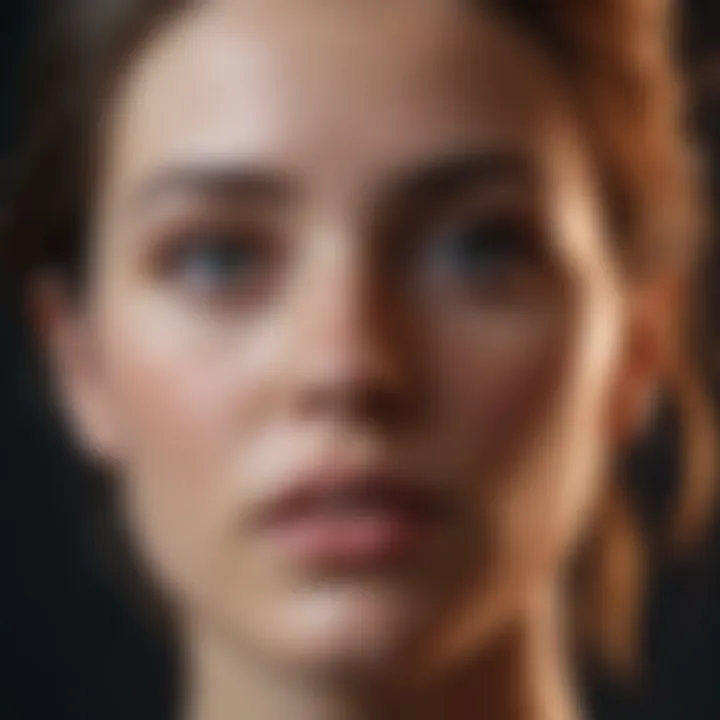
Evolutionary Significance of 16s rRNA
Tracing back through the evolutionary tree, the 16s ribosomal RNA gene has become a linchpin in phylogenetics. Its evolutionary significance lies in its ability to shed light on the connections between organisms, both past and present. The gene's slow rate of mutation helps establish a lineage of descent, giving researchers a clearer understanding of how species have diverged over time. Essentially, it serves as a genetic marker, with its variations pointing to evolutionary events and relationships.
Studying these sequences can clarify not only lineage but also the history of vital functions such as metabolic capabilities and pathogenic potential among bacteria. This insight is invaluable in fields like microbiology and medicine, where understanding these differences allows for better classifications of microbes, including those that might contribute to diseases in humans or plants.
Additionally, with advances in sequencing technologies, researchers are now delving into metagenomic explorations, providing further revelations about the ecological roles of these microbes and their interactions within ecosystems. As the field continues to grow, the evolutionary significance of 16s rRNA will undoubtedly led to unprecedented insights into the microbial world.
Methodologies in 16s Gene Sequencing
In the realm of molecular biology, especially when delving into the intricate world of microorganisms, the methodologies employed in 16s gene sequencing stand as the backbone of accurate identification and classification. Each technique presents unique features, advantages, and drawbacks, shaping the landscape of microbiological research. By understanding these methodologies, one can appreciate not just the science behind them but also their practical implications across various domains, from clinical diagnostics to environmental monitoring.
Polymerase Chain Reaction (PCR) Techniques
Polymerase Chain Reaction, or PCR, is often viewed as the workhorse of molecular biology. It's a method that amplifies specific segments of DNA, transforming minute amounts into quantities sufficient for sequencing. The technique is pivotal when working with the 16s rRNA gene because it allows scientists to target and duplicate this gene, even when present in trace amounts in a sample.
- Specificity: PCR utilizes primers that are complementary to sequences flank the target gene. This specificity increases the likelihood that the resultant amplifications are indeed from the 16s rRNA gene, minimizing contamination effects.
- Speed: Compared to other methods, PCR is noticeably quicker. Researchers can obtain multiples of the desired DNA in a few hours, a stark contrast to the longer timeline required by some other sequencing methods.
- Sensitivity: The ability to amplify DNA from limited sources—think of environmental samples riddled with diverse microbes—makes PCR essential for studies in microbiomes.
Despite its advantages, PCR isn't without challenges. Primer design can be tricky. If the primers do not bind correctly, the amplification may fail or produce misleading results.
Next-Generation Sequencing (NGS)
When it comes to modern methodologies, Next-Generation Sequencing has revolutionized the landscape. By allowing massive parallel sequencing, it enables an in-depth analysis of microbial communities. The benefits are profound:
- Throughput: NGS can process millions of sequences simultaneously, making it particularly advantageous for high-diversity samples often encountered in environmental studies.
- Coverage: It offers a comprehensive picture of the microbial community, capturing not just the dominant species but also rare organisms that could provide crucial insights.
- Cost-Effectiveness: The drop in sequencing costs over recent years has made NGS an appealing option for a broader range of studies, democratizing access to genetic information.
However, one should also consider the complexity involved in data analysis. The sheer volume of data produced can overwhelm researchers without adequate computational resources or bioinformatics skills.
Sanger Sequencing Method
Sanger sequencing, though older, still holds critical importance, especially for confirming results obtained from more complex methodologies. It is characterized by its simplicity and reliability. Here’s what makes it a noteworthy option:
- Accuracy: An established method that delivers trustworthy results. When the stakes are high, such as in clinical diagnostics, Sanger remains a reliable choice.
- Ease of Interpretation: The output is straightforward, often yielding clear sequences that can be easily compared against databases to identify organisms.
Nevertheless, Sanger sequencing falls short in terms of throughput and cost-effectiveness when scaling to large studies. It is not typically the first choice for exploratory phases of research but complements other techniques well.
Comparison of Sequencing Techniques
Now, let's step back and look at how these methodologies stack up against each other. Each technique has its own niche, but their comparative advantages can guide researchers in choosing the right tool for their specific needs:
| Method | Throughput | Cost | Accuracy | Complexity | | PCR | Moderate | Low | High | Low | | Next-Generation Sequencing | High | Variable | Variable | High | | Sanger Sequencing | Low | Moderate | Very High | Low |
In summary, understanding the strengths and limitations of PCR, NGS, and Sanger sequencing is crucial for effectively leveraging these methodologies in 16s gene sequencing. Each has its place in the lab, capable of delivering insights that advance our understanding of microbial diversity and function. Balancing efficiency with reliability ensures that researchers can yield meaningful results that might one day lead to groundbreaking discoveries.
Applications of 16s Gene Sequencing
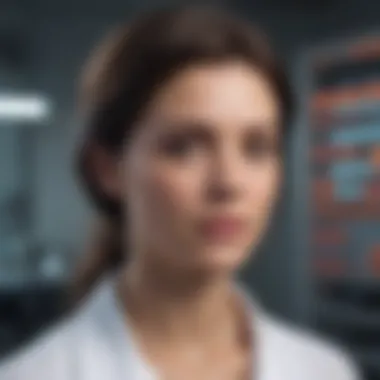
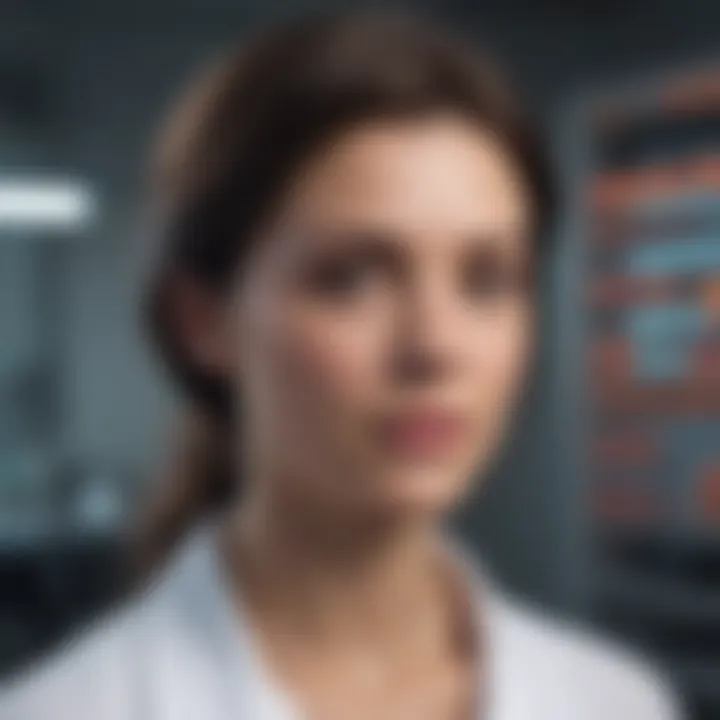
The significance of 16s gene sequencing cannot be overstated, particularly in today's world where microbial identities, interactions, and behaviors are gaining increased scrutiny in various fields. From uncovering the mysteries of the human microbiome to ensuring food safety, the diverse applications of this technique are both fascinating and essential. Below, we delve into several critical areas where 16s gene sequencing is playing a transformative role.
Microbiome Research
Microbiome research is at the forefront, shedding light on the complex communities of microorganisms that inhabit various environments, including the human body. By utilizing 16s gene sequencing, researchers can achieve a detailed profile of microbial diversity. This, in turn, helps in understanding how these microorganisms interact among themselves and with their host, providing insights into health and disease. For instance, imbalances in the microbiome—often referred to as dysbiosis—have been linked to conditions such as obesity, diabetes, and inflammatory bowel disease.
"Through 16s sequencing, we can tap into the intricate web of relationships that define our bodily functions and overall well-being."
Clinical Diagnostics and Pathogen Detection
In clinical settings, the application of 16s gene sequencing significantly enhances pathogen detection. Traditional methods, often time-consuming and reliant on culture techniques, can miss many pathogens or take too long for results. 16s sequencing provides a rapid and reliable means to identify bacterial pathogens directly from clinical specimens. Its ability to amplify and analyze small fragments of ribosomal RNA means clinicians can utilize it for diagnosing various infections with improved accuracy. This proactive approach not only speeds up patient management but also assists in monitoring antimicrobial resistance, thereby informing better treatment choices.
Environmental and Ecological Studies
The environment is another key area where 16s gene sequencing shines. It enables scientists to study biodiversity in habitats ranging from soils to oceans. This technology has been critical, especially regarding conservation efforts, by identifying keystone species within ecosystems and understanding their roles. For example, understanding microbial communities in agricultural soils can lead to improved crop management practices, promoting sustainability. Additionally, assessing microbial health in aquatic systems can serve as an indicator of environmental change or pollution, thus aiding in conservation strategies.
Food Safety and Quality Control
Ensuring food safety is a significant application of 16s gene sequencing, particularly in detecting microbial contaminants. Through precise identification of pathogens in food products, manufacturers can swiftly address potential outbreaks before they escalate. By continually monitoring food processing environments, businesses can maintain strict quality controls that safeguard consumer health. Also, as consumers become more health-conscious and curious about their food sources, 16s sequencing can aid in the verification of probiotic contents, ensuring accurate labeling and enhancing product integrity.
Pharmaceutical Development
In the realm of pharmaceuticals, 16s gene sequencing facilitates the discovery of new antibiotics and other therapeutic agents. As antibiotic resistance grows, there is an urgent need for new treatments. By analyzing microbial communities in various environments, researchers can identify potentially beneficial microorganisms that produce antibiotic compounds. Furthermore, the technique aids in understanding the mechanisms through which bacteria produce these substances, thus guiding drug development.
In summary, the applications of 16s gene sequencing are both broad and deep, addressing critical challenges and contributing to advancements across various disciplines. Its utility ranges from enhancing public health safety to fostering ecological sustainability and driving medical innovation. As continuous improvements in technology and methodologies unfold, the applications of this powerful tool will only expand, leading to deeper insights and new solutions in the years to come.
Challenges in 16s Gene Sequencing
Understanding the challenges present in 16s gene sequencing is vital for researchers and professionals aiming to leverage this technology effectively. With the prevalence of the 16s rRNA gene in microbial identification, it’s crucial to recognize the limitations and hurdles that can affect study outcomes. The challenges presented not only shape the landscape of research but can also limit the potential applications of sequencing techniques across various domains like clinical diagnostics and environmental monitoring.
Limitations of Current Technologies
Despite considerable advancements in sequencing technologies, several limitations persist in the context of 16s gene sequencing. For instance, traditional methods often lack the resolution needed to differentiate closely related species. While the 16s gene serves as a solid foundation for taxonomy, it may fall short when resolving organisms with high sequence similarity.
- Depth of Coverage: Current sequencing platforms sometimes can't ensure enough coverage of the target regions, potentially leading to an underrepresentation of certain species in complex samples.
- PCR Biases: Polymerase chain reaction techniques used prior to sequencing can introduce biases, amplifying certain sequences over others, creating a skewed representation of the microbial community.
- Cost Implications: Funding constraints can limit access to cutting-edge sequencing technology. Lower-cost options may sacrifice data quality, complicating interpretation.
These technical limitations serve as a reminder that while gene sequencing holds promise, a critical eye must be kept on the methodologies employed.
Data Analysis and Interpretation Issues
Data generated from 16s gene sequencing is not inherently easy to interpret, raising significant issues in the field. As sequencing technology becomes more sophisticated, so too does the complexity of the datasets produced.
- Bioinformatics Challenges: Analyzing large volumes of data demands robust computational tools and expertise. Improper data handling can lead to erroneous conclusions.
- Taxonomic Resolution: Classifying sequences is often muddled by variations within the 16s rRNA gene, resulting in unclear taxonomic assignments that may misrepresent microbial diversity.
- Standard Operating Procedures: There is often a lack of consensus on analysis pipelines, which can yield disparate results depending on the methods employed.
A clear understanding of these data interpretation issues is necessary for producing reliable and reproducible findings in microbial research.
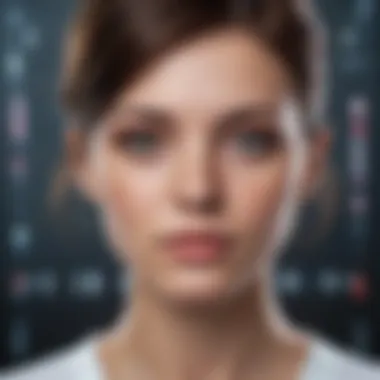
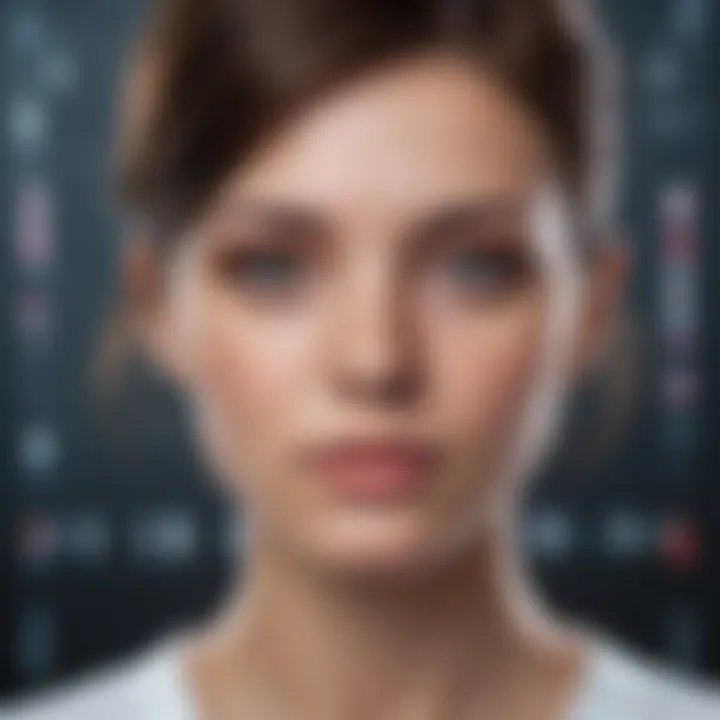
Standardization and Reproducibility Concerns
Standardization in 16s gene sequencing is critical for ensuring that findings are reproducible across various studies. However, the variability in protocols can lead to significant discrepancies.
- Protocol Variability: Differences in sample collection, DNA extraction, and sequencing platforms can introduce variance that complicates data comparison.
- Measurement Metrics: Establishing consistent metrics for characterizing microbial communities is a challenge, as various studies may report their results using different criteria.
- Inter-laboratory Comparisons: Lack of standard guidelines often leads to issues in reproducibility between different laboratories, making collaborative efforts and meta-analyses difficult.
Recognizing these challenges is essential for researchers aiming to further develop methodologies that capitalize on the power of 16s gene sequencing while minimizing potential pitfalls.
Future Directions in 16s Gene Sequencing
As the landscape of molecular biology rapidly evolves, the future of 16s gene sequencing is laden with potential. Analyzing microbial communities in different environments is becoming increasingly crucial as we acknowledge the impact of microorganisms on ecosystems, human health, and industry. This section delves into the emerging opportunities, advanced techniques, and the ethical implications surrounding the utilization of 16s gene sequencing.
Emerging Technologies and Innovations
Recent advances in technology are shaping the next chapter for 16s gene sequencing. The advent of long-read sequencing technologies, such as the Oxford Nanopore and Pacific Biosciences systems, provides a unique vantage point to uncover detailed microbial genomes. This cuts down the complexity often seen with traditional short-read sequencing methods. Coupled with these innovations, there’s increasing interest in combining 16s rRNA gene sequencing with metagenomics. This combination enhances the ability to identify not only the type of bacteria present but also their functional potential. The integration of artificial intelligence and machine learning is also gaining traction. Algorithms trained on extensive sequencing datasets can predict microbial interactions or even identify novel species based on their genetic sequences.
Integration with Other Omics Technologies
The interdisciplinary approach in science has never been more vital. Integration of 16s gene sequencing with other omics technologies, such as metabolomics and proteomics, is a pivotal future direction. For example, a comprehensive understanding of the microbiome could greatly improve if we also analyze the metabolites produced by microorganisms within a specific environment. This multi-omics approach allows scientists to connect genotype with phenotype, offering insights into how the microbiome affects health, disease, and environmental changes. Imagine understanding how a diet might influence gut microbiota through protein and metabolite analysis alongside gene sequencing; this could revolutionize dietary recommendations and health strategies.
Potential Ethical Considerations
As with any advancement in genetics, ethical concerns loom large. The expansion of 16s gene sequencing capabilities raises questions regarding privacy and data use, especially in clinical settings. Who owns the genetic information gained through sequencing? Furthermore, there is a risk of misinterpreting data leading to incorrect conclusions about microbial presence related to health implications. Another concern involves the unintended consequences of microbial data manipulation in agricultural or environmental contexts. Altering the microbiome in a controlled manner could have repercussions in the ecosystem which might not be easy to predict.
In light of these concerns, it’s imperative to establish robust ethical frameworks that address consent, ownership, and potential misuse of genetic data. This will require collaboration among scientists, ethicists, and policymakers to navigate the complexities surrounding biotechnological advancements responsibly.
"The future of science is not just about discovery; it's about communication, collaboration, and ethical responsibility."
The End
The conclusion of this article encapsulates the pivotal role of 16s gene sequencing in the broader context of molecular biology and microbial studies. This technique has not only revolutionized microbial identification but also serves as a stepping stone for a myriad of applications across various fields. By providing a reliable means to classify and understand microorganisms, 16s sequencing has carved a niche that underscores the complexities of life at the microscopic level.
In light of the discussions covered, the importance of 16s gene sequencing lies in its multifaceted benefits:
- Comprehensive Microbial Profiling: It offers a way to explore the microbial diversity that exists, which is crucial for applications in health, agriculture, and environmental science.
- Technological Adaptability: As methodologies continue to evolve, the adaptability of 16s sequencing enables researchers to keep pace with innovations in genomic studies.
- Data-Driven Insights: The examination of sequencing data fosters a deeper understanding of microbial interactions, evolution, and ecology.
The considerations surrounding this technique also demand attention. While 16s sequencing has its advantages, acknowledging its limitations is essential for accurate interpretation as highlighted in earlier sections. The ongoing refinement of analytical methods, data interpretation skills, and technological advancements will shape the future landscape of this field. Understanding these dynamics will be paramount for researchers aiming to leverage 16s gene sequencing more effectively.
This summary leads us seamlessly into the next aspect of our conclusion with key insights that can be drawn from the article's exploration of 16s gene sequencing.
Summary of Key Insights
- Fundamental Understanding: The 16s ribosomal RNA gene is a cornerstone for microbial classification owing to its presence in all bacteria and its conservational elements.
- Technological Evolution: The various sequencing methodologies like PCR, NGS, and Sanger methods present unique strengths and weaknesses that researchers should weigh depending on their objectives.
- Diverse Applications: From health diagnostics to food safety, the implications of 16s gene sequencing span multiple domains, making it an integral tool in both research and industry.
- Addressing Challenges: Current limitations regarding technology, data analysis, and standardization emphasize the need for continued innovations and collaborative efforts within the scientific community.
These insights underscore the profound impact of 16s sequencing on scientific research and technological advancements.
Implications for Scientific Research
The implications of 16s gene sequencing for scientific research are vast. As a foundational tool, it enables scientists to
- Unravel Taxonomy: Gain insights into the complex relationships and taxonomy of microorganisms, facilitating studies in phylogenetics and evolution.
- Enhance Medical Diagnostics: Improve clinical diagnostics, leading to earlier pathogen detection, personalized treatments, and better public health strategies.
- Drive Environmental Initiatives: Inform environmental sustainability efforts by identifying microbial communities in various ecosystems, aiding conservation strategies.
- Support Cross-Disciplinary Work: Engage researchers from multiple disciplines, including microbiology, ecology, medicine, and bioinformatics, fostering collaborative investigations and discoveries.
As we move forward, the role of 16s gene sequencing will likely become even more central to understanding and manipulating the microbial world. Future research, bolstered by innovations and integrative approaches, promises to deepen our understanding of life forms that thrive beyond human sight.