DLP 3D Printing: Principles, Applications, and Future
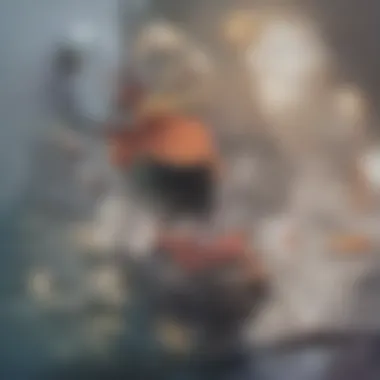
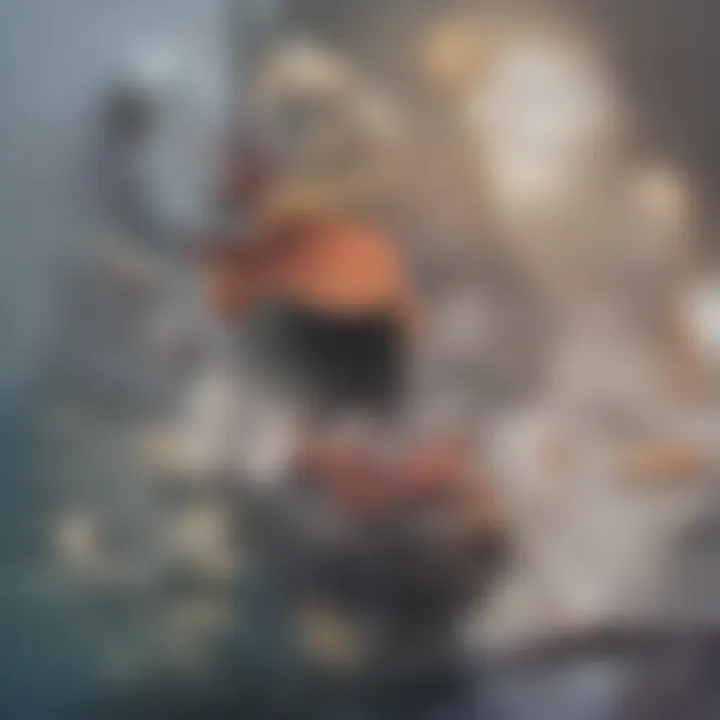
Intro
Digital Light Processing (DLP) 3D printing has become a pivotal technology in the landscape of additive manufacturing, distinguished for its precision and versatility. As industries evolve, the demand for innovative materials and sophisticated fabrication techniques rises. DLP 3D printing responds to this need by enabling rapid prototyping and production in fields such as healthcare, automotive, and consumer goods.
This article will serve as a comprehensive guide to DLP 3D printing, covering its essential principles, operational mechanics, diverse applications, and future directions. By analyzing both the advantages and limitations of this technology, we will gain a nuanced understanding of its place in the broader context of 3D printing methodologies. We will examine current trends, innovations, and potential transformations in DLP technology.
Research Methodology
Description of research design and approach
In studying DLP 3D printing, a multi-faceted research approach is utilized. The investigation integrates qualitative assessments of existing literature and quantitative analysis from various case studies. By synthesizing historical data and current technological assertions, we are able to paint a complete picture of DLP's evolution and current standing.
Materials and methods used in the study
This research relies on academic journals, industry reports, and participatory studies from manufacturers. Key materials include:
- Peer-reviewed articles: These sources provide insights into technical advancements and academic discussions surrounding DLP's efficacy.
- Case studies: Real-world applications offer practical perspectives on DLPβs impact across sectors.
- Manufacturer specifications: Technical documents from leading manufacturers highlight material compatibility and performance metrics of DLP printers.
The combination of these materials allows for a thorough exploration of current and future trends in DLP technology.
Discussion and Interpretation
Interpretation of results in the context of existing literature
The current findings indicate a positive trajectory for DLP 3D printing, affirming its relevance against other methods such as FDM and SLA. DLP's ability to utilize a wide variety of photopolymer resins makes it increasingly attractive for meticulous, detail-oriented applications. Furthermore, the efficiency of DLP printers in producing high-resolution prints within a short timeframe is consistently echoed throughout the literature.
DLP 3D printing is consistently praised for its speed and resolution, winning preference in applications demanding precision.
Implications for future research or practical applications
The implications of this study extend beyond simply affirming DLP's current relevance. With ongoing advancements, particularly in material science and processing capabilities, there is considerable scope for DLP technology to penetrate new markets. The encouragement of research into biocompatible materials presents exciting possibilities for healthcare applications, including prosthetics and dental products.
Additionally, understanding the limitations of DLP, such as its dependence on photopolymerization and the resulting environmental impacts, will be crucial for guiding future developments. Innovative approaches to mitigate these drawbacks may lead to a more sustainable integration of DLP printing techniques.
Prelims to DLP 3D Printing
DLP (Digital Light Processing) 3D printing is an innovative technology that has gained notable prominence in additive manufacturing. It plays a significant role in various industries due to its efficiency and precision. Understanding its principles and operational mechanics is essential for students, researchers, and professionals who are engaged with or interested in 3D printing.
Overview of 3D Printing Technologies
3D printing encompasses a range of technologies that enable the creation of three-dimensional objects from a digital file. Among the most common methods are Fused Deposition Modeling (FDM), Stereolithography (SLA), and DLP. Each of these techniques has unique attributes that cater to different needs in terms of production speed, material selection, and detail accuracy.
- Fused Deposition Modeling (FDM): Utilizes thermoplastic materials that are heated and extruded through a nozzle. It is popular for its simplicity and cost-effectiveness but is often limited in resolution compared to DLP.
- Stereolithography (SLA): Involves a laser that cures photopolymer resin layer by layer. While SLA produces high-quality parts, the process can be slower and may require a longer curing time.
- Digital Light Processing (DLP): Uses a digital light projector to flash images of each layer onto a vat of resin. This method allows for faster printing compared to SLA and can achieve high resolution and fine details.
The diversity of 3D printing technologies facilitates advancements in prototyping and manufacturing across sectors, including medical, industrial, and educational fields.
Understanding DLP Technology
DLP technology employs light to manipulate photopolymer resins, transforming them into solid objects. The key components of this process include a light source, a digital micromirror device (DMD), and a vat containing liquid resin. The DMD plays a crucial role by allowing precise control of the light patterns projected onto the resin.
- Light Source: Typically a digital light projector or an LED, which emits the light required to cure the resin. It can provide a uniform beam, essential for layer curing.
- Digital Micromirror Device (DMD): Comprises thousands of tiny mirrors, each representing a pixel. These mirrors tilt to reflect light either toward the resin or away from it, enabling the projection of images layer by layer.
- Curing Process: DLP operates by exposing a layer of resin to the light projected from the DMD. The light solidifies the resin in specific areas, creating the desired layer of the object. This method of layer curing allows for faster printing speeds compared to other techniques.
The efficiency and accuracy of DLP technology make it an appealing choice for many applications, resonating with the current demands for high-quality 3D printed objects.
The Mechanics of DLP 3D Printing
Understanding the mechanics of DLP 3D printing is fundamental. This section serves to elucidate the core principles that drive the technology. It gives insight into how DLP functions, highlighting the key components and processes that enable effective additive manufacturing. Knowledge of these mechanics is crucial for students, researchers, and professionals aiming to leverage DLP technology in various fields.

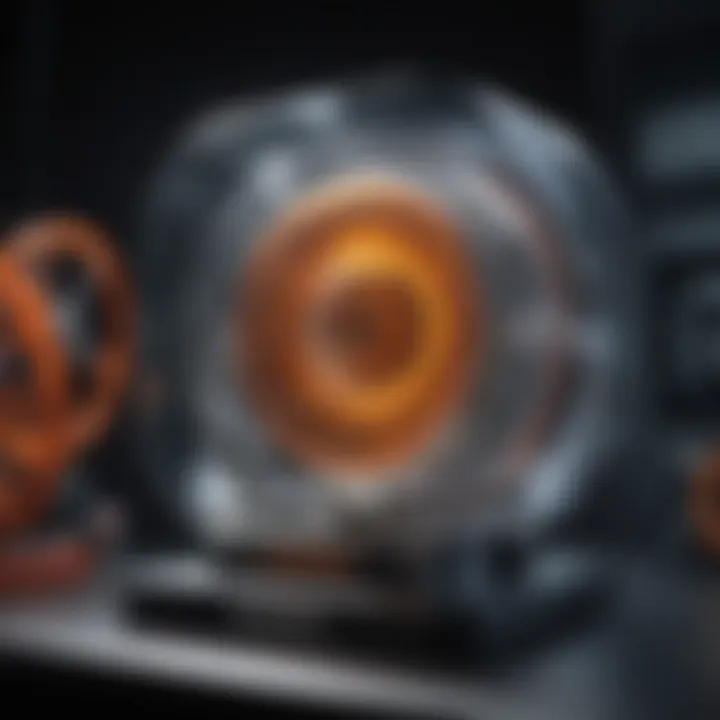
Principle of Light Projection
At the heart of DLP 3D printing lies the principle of light projection. This innovative process utilizes a digital light projector to cure photopolymer resin into solid objects layer by layer. The projector displays a cross-sectional image of the object being created. Each layer is illuminated by a high-intensity light source, facilitating rapid curing of the resin. This technique allows for a high precision and efficient production of complex geometries.
The light source's wavelength is typically in the ultraviolet spectrum, which activates the photoinitiator in the resin. When exposed, the liquid resin converts into a solid state. This transformation makes it possible to create detailed features with smooth surface finishes. Furthermore, the projection technique permits multiple layers to be cured simultaneously, significantly reducing production time compared to traditional methods.
Layer Curing Process
The layer curing process is critical to the successful implementation of DLP technology. Initially, a thin layer of resin is spread over the build platform. Once the projector beams the cross-sectional pattern onto this layer, selected areas of the resin harden due to the light exposure. After the first layer has cured, the build platform moves down slightly to prepare for the next layer.
This sequential curing continues until the entire object is formed. Notably, the layer thickness can be adjusted depending on the desired final properties of the printed part. Ranging from 0.1 mm to 0.25 mm, thinner layers yield finer details but increase print duration. A balance must be made between detail and efficiency during this process.
"The layer curing process is what distinguishes DLP from other 3D printing methods, allowing for rapid production without sacrificing detail."
Hardware Components
The hardware components involved in DLP 3D printing play a pivotal role in the overall functionality of the system. The primary components include the light source, the build platform, the resin tank, and the control unit.
- Light Source: Generally, DLP 3D printers use a digital light projector, often consisting of a bright LED. The resolution of the projector dictates how finely detailed the layer images will be.
- Build Platform: This platform holds the object as it is printed and moves up or down to permit layer formation.
- Resin Tank: The tank contains the liquid resin. Its design allows for easy displacement of the uncured resin as layers are formed.
- Control Unit: This system directs the operations of the printer, ensuring precise timing and the sequence of light projections.
This combination of components works in unison to create a seamless experience of turning digital designs into tangible objects. By grasping the mechanics of these elements, users can effectively troubleshoot and optimize their DLP 3D printing processes.
Materials Used in DLP 3D Printing
The materials used in DLP 3D printing serve as the foundation of this technology. The choice of materials not only influences the quality and durability of the printed objects but also determines the range of applications for which DLP is suitable. Understanding the types of resins and their properties helps in making informed decisions regarding material selection for specific applications.
Types of Resins
In DLP 3D printing, the primary material is photopolymer resin. These resins react to UV light, curing and solidifying to form intricate structures. There are several categories of resins based on their characteristics:
- Standard Resins: These are suitable for general-purpose applications. They are often used for creating prototypes and models with decent detail and moderate strength.
- Dental Resins: Specifically formulated for dental applications, these resins exhibit properties that induce biocompatibility and resistance to dental chemicals. They are used for producing dental models, crowns, and bridges.
- Castable Resins: These resins burn out cleanly when heated, making them ideal for jewelry making. They allow for precise detail that is essential for casting.
- Flexible Resins: These materials retain some elasticity after curing, making them suitable for applications requiring movement or deformation, such as gaskets and seals.
- High-Temperature Resins: Capable of withstanding high heat, these are utilized in applications where thermal resistance is critical, such as automotive parts.
- Durable Resins: Designed for strength and stability, durable resins are commonly used in functional prototypes needing toughness and resistance to wear.
Material Properties and Selection
When selecting a resin for DLP 3D printing, several properties must be considered:
- Viscosity: The flow characteristics of the resin affect the printing process. Lower viscosity resins are easier to work with but may require specific conditions to cure accurately.
- Cure Time: Different resins have varying curing times, which can impact production speed.
- Mechanical Properties: These include strength, flexibility, and brittleness. Depending on the final application, one might choose a resin that offers the appropriate balance of these properties.
- Color and Finish: Resins come in a variety of colors and finishes, which can affect the aesthetic quality of the printed object.
- Cost: Budget constraints might limit options for high-end specialty resins.
"The choice of resin directly influences the capabilities of DLP 3D printing, shaping not just the product's aesthetics but its functional performance as well."
Ultimately, the understanding of materials used in DLP 3D printing is critical for achieving desired outcomes across various applications. This knowledge allows students, researchers, and professionals to innovate and enhance their projects effectively.
Applications of DLP 3D Printing
The applications of DLP 3D printing are vast and diverse, showcasing its utility across multiple fields. This technology stands out due to its speed, precision, and the versatility of materials it can use. Understanding the specific applications can offer insights into the future directions of this technology and its potential impact on various industries.
By examining its different applications, professionals can better grasp how DLP printing is redefining manufacturing, healthcare, education, and research. This section will detail significant areas where DLP 3D printing plays a crucial role, along with the unique benefits each sector experiences.
Industrial Manufacturing
DLP 3D printing has made significant inroads into industrial manufacturing primarily due to its speed and accuracy. This method is particularly advantageous for producing prototypes and small runs of custom parts. Industries utilize DLP printing for rapid tooling and producing molds, allowing for faster iterations in the manufacturing process.
Benefits include:
- Reduction of lead times: Parts can be printed quickly, accelerating project timelines.
- Customization capabilities: DLP technology allows for precise customizations without the need for large-scale retooling.
- Material efficiency: Less waste is produced during the printing process compared to traditional methods, which is increasingly important in lean manufacturing environments.
DLP printing is also used in the production of complex geometries that may be too difficult or expensive to achieve through traditional methods. Companies that harness these capabilities can gain a competitive edge.
Medical and Dental Applications
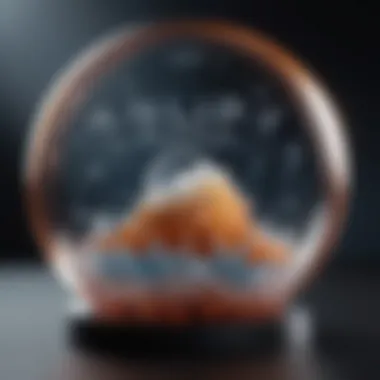
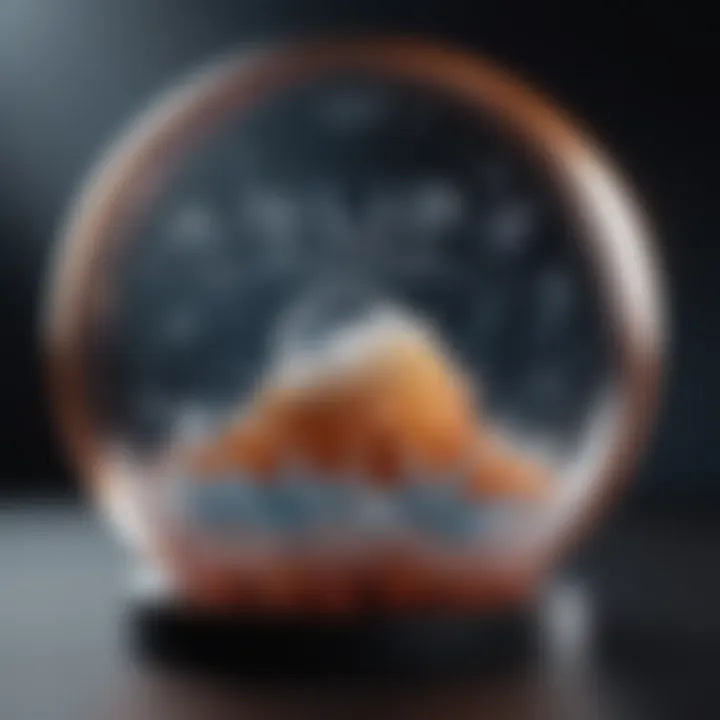
In the medical and dental fields, DLP 3D printing has proven transformative. The ability to create precise, patient-specific models, guides, and prosthetics is revolutionizing patient care and enhancing surgical outcomes.
Key applications include:
- Surgical guides: DLP technology enables the production of tailored surgical guides, improving the accuracy of procedures.
- Dental implants: Precision is crucial in dental applications. DLP printing allows for highly detailed and custom-fit dental restorations, like crowns and bridges.
- Orthotics and Prosthetics: Custom-made orthotic devices are easier to produce with DLP, providing much-needed comfort and functionality to patients.
Educational and Research Contexts
DLP 3D printing is also finding its place in educational and research settings. This technology offers students and researchers the opportunity to engage deeply with additive manufacturing processes. Incorporating DLP printing into academia can foster innovation and creativity.
In educational settings, DLP applications include:
- Hands-on learning: Students learn about 3D printing fundamentals through interactive projects and experiments.
- Prototype development: DLP technology allows rapid prototyping for research projects, helping researchers validate their concepts swiftly.
- Access to advanced materials: Educational institutions often collaborate with industry partners, giving students exposure to a wide range of materials and techniques.
Advantages of DLP 3D Printing
The advantages of DLP 3D printing play a critical role in its adoption across various industries. Understanding these benefits assists stakeholders in making informed decisions regarding the integration of this technology into their operations. DLP printing is not only efficient but also delivers high-quality outputs, making it a preferred choice for diverse applications. Below, we examine three key advantages: speed of production, precision and detail, and cost-effectiveness.
Speed of Production
One notable advantage of DLP 3D printing is the speed of production. This method utilizes digital light to cure entire layers of resin simultaneously. Unlike filament-based methods that print layer by layer, DLP technology projects light onto a resin vat, curing large portions at once. This capability significantly reduces print times, making it possible to produce complex models quickly.
Faster production is especially beneficial in industries where time is crucial, such as rapid prototyping and product development. For instance, manufacturers can create prototypes in hours rather than days, reducing lead times and speeding up the product development cycle.
Moreover, shorter turnaround times enable businesses to respond quickly to market demands, maintaining a competitive edge. The time saved can also be reinvested in design improvements or additional projects, amplifying productivity on many levels.
Precision and Detail
DLP 3D printing is renowned for its precision and attention to detail. The technology allows for the creation of intricate and complex geometries, thanks to its superior resolution. The light source, typically a digital light projector, can achieve finer details compared to many other printing methods.
This high level of accuracy is crucial in sectors where exact specifications are required, such as medical, dental, and jewelry manufacturing. For example, in the dental industry, DLP has empowered dentists to create detailed crowns and braces tailored to individual patients with utmost precision.
In addition, the smooth surface finish of printed objects reduces the need for extensive post-processing, saving both time and resources. This combination of precision and efficiency ensures that the final products meet strict quality standards and aesthetic demands in competitive markets.
Cost-Effectiveness
Cost-effectiveness is another essential advantage of DLP 3D printing. While the initial investment in DLP equipment may be higher than that for traditional methods like FDM, the long-term savings can be substantial. Reduced material waste is a key factor contributing to overall cost savings.
Unlike some other printing methods, DLP uses liquid resins, which means excess material can be recycled. This efficiency translates into lower operational costs and minimized resources spent on raw materials.
Additionally, the speed and precision of DLP printing lead to fewer defects and the need for less rework, further driving down costs. Businesses can achieve high-quality outputs without overspending, enhancing their bottom lines.
"DLP 3D printing combines rapid production, exceptional detail, and cost benefits, making it a powerful tool for industries seeking efficiency and quality."
Limitations of DLP 3D Printing
Understanding the limitations of DLP 3D printing is essential for professionals and researchers aiming to maximize its potential while also recognizing areas for improvement. Despite its advantages in speed and precision, DLP technology has some downsides that can affect its applicability in various scenarios. Knowing these limitations helps in making informed decisions during material selection and application processes.
Material Limitations
DLP printing relies heavily on photopolymer resins. These materials come with inherent limitations that impact the final productβs properties. One significant aspect to consider is the
- Sensitivity to Environmental Factors: Many resins can be sensitive to temperature and humidity, which can lead to inconsistent results if not properly controlled.
- Limited Mechanical Properties: Certain resins tend to be brittle compared to thermoplastics used in FDM printing. This makes them less suitable for functional parts that require significant durability or flexibility.
- Thermal Stability: DLP resins may degrade at elevated temperatures, limiting their use in high-temperature applications.
Thus, selecting the right resin is essential depending on the intended application, but it often narrows down the versatility of DLP printing in various sectors.
Post-Processing Requirements
Post-processing is another crucial aspect of DLP printing. Once a print is complete, it typically requires extensive post-processing steps to achieve the desired level of finish.
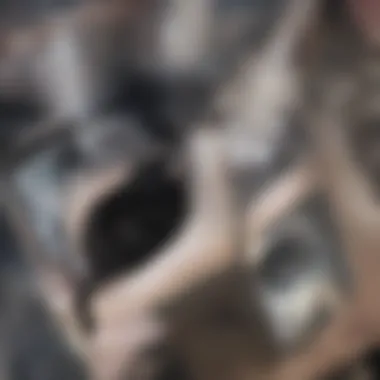
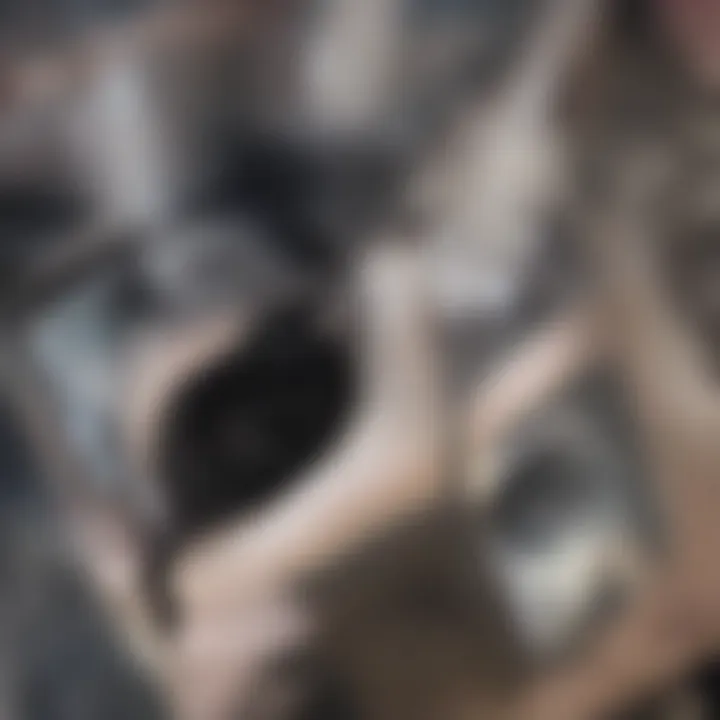
- Washing and Curing: After printing, parts must be washed in isopropyl alcohol to remove uncured resin. This is a time-consuming process and may introduce risks if not done properly.
- Surface Treatment: Achieving a smooth surface finish often necessitates additional steps like sanding or coating, which further extends production time and costs.
- Handling Safety: The resins used in DLP printing can be toxic. Proper safety precautions, including gloves and fume extraction systems, are vital during handling and processing.
Overall, the need for meticulous post-processing can hinder the speed advantage normally associated with 3D printing technologies. Understanding these limitations enables users to make the best use of DLP capabilities while being prepared for the challenges involved.
Comparative Analysis of 3D Printing Methods
In the ever-evolving landscape of additive manufacturing, understanding the different methods of 3D printing is essential for making informed decisions. This section provides a comprehensive comparative analysis of various 3D printing methods, specifically focusing on Fused Deposition Modeling (FDM) and Stereolithography (SLA) in relation to Digital Light Processing (DLP). Analyzing these technologies offers valuable insights into their respective strengths, weaknesses, and ideal applications.
FDM vs. DLP
Fused Deposition Modeling (FDM) is one of the most widely used 3D printing techniques. It involves melting thermoplastic filaments and depositing them layer by layer to create an object. In contrast, DLP uses a digital light projector to cure liquid resin, forming layers with high precision.
The primary differences between FDM and DLP can be outlined as follows:
- Material Use: FDM utilizes filament materials, which can be more versatile in terms of physical properties. DLP predominantly relies on liquid resins, which can produce higher detail but might have a narrower range of material properties.
- Print Speed: DLP often outruns FDM in production speed. Since DLP cures an entire layer at once rather than line by line, it typically results in faster turnaround times.
- Print Resolution: DLP provides superior print resolution due to the controlled projection of light, which allows for intricate details in finished prints. Meanwhile, FDM printers can face challenges with detail fidelity, especially on complex geometries.
- Post-Processing: FDM prints generally require less post-processing when finished, as they are usually ready to use after cooling. Conversely, DLP prints often require washing and curing stages to finalize their strength and aesthetics.
These factors make FDM suitable for rapid prototyping and functional parts, while DLP shines in applications demanding high detail, such as jewelry or dental impressions.
SLA vs. DLP
Stereolithography (SLA) is another prominent 3D printing method that, like DLP, utilizes photopolymerization to create parts from liquid resin. However, SLA uses a laser to cure the resin point-by-point, while DLP employs a light projector to cure an entire layer.
Comparing SLA to DLP reveals several key distinctions:
- Layer Curing Technology: DLP's approach generally leads to faster print times since it cures whole layers at once, as opposed to the sequential point treatment in SLA.
- Surface Finish: Although both methods can produce high-quality surface finishes, DLP often results in smoother surfaces due to the curing process, reducing the need for extensive post-processing in some cases.
- Dimensional Accuracy: DLP often provides better dimensional accuracy and consistency across multiple prints because of its uniform light exposure. SLA may face variability, especially in larger or more complex structures.
- Material Range: While SLA has been traditionally viewed as limited in terms of material variety, recent advancements are broadening its material capabilities. DLP, however, benefits from a more extensive selection of specialized resins optimized for various applications.
The choice between SLA and DLP is often based on specific project requirements such as speed, detail, and material properties. This understanding enhances decision-making for users in various industries, such as healthcare and manufacturing.
"Understanding the strengths and weaknesses of each method is crucial for the successful application of 3D printing technology."
Future Trends in DLP 3D Printing
The topic of future trends in DLP 3D printing is crucial in understanding how this technology will evolve and adapt. As industries seek more efficient and versatile manufacturing solutions, the evolution of DLP technology plays a pivotal role. Enhanced materials, innovative processing methods, and greater integration with other technologies are shaping the landscape of additive manufacturing. Engaging with these trends is essential for professionals and researchers who aim to stay ahead in their respective fields.
Innovations in Material Science
Advancements in material science are expected to transform DLP 3D printing significantly. One of the most promising areas is the development of new resin formulations. These resins offer enhanced properties, such as increased strength, flexibility, or specific thermal characteristics. Researchers increasingly explore biocompatible materials for medical applications, allowing for more specialized and safe implants or prosthetics. This focus on innovative materials can lead to an expanded range of applications and improved performance in existing uses.
Furthermore, techniques like combining polymers with nanoparticles are gaining attention for creating composite materials. These composites may exhibit superior mechanical properties or unique functionalities. The versatility of these materials allows DLP to compete effectively against traditional manufacturing processes, opening new avenues for designers and engineers.
Emerging Technologies
Embracing emerging technologies plays a vital role in shaping the future landscape of DLP 3D printing. One trend is the integration of machine learning and artificial intelligence in the printing process. By leveraging data, these systems can enhance printing efficiency, optimize designs, and reduce errors. A more intelligent process can lead to faster iterations of prototypes and a swifter response to market demands.
Moreover, the expansion of hybrid manufacturing methods is notable. The combination of DLP with subtractive methods enhances capability, allowing for intricate designs that require precision beyond what solely additive processes can achieve. The potential for an interconnected ecosystem, where DLP systems communicate with other manufacturing technologies, will further streamline production lines.
The fusion of DLP 3D printing with digital workflows, often referred to as Industry 4.0, signifies a shift towards more integrated and automated manufacturing environments. This convergence can result in significant efficiency gains and cost reductions while maintaining quality and precision. Each of these innovations foreshadows a continually evolving DLP landscape, emphasizing the need for ongoing research and adaptation in the industry.
End
The conclusion of this article is a significant aspect, as it encapsulates the essence and relevance of DLP (Digital Light Processing) 3D printing. By synthesizing all present insights about DLP technology, one can appreciate not just its achievements but its broader implications in various fields. DLP 3D printing stands out due to its precision and speed, making it highly suitable for applications ranging from industrial manufacturing to healthcare.
Summary of Key Insights
DLP 3D printing offers numerous advantages, including:
- Speed and efficiency: DLP machines can produce detailed objects much faster than some other methods, providing an edge in fast-paced industries.
- Quality of details: The technology enables high-resolution outputs, which are crucial in sectors that demand intricate designs like jewelry and dental prosthetics.
- Material versatility: A wide range of resins can be employed in DLP printing, allowing users to tailor materials to suit specific needs, such as biocompatibility in medical applications.
Moreover, limitations like material constraints and necessary post-processing cannot be ignored. Challenges faced in the realm of resin stability and curing conditions might dictate the feasibility of DLP in various cases. A clear understanding of these elements aids users in making informed decisions about technology application.
The Future of DLP 3D Printing
The future of DLP 3D printing appears promising, with continuous advancements in both hardware and material science. Innovations such as new photopolymer materials may lead to greater functional properties, widening the scope of applications. There is growing interest in exploring new sectors, including aerospace and automotive, which may leverage DLPβs unique capabilities.
Furthermore, emerging technologies may further integrate with DLP systems, enhancing their performance and reliability. As the demand for customized and efficient solutions grows, DLP 3D printing might evolve into a standard practice across multiple industries, solidifying its place in the additive manufacturing landscape.