Exploring Direct CO2 Capture Technologies and Impacts
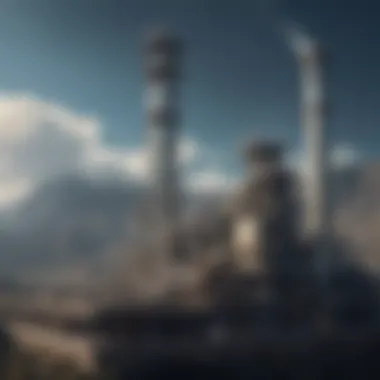
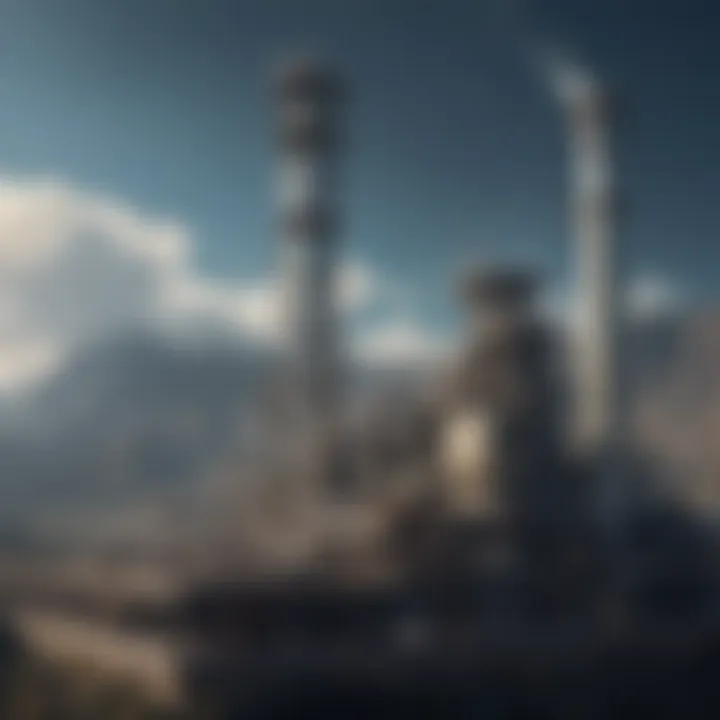
Intro
In recent years, the specter of climate change has pushed the boundaries of scientific inquiry towards innovative solutions. Among these solutions, direct CO2 capture technologies have emerged as a promising frontier in our battle against rising greenhouse gas emissions. This exploration is not merely academic; it addresses pressing global needs and serves as a vital component of strategies aimed at achieving carbon neutrality.
Direct CO2 capture involves an array of methods designed to extract carbon dioxide directly from the ambient air or from industrial processes. Many scientists believe this could play a significant role in offsetting emissions from sectors that are hard to decarbonize. As such, understanding how these technologies function, their effectiveness, and the obstacles they face is crucial for a sustainable future.
This article will peel back the layers of CO2 capture mechanisms, scrutinize their effectiveness, and highlight the necessary regulatory frameworks that could bolster their development. It aims to aid students, researchers, educators, and professionals alike in comprehending not only the operational aspects of these technologies but also their broader implications for environmental practices around the globe.
With the world pivoting toward greener alternatives, the importance of direct CO2 capture cannot be overstated. This article will detail the intricate web of methodologies, address the current state of research, and ascertain the potential impacts these innovations hold for climate change mitigation.
Let’s embark on this journey, navigating the realms of direct CO2 capture and exploring its vital part in our future.
Understanding Direct CO2 Capture
Direct CO2 capture has emerged as a pivotal technological approach in combating climate change. With carbon emissions soaring due to industrial activities, it is increasingly recognized that innovating durable solutions is vital. This section aims to shed light on the essence of direct CO2 capture, exploring what it is, how it works, and why it holds significant weight in today's climate strategy.
Defining Direct CO2 Capture
Direct CO2 capture refers to a series of innovative processes designed to extract carbon dioxide (CO2) directly from the atmosphere. Unlike traditional methods, which rely on capturing emissions from specific sources like power plants, these techniques seek to address ambient CO2 levels in the atmosphere. The captured carbon can either be stored in geological formations or utilized in various industrial applications.
Essentially, this process operates on one central idea: remove CO2 from the air to help mitigate its harmful effects on climate. Think of it as putting a vacuum into the atmosphere, sucking out unwanted pollutants and aiming for a cleaner planet.
One of the most striking aspects of direct capture is its adaptability. The technologies range from adsorption, where CO2 adheres to solid materials, to absorption, where it is dissolved in liquids. These methods often utilize chemical reactions to bind the CO2, which can then be segregated and stored or repurposed. This flexibility offers a framework that can be tailored to specific environments and needs.
Importance in Climate Strategy
The role of direct CO2 capture in climate strategy is nothing short of crucial. As countries set ambitious targets for reducing greenhouse gases, capturing carbon directly from the air provides a supplementary mechanism to traditional emission reductions. It can actively reverse past emissions and play a vital role in achieving net-zero goals.
"The capacity of direct CO2 capture technologies to mitigate atmospheric CO2 levels is a game changer in our fight against global warming."
In practical terms, integrating direct capture into current climate strategies can lead to numerous benefits, such as:
- Enhanced Carbon Management: By providing a method of actively removing CO2, this technology allows for more comprehensive management of carbon emissions.
- Support for Renewable Energy: Coupling the technology with renewable energy sources can lead to an even lower carbon footprint, making energy production cleaner.
- Economic Opportunities: Innovations in CO2 capture can spearhead new industries and job creation, thus bolstering the economy while addressing environmental issues.
Furthermore, public and private investments in this field can amplify research efforts, making the technologies more efficient, cheaper, and widely accessible.
In summary, understanding direct CO2 capture is essential to grasp the broader climate change narrative. It not only provides practical solutions but also complements policy efforts aimed at a carbon-neutral future.
The Scientific Foundations of CO2 Capture
Understanding the scientific principles behind direct CO2 capture is not just a technical requirement but a necessity for tackling climate change effectively. These principles help clarify how CO2 is captured and the potential efficiencies and limitations embedded within the various methodologies. This scientific underbelly is crucial for informing policy and investment decisions, enabling stakeholders to aim accurately for carbon neutrality goals. By comprehending the underlying science, researchers and practitioners can innovate and optimize capture technologies further, adding to the collective knowledge base in this vital field.
Chemical Reactions Involved
The chemical reactions involved in CO2 capture are fundamental to the functionality of various capture techniques. When it comes to direct CO2 capture, two primary processes are notable: adsorption and absorption. In adsorption, CO2 molecules adhere to the surface of a solid material, while in absorption, CO2 is dissolved in a liquid solvent.
To illustrate:
- Adsorption often utilizes materials like zeolites or activated carbon. In these cases, CO2 molecules cling to the surface due to physical or chemical forces. The efficacy of this process largely hinges on the surface area and porosity of the material. The larger the surface area, the more CO2 particles can attach.
- Absorption, on the other hand, typically employs aqueous amine solutions or similar solvents. Through a chemical reaction, CO2 reacts with the solvent to form carbamates, enabling the gas to be trapped in a liquid form, which can be more easily managed and segregated.
Understanding these chemical foundations allows for better optimization of materials and solvents. It also aids in developing hybrids of capture technologies, combining these fundamental reactions into more effective systems.
Thermodynamics and Kinetics
The second scientific pillar relates to thermodynamics and kinetics. These concepts govern how effectively CO2 can be captured, processed, and eventually released from capture systems.
- Thermodynamics plays a role in determining the feasibility of capture reactions. The enthalpy and Gibbs free energy changes can inform whether a reaction is spontaneous under given conditions. For instance, capturing CO2 at higher temperatures might require additional energy input, making the process less attractive.
- Kinetics, on the other hand, looks at the rate of the reactions. How quickly can CO2 be removed from the atmosphere and processed? Often, kinetics can be a limiting factor in large-scale applications. If a reaction is thermodynamically favorable but too slow, it can bottleneck the entire capture process.
Both the thermodynamic principles, as well as the kinetic constraints, must be taken into account during the design of CO2 capture systems. Efficient designs optimize for both, ensuring that the energy input is minimized while maximizing the speed of the process.
"Mastery of the intricate dance of thermodynamics and kinetics can transform the field of direct CO2 capture, turning theoretical possibilities into real-world solutions."
In summary, the scientific foundations of CO2 capture are pivotal for the advancement of this technology. By diving deep into the chemical reactions and the principles of thermodynamics and kinetics, we equip ourselves with the knowledge necessary to innovate new techniques and refine existing ones, thus making significant strides toward combating climate change.
Primary Methods of Direct CO2 Capture
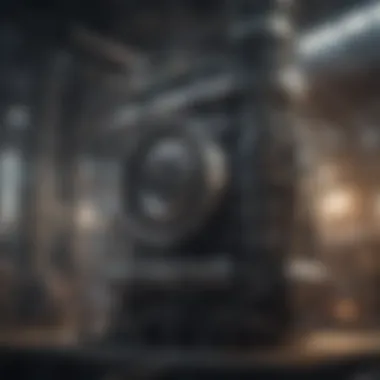
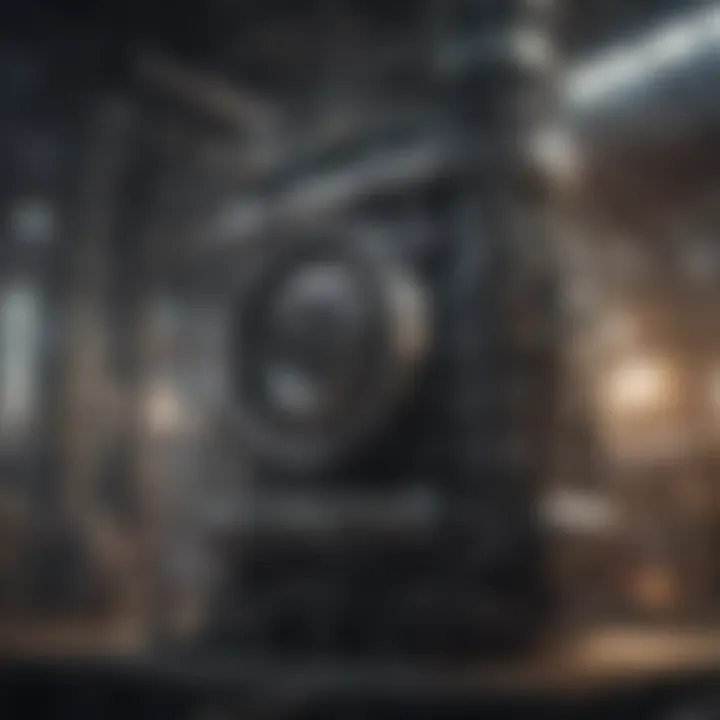
The exploration of direct CO2 capture technologies encompasses various methods, each with distinct advantages and challenges. Understanding these primary methods is crucial as they directly contribute to the broader goal of reducing atmospheric carbon dioxide levels, combating climate change, and working towards global carbon neutrality. The methods discussed below illuminate how diverse approaches can be tailored to specific contexts, allowing for effective implementation in various industrial applications.
Adsorption Techniques
Adsorption is a process where molecules from a gas phase adhere to the surface of a solid material. In the case of CO2 capture, adsorbents such as activated carbon or zeolites are employed to trap carbon dioxide. This method is advantageous because it can operate under ambient conditions, meaning less energy is required compared to some other techniques.
One significant benefit of adsorption techniques is their selective nature. Some adsorbents can differentiate between CO2 and other gases, enabling streamlined capture from complex mixtures like flue gas. Furthermore, these materials can undergo cycling, allowing for the regeneration of the adsorbent, thus making the process more economically viable.
However, challenges exist, particularly regarding the capacity of the adsorbents and the heat required for regeneration. As research progresses, the development of new materials promises to enhance the performance of adsorption for CO2 capture.
Absorption Processes
Absorption, unlike adsorption, involves the dissolution of carbon dioxide into a liquid solvent. Common solvents for CO2 absorption include aqueous solutions of amines, which chemically react with CO2 to form a compound. This method is widely used in industries, particularly where high concentrations of CO2 are present, like natural gas processing or power plants.
The primary advantage of absorption processes lies in their ability to capture large volumes of CO2 efficiently. The technology is mature, meaning it has been implemented at high capacities, with operational data available to optimize performance. However, absorbing CO2 also comes with its own set of hurdles, including the need for significant energy input to regenerate the solvent. Innovations in solvent development and process design continue to address these issues, enhancing overall efficiency and reducing costs.
Membrane Separation Methods
Membrane separation is an emerging technology that leverages selective permeability to separate CO2 from other gases. Typically, polymeric membranes are utilized, allowing smaller molecules like CO2 to pass through while retaining larger molecules. This method is appealing due to its low energy consumption compared to traditional separation techniques.
Membrane technology integrates smoothly into existing infrastructures, providing an efficient retrofit option for industries seeking to reduce their carbon emissions. Nevertheless, the scalability of such systems raises questions. The long-term durability of membranes under operational stress and their cost efficiency in large applications are areas of ongoing investigation. Researchers are hopeful that advancements will soon resolve these scalability issues, making membrane separation a reliable choice for CO2 capture.
Cryogenic Techniques
Cryogenic CO2 capture involves cooling gas streams to very low temperatures, where CO2 can be liquefied and separated from other gases. This method has the unique advantage of enabling the capture of extremely pure CO2, which is particularly valuable for processes where high purity is essential, such as in beverage carbonation or enhanced oil recovery.
The primary benefits here include high capture rates and the possibility of recycling the captured gas. However, the energy requirements for cooling and maintaining cryogenic conditions can be significant. Just like with other methodologies, further innovations are critical to improve efficiency and lower operational costs.
As industries dive deeper into CO2 capture methodologies, the challenge remains—how to make these processes more economically feasible while maintaining their effectiveness.
Comparative Analysis of Capture Technologies
Comparative analysis of capture technologies plays a pivotal role in understanding the diverse approaches available for direct CO2 capture. By examining various technologies, one can pinpoint their strengths and weaknesses, leading to informed decisions on which methods may be most effective for specific applications. This analysis is not just an academic exercise; it has far-reaching implications for policy-making, investment, and implementation strategies aimed at reducing atmospheric CO2 levels.
Each capture method—be it adsorption, absorption, membrane separation, or cryogenic techniques—comes with its unique set of efficiency metrics, cost implications, and scalability issues. Understanding these factors can help stakeholders assess the potential of these technologies within their respective contexts.
Efficiency Metrics
Efficiency metrics are essential for evaluating the performance of CO2 capture technologies. These measures typically assess the amount of CO2 captured relative to the energy consumed and the volume of air processed. The calculation isn't always straightforward, as it can depend on several variables such as:
- Energy consumption: This refers to the energy input required to capture CO2. Renewable energy sources can significantly enhance efficiency by lowering the carbon footprint of the capture process itself.
- Purity of captured CO2: The quality of the captured CO2 can affect its later usage, particularly if it is intended for resale or for industrial applications. A higher purity level leads to better outcome in many processes.
- Capture rate: The speed at which CO2 is captured often dictates how scalable a technology is. Faster processes can translate to economies of scale, making them more viable for larger operations.
The evaluation of these metrics often requires a balance. For instance, a method that captures CO2 at a rapid rate may need higher energy inputs, negating some of its advantages. Thus, touching on efficiency leads to more complex questions about optimality and applicability in real-world scenarios.
Cost Considerations
Cost is a crucial factor that influences the adoption of any technological solution. In the realm of CO2 capture, it involves both initial investment and ongoing operational costs. Here's a breakdown of key considerations:
- Capital Expenditure: The initial costs of setting up the infrastructure can be significant. Technologies like cryogenic capture may require more substantial investment compared to simpler adsorption methods.
- Operating Costs: Long-term expenses such as maintenance, energy, and manpower need to be factored in. Technologies that appear cheaper up front may incur higher operational costs down the line.
- Market Viability: The economic landscape is shifting, and CO2 can now be seen as a commodity thanks to advances in carbon capture utilization. Therefore, cost-effectiveness can also be shaped by market demand and regulatory incentives.
A technology that strikes the right balance between capital expenditure and operational viability is likely to gain traction, especially in environments that prioritize cost-efficiency.
Scalability Issues
The scalability of capture technologies addresses how well a particular method can adapt to growing demand or larger applications without compromising effectiveness. A few critical aspects to consider include:
- Infrastructure Requirements: Some methods may need extensive refitting of existing facilities, making them less appealing for quick adoption.
- Technological Maturity: Emerging technologies may offer promising results; however, if they haven’t been demonstrated at scale yet, stakeholders might hesitate about committing resources.
- Regulatory Frameworks: Various regulations may support or hinder scalability. Clear guidelines can motivate investment in innovative technologies, while ambiguous regulations can stall progress.
In summary, the comparative analysis of capture technologies highlights the myriad factors influencing their effectiveness in different contexts. By assessing efficiency metrics, understanding cost implications, and considering scalability, stakeholders can make informed choices that advance their environmental goals and contribute to the broader strategy of mitigating climate change.
Challenges in Direct CO2 Capture
The journey towards effective direct CO2 capture is certainly not a walk in the park. Numerous hurdles span technological, economic, and social realms, each entwined with its own complexities. Understanding these challenges is crucial, as they hold the keys to improving capture technologies and ensuring their wider acceptance. Identifying and addressing these barriers unlocks the potential of CO2 capture in the battle against climate change, making this section vital in comprehending the landscape of direct CO2 capture technologies.
Technological Barriers
In the realm of direct CO2 capture, technology often feels like a double-edged sword. Many promising methods exist, but not all are ready for prime time. The effectiveness of these technologies hinges on several factors, including:
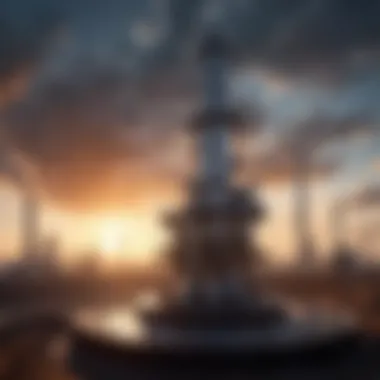
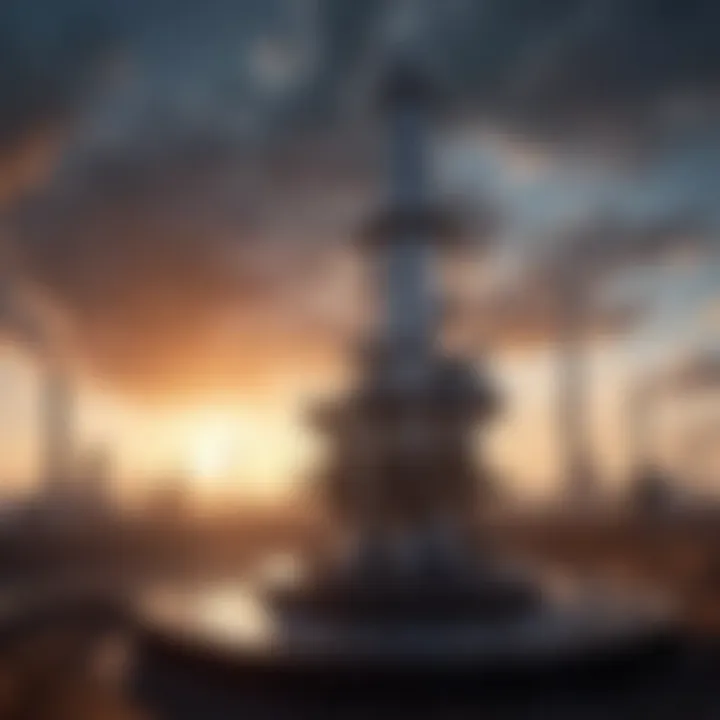
- Efficiency in capturing CO2 without excessive energy consumption.
- Material Durability that can withstand long-term exposure to harsh operational conditions.
- Process Integration with existing industrial structures.
Take, for instance, the absorption processes which involve complex chemical reactions. While capable of achieving high capture rates, they often demand intensive energy input, raising questions about their feasibility for large-scale operations. This leads to a pressing need for the development of novel materials and methods that can perform consistently while minimizing energy demands.
Economic Limitations
When it comes to economics, it’s a tough slog. Direct CO2 capture systems don't come cheap. The costs associated with setup, operation, and maintenance can put a significant strain on budgets—especially for companies trying to balance profitability with sustainability. Frequently highlighted economic concerns include:
- Capital Costs: The initial investment for machinery and technology can be prohibitive.
- Operational Costs: Ongoing expenses for energy, materials, and skilled labor must be factored in.
- Market Viability: Without substantial incentives or financial backing, many projects may struggle to remain viable over time.
For example, amine-based systems, which show promise in capturing CO2 effectively, require significant capital outlays that can deter investment. The economic realities must align with climate goals—a tall order for many.
Public Perception and Acceptance
Lastly, we can't ignore the public's role in the story of direct CO2 capture. Just because technology might be sound doesn’t mean the people will embrace it. Factors influencing public perception include:
- Understanding: Awareness of what direct CO2 capture entails and its benefits to the environment.
- Trust: Confidence in the efficacy and safety of these technologies.
- Engagement: Communities being involved in discussions around capture projects.
A government-funded study revealed that the acceptance of carbon capture technologies increased when individuals felt informed and engaged in the decision-making process. People are more likely to support initiatives if they understand how they contribute to mitigaring climate change. A lack of clarity or insufficient outreach can stymie potential advances in CO2 capture systems.
"To win the hearts of the public, transparency and education about CO2 capture technologies is paramount—it's not just about removing CO2, but also about building a bridge of trust."
Thus, bridging the gap between technology and societal acceptance is pivotal for the success of direct CO2 capture systems. Each challenge intertwined with this venture serves as a crucial piece of a much larger puzzle, essential for crafting effective climate strategies moving forward.
Environmental Impacts of CO2 Capture
Understanding the environmental impacts of direct CO2 capture is crucial in assessing the efficacy of these technologies in mitigating climate change. As the globe grapples with rising temperatures and erratic weather patterns, the need for effective carbon management strategies has never been more urgent. The environmental implications of the various methods employed in CO2 capture must be meticulously analyzed to ensure that the benefits outweigh any potential negative consequences. This section unpacks the intricacies involved in evaluating these impacts through lifecycle analyses and assessing their role in reducing the carbon footprint.
Lifecycle Analyses
Lifecycle analyses (LCA) offer a systematic approach to evaluating the environmental impacts of direct CO2 capture technologies from cradle to grave. This process involves looking at each stage—from raw material extraction, production, use, and disposal—to assess overall sustainability. For instance, carbon capture technologies that rely heavily on energy-intensive processes might contribute to CO2 emissions in their operational phase, potentially offsetting their intended benefits.
Several considerations come into play during lifecycle assessments:
- Material Inputs: The type of materials used in CO2 capture systems can play a monumental role in their environmental footprint. For example, utilizing non-renewable resources can deplete ecosystems, leading to adverse environmental effects.
- Energy Consumption: Energy required to run capture systems is not negligible. It's necessary to evaluate whether the energy used comes from renewable sources or fossil fuels. A system powered by solar energy differs vastly in sustainability compared to one that still leans on coal.
- End-of-Life Management: What happens when capture technologies reach the end of their lifespan? Understanding the waste generated and how it is disposed of is essential for a complete environmental picture.
"Life-cycle thinking allows us to understand the full environmental impacts of our actions, enabling better choices for a sustainable future."
Conducting LCAs thus serves as a powerful tool in identifying trade-offs and optimizing the operational strategies of direct CO2 capture technologies, ultimately contributing to more informed policy decisions and advancements in the field.
Reduction of Carbon Footprint
The role of CO2 capture in reducing the carbon footprint cannot be overstated. As industries lay heavy on the earth's resources, integrating these technologies into conventional practices could herald a marked shift towards carbon neutrality.
Several impactful aspects include:
- Mitigation of Emissions: By capturing CO2 at the source, industries can significantly lower the amount of greenhouse gases released into the atmosphere. Power plants, for instance, can implement direct CO2 capture systems to siphon off emissions before they even reach the air.
- Encouraging Sustainable Practices: The adoption of CO2 capture technology often encourages organizations to rethink their production and operational processes. It fosters a culture of sustainability that resonates through their supply chains and customer interactions.
- Long-Term Viability: While the technology's upfront costs might seem steep, the long-term reduction in carbon emissions will outweigh initial investments. This proposition encourages entities to look beyond immediate financial returns toward their larger environmental responsibilities.
Policy and Regulatory Landscape
Navigating the intricacies of direct CO2 capture requires an understanding of the policies and regulations shaping its development and implementation. The framework established by governments and international bodies plays a crucial role in incentivizing innovation, directing funding, and ensuring that technologies align with broader climate objectives. Without this governance, the momentum toward sustainable practices could stall, creating a gap between potential solutions and real-world application.
The importance of a well-structured policy is not solely about establishing guidelines; it is also about fostering an environment where capture technologies can thrive. Investments in infrastructure, research, and public awareness hinge on clear directives from policymakers. Additionally, as the urgency to combat climate change escalates, adapting existing rules to facilitate rapid advancements in technology becomes necessary.
International Agreements
International agreements, such as the Paris Accord, serve as a cornerstone for countries working together to address climate change. These agreements often include targets which compel nations to reduce greenhouse gas emissions. Direct CO2 capture finds its place within these frameworks as a specific strategy for meeting these ambitious goals.
Key elements of international agreements include:
- Establishing binding targets for emission reductions.
- Promoting sharing of technology and resources between countries.
- Providing funding and support for research and deployment in developing nations.
The cooperation catalyzed by these agreements can expedite the adoption of direct CO2 capture technologies, ensuring they become part of the climate solution toolkit. In many instances, nations report their progress through these international channels, leading to a competitive spirit aimed at technological advancement.
*"As the world looks to technological solutions to combat climate change, international agreements ensure that CO2 capture isn't just local but part of a global strategy."
National Policies
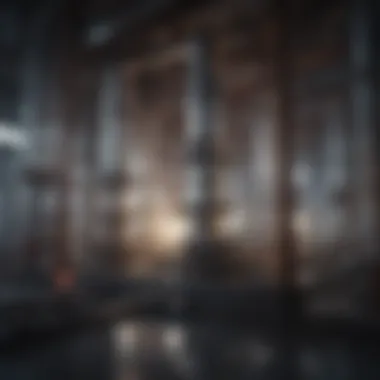
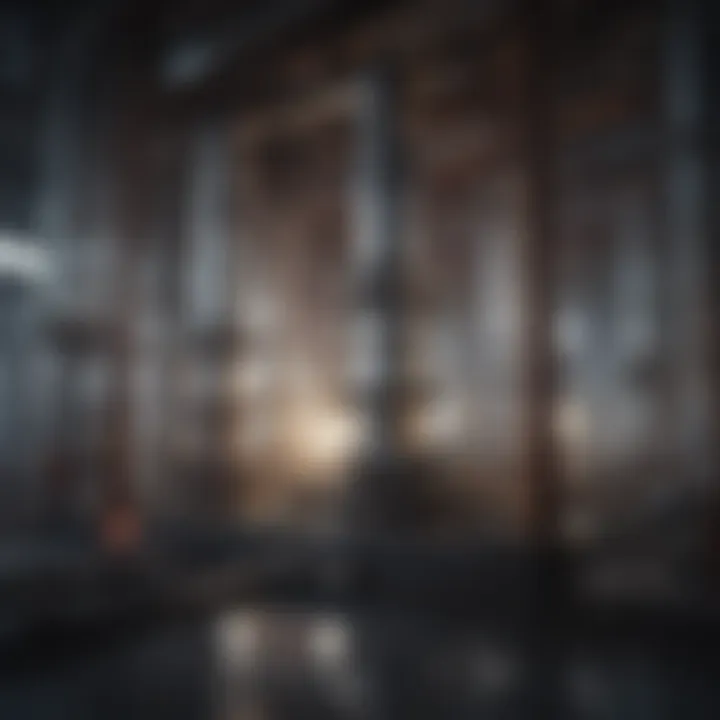
While international agreements set broad goals, national policies translate these targets into actionable frameworks. These policies shape how various regions approach direct CO2 capture, evaluate effectiveness, and allocate funding. They can range from tax incentives for companies deploying capture technologies to regulatory frameworks that dictate how much CO2 can legally be emitted.
Important aspects of national policies involve:
- Financial incentives: Subsidies and tax deductions can make the implementation of CO2 capture technologies more appealing for businesses.
- Research funding: Governments may allocate budgets towards supporting innovative research that enhances capture efficiency.
- Regulatory frameworks: Establishing guidelines on how and where CO2 capture should be applied.
In many nations, clarity in policy can result in increased investment from both the public and private sectors, facilitating the large-scale implementation that is essential for the success of CO2 capture strategies. Ultimately, strong national policies can mirror the ambitions expressed in international agreements, creating a cohesive approach towards reducing the overall carbon footprint.
Future Directions in CO2 Capture Technologies
The field of direct CO2 capture is evolving rapidly, becoming a beacon of hope in the quest for effective climate solutions. As concerns grow over greenhouse gas emissions and their impact on our planet, novel approaches and technologies come into sharp focus. This section delves into the future directions that CO2 capture technologies are heading towards, emphasizing the innovations on the horizon, the integration of these technologies with renewable energy systems, and the benefits that could spur their adoption.
Innovative Research Trends
Research into CO2 capture is replete with exciting developments, advancing methodologies that promise greater efficiency and lower costs. Many scientists are exploring the use of bioinspired materials, which mimic nature's processes for capturing and storing carbon, like the way trees absorb CO2. This biomimicry approach could yield novel materials that improve capture rates significantly.
Additionally, there’s growing interest in carbon capture utilization and storage (CCUS), which aims not only to capture CO2 but also convert it into useful products. For instance, ongoing research is focused on turning CO2 into fuels or valuable chemicals through various catalytic processes. This dual benefit can transform what was once seen as waste into commercially viable products.
Some researchers are also experimenting with new adsorbents that can selectively capture CO2 at lower energy costs. Innovations in porous metals and molecular sieves could lead to materials that capture CO2 more effectively at room temperature, potentially making the process more accessible and less energy-intensive. As the saying goes, "No stone should be left unturned," and the scientific community is leaving no stone unturned in their pursuit of the next breakthrough.
Integration with Renewable Energy
The interplay between CO2 capture technologies and renewable energy sources marks another frontier that holds significant potential. With the rise of solar, wind, and other clean energy resources, capturing CO2 can take on a synergistic role.
By integrating CO2 capture with renewable energy systems, we can produce energy while simultaneously offsetting emissions. For example, researchers are exploring how excess renewable energy can be used to power capture processes, making CO2 removal more sustainable.
This integration also opens up avenues for on-site capture at sources of renewable energy generation, wherein CO2 is captured as electricity is produced. This combined approach not only improves the overall efficiency of energy systems but also addresses the carbon footprint of electricity generation.
Furthermore, pairing direct CO2 capture with hydrogen production from renewable sources can lead to cleaner fuel alternatives. This aligns with the transition to a hydrogen economy, fundamentally reshaping our energy landscape.
"In a world of limited resources, it’s a game-changer to turn pollution into potential by leveraging nature’s own remedies alongside tech prosperity."
In summary, as we stride towards a better future, the promising innovations and integrations in CO2 capture technologies offer much-needed hope. Fostering these advancements will require collaboration across disciplines, but the prospects are as vast as they are vital for sustainable progress.
Case Studies and Real-World Applications
The practical application of direct CO2 capture technologies offers invaluable insights into their real-world effectiveness and the challenges they face. This section shines a spotlight on case studies that exemplify successful implementations, while also deriving lessons from industry leaders embracing these technologies. Understanding these elements enriches our comprehension of how direct CO2 capture can contribute meaningfully to global efforts in mitigating climate change.
Successful Implementations
There are several noteworthy examples that illustrate the successful deployment of direct CO2 capture technologies in various sectors. One prime example is Climeworks, which operates facilities in Europe that utilize direct air capture to extract CO2 from the atmosphere. Climeworks has been operational since 2017, and its technology captures approximately 4,000 tons of CO2 annually. The captured gas can then be utilized for various purposes, including carbonated drinks, or even stored deep underground in geological formations. This scalability demonstrates that direct air capture can be economically viable and practically feasible.
Another prominent project is the Carbon Clean Solutions, implemented at a cement plant in the UK. Here, direct CO2 capture is integrated into the existing infrastructure, effectively capturing emissions from the production process. With this method, the company successfully reduces over 50,000 tons of CO2 every year. This showcases how industries traditionally linked to high emissions can innovate and adapt systems that curtail their carbon footprint, contributing positively to climate goals.
Lessons from Industry Leaders
Industry leaders provide insights into best practices and potential pitfalls when it comes to direct CO2 capture technology. A critical lesson from ExxonMobil is the need to align technological advancement with robust public communication strategies. Successfully implementing capture systems often hinges on addressing public concerns, which helps in fostering acceptance and funding opportunities for these projects.
Moreover, a case from Shell's work in Canada reveals the effectiveness of collaborative approaches. Shell partners with various stakeholders, including local governments and environmental organizations, to promote understanding and support for their projects. Such collaboration not only enhances the credibility of the initiatives but also could lead to pooling resources for more comprehensive projects.
"The true potential of direct CO2 capture lies not just in technology but in how we interact with communities and drive collective action."
— Industry Leader Insight
Lastly, the importance of diversification in capturing carbon was exhibited by CarbonCure Technologies. They have found unique methods to utilize captured CO2 in concrete production, which not only sequesters emissions but also enhances the strength of concrete itself. Their ability to marry sustainability with economic advantage highlights how innovation can lead to breakthrough practices in carbon management.
These cases collectively underscore the practical benefits and opportunities emerging from direct CO2 capture applications. Each example and lesson brings us closer to a clearer path for integrating these technologies into the fabric of various industries, aiding the global fight against climate change.
Finale
As we wrap up this exploration into direct CO2 capture technologies, it is crucial to acknowledge the significant role they play in addressing one of the most pressing challenges of our time: climate change. This article has provided insights on various aspects of CO2 capture, revealing how innovative methods and technologies are evolving to meet the demands of a changing environment.
Summarizing Key Points
In summary, the journey through the different techniques of direct CO2 capture has unveiled several critical takeaways:
- Diverse Methods: From adsorption to cryogenic techniques, each method has unique merits and limitations that determine its applicability in real-world scenarios.
- Efficiency and Costs: Understanding the efficiency metrics alongside the associated costs is fundamental for stakeholders aiming to implement these technologies.
- Challenges: Both technological and economic barriers persist, posing significant challenges. Moreover, addressing public perception is equally important to foster acceptance and support for such initiatives.
- Environmental Benefits: Lifecycle analyses indicate that while the technologies represent a significant step toward reducing carbon footprints, they must be part of broader environmental strategies.
- Policy Influence: The regulatory landscape shapes the practical implementation of CO2 capture, often determining its feasibility and scalability.
These points emphasize the multifaceted nature of direct CO2 capture, highlighting its potential while also acknowledging the hurdles that need addressing.
Final Thoughts on Future Prospects
Looking forward, the future of direct CO2 capture technologies is both promising and uncertain. Researchers are pushing boundaries in innovative approaches. There’s a growing synergy between CO2 capture and renewable energy systems that could revolutionize the sector. By integrating these technologies, we could not only capture carbon effectively but also utilize it as a resource, enhancing sustainability.
The pursuit for cleaner air is not merely a scientific endeavor; it’s a survival imperative.
As awareness grows, we are likely to see increased investment and interest from both government and private sectors. With the right frameworks, advancements in technology could render CO2 capture not only essential but economically viable. The path ahead calls for collaboration among academia, industry leaders, and policymakers to pave the way for technologies that can turn the tide against rising carbon levels.