Exploring MEMS Mirrors: Innovations and Applications
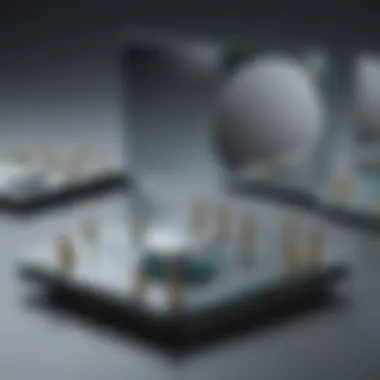
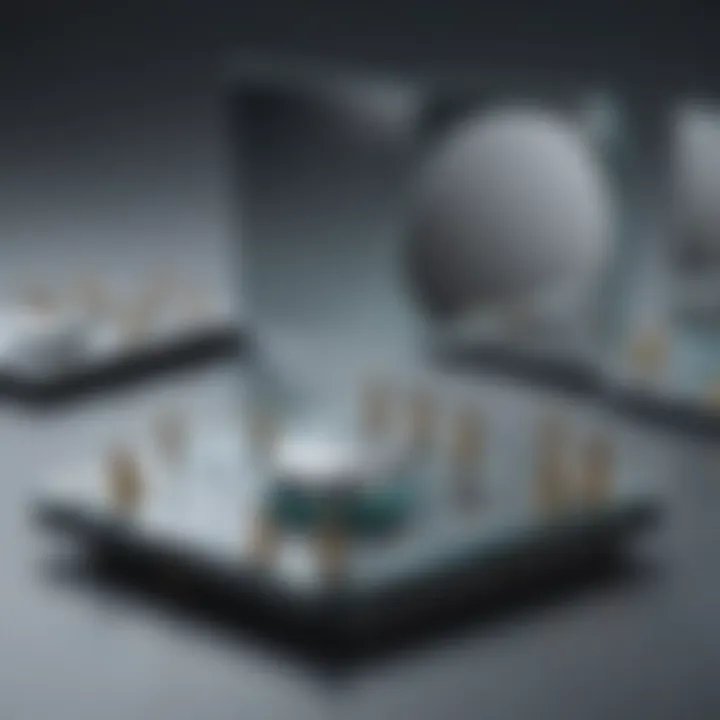
Intro
Micro-Electro-Mechanical Systems (MEMS) mirrors are integral to modern optics and imaging. Their unique design and functionality set them apart from traditional mirror technologies. This section will outline the critical aspects that contribute to their increasing application across various industries.
Understanding MEMS mirrors starts with their design principles rooted in microfabrication techniques. These techniques enable the creation of small-scale devices with impressive precision, allowing for advancements in both performance and capability. MEMS mirrors can manipulate light through electrostatic or electromagnetic actuation, greatly enhancing their versatility.
Further, this article will provide an in-depth exploration of the materials and methods that impact the functionality of MEMS mirrors. Various materials like silicon, polymers, and metals play a crucial role in determining their reliability and efficiency.
In examining the applications of MEMS mirrors, this article will emphasize areas such as telecommunications and biomedical imaging. Each of these sectors demonstrates significant advancements due to the integration of MEMS technology.
Finally, discussions on recent innovations and future trends in MEMS technology will show how research in this domain is evolving, addressing challenges and enhancing commercial viability. Headlines in MEMS development promise to shape the market and drive further research initiatives.
Prologue to MEMS Mirrors
The exploration of Micro-Electro-Mechanical Systems (MEMS) mirrors is paramount in the evolving landscape of optics and imaging technologies. This section aims to elucidate the significance of MEMS mirrors, emphasizing their multifunctionality and diverse applications. Understanding MEMS technology is not only critical for researchers and industry professionals but also for students who aspire to innovate within this domain.
Defining MEMS Technology
MEMS technology can be defined as the integration of mechanical and electrical components at the microscale. This technology is characterized by miniaturization, enabling the fabrication of devices that can perform precise mechanical functions. MEMS mirrors, as a subset of this technology, demonstrate exceptional capabilities in manipulating light with high accuracy. They are typically composed of movable components that can redirect light beams, making them invaluable in several sectors.
Key benefits of MEMS mirrors include:
- Compact Size: Their small form factor allows for integration into tight spaces, enabling sophisticated devices with reduced footprint.
- Low Power Consumption: Compared to traditional optical devices, MEMS mirrors require significantly less power.
- Rapid Response Times: These mirrors can operate at high speeds to facilitate real-time applications, enhancing user experiences in optical communications and imaging.
Historical Context and Development
The history of MEMS mirrors reflects rapid advancement in both technology and application. Initially, MEMS technology emerged in the late 20th century, driven by developments in semiconductor fabrication techniques. The early iterations of MEMS mirrors were used primarily in telecommunications for data routing. With time, applications expanded into fields such as medical imaging, automotive sensors, and consumer electronics.
The development of MEMS mirrors has paved the way for innovations, especially concerning manufacturing processes and performance metrics. Research and development efforts focused on improving actuation methods, materials, and design. The historical evolution showcases a trajectory that aligns with broader technological advancements, indicating a robust framework for future innovations in this space.
"MEMS mirrors not only signify advances in optics but also demonstrate the convergence of microfabrication and emerging technologies, pointing to exciting future possibilities."
In summary, the introduction to MEMS mirrors highlights their essential role in contemporary optical systems. By establishing a clear understanding of MEMS technology and its historical development, readers can better appreciate the subsequent sections. This knowledge will lay a solid foundation for delving into the fundamental principles, applications, and innovations surrounding MEMS mirrors.
Fundamentals of MEMS Mirrors
The topic of Fundamentals of MEMS mirrors is crucial in understanding how these devices operate and their significance in various applications. MEMS mirrors rely on specific principles and designs that govern their functionality, making it imperative to comprehend these fundamentals. By grasping these concepts, readers will appreciate the intricacies involved in designing MEMS mirrors and how advances in this field can significantly impact optics and imaging technology.
Operational Principles
Electrostatic Actuation
Electrostatic actuation is a primary mechanism used to control MEMS mirrors. It operates by creating an electric field between the fixed and movable parts of the device. This field generates a force that results in the movement of the mirror. The key characteristic that makes electrostatic actuation so beneficial is its efficiency in converting electrical energy into mechanical motion. This efficiency translates to rapid response times, allowing for high-speed applications in various fields.
One unique feature of electrostatic actuation is its ability to function without any moving parts beyond the mirror itself. This creates fewer points of failure, enhancing the reliability of the device. However, there are disadvantages, including the necessity for high voltage levels, which can complicate the design and limits some applications. Nonetheless, electrostatic actuation remains a popular choice due to its benefits in precision control and rapid response.
Piezoelectric Actuation
Piezoelectric actuation provides an alternative mechanism for driving MEMS mirrors. This method relies on piezoelectric materials, which change shape when an electric field is applied. This transformation allows for precise control over the mirror's position. The key characteristic of piezoelectric actuation is its ability to generate significant displacement with relatively low power.
A unique feature of piezoelectric actuation is its linear response, which is advantageous in applications requiring accurate positioning. One disadvantage, however, is that piezoelectric materials can be more vulnerable to fatigue over time compared to electrostatic systems. Despite this, piezoelectric actuation is favored in applications needing exceptional precision, such as in medical imaging technologies.
Design Parameters
Size and Shape
Size and shape are critical design parameters for MEMS mirrors. The dimensions of the mirror influence its optical performance, such as reflectivity and beam quality. Smaller mirrors can facilitate finer control and are suitable for compact systems, while larger mirrors are necessary for specific applications requiring broader light beams. The optimal size and shape also depend on the desired operational wavelength and the type of actuation used.
One unique feature to consider is that the aspect ratio of a mirror can impact its stiffness and response time. A higher aspect ratio may improve sensitivity but can adversely affect robustness. Therefore, careful consideration of size and shape is essential to achieve a balance between performance and durability.
Material Selection
Material selection plays a significant role in determining the performance of MEMS mirrors. Common materials include silicon, polymers, and specialized ceramics. Each material has distinct properties that affect the mirror's weight, thermal stability, and response time.
For instance, silicon's high stiffness and ease of fabrication make it a preferred choice in many applications. On the other hand, polymers can offer flexibility and lower weight, advantageous in specific device applications. However, the trade-off may include reduced thermal stability compared to more robust materials like silicon.
Fabrication Techniques
Fabrication techniques for MEMS mirrors play a crucial role in determining their performance, reliability, and scalability. These methods influence the characteristics of the mirrors, including their size, shape, and the precision of their movements. Understanding these techniques helps in maximizing the advantages of MEMS technology and developing innovative applications across various fields. Here, we delve into two primary fabrication processes: photolithography and etching processes, which include dry etching and wet etching. Each method contributes uniquely to the overall functionality and quality of MEMS mirrors.
Photolithography
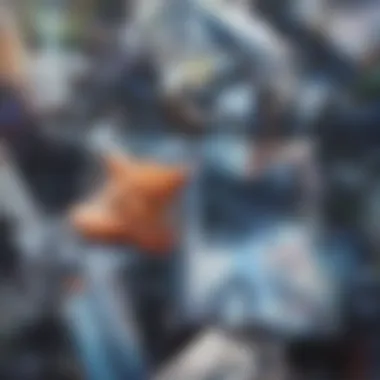
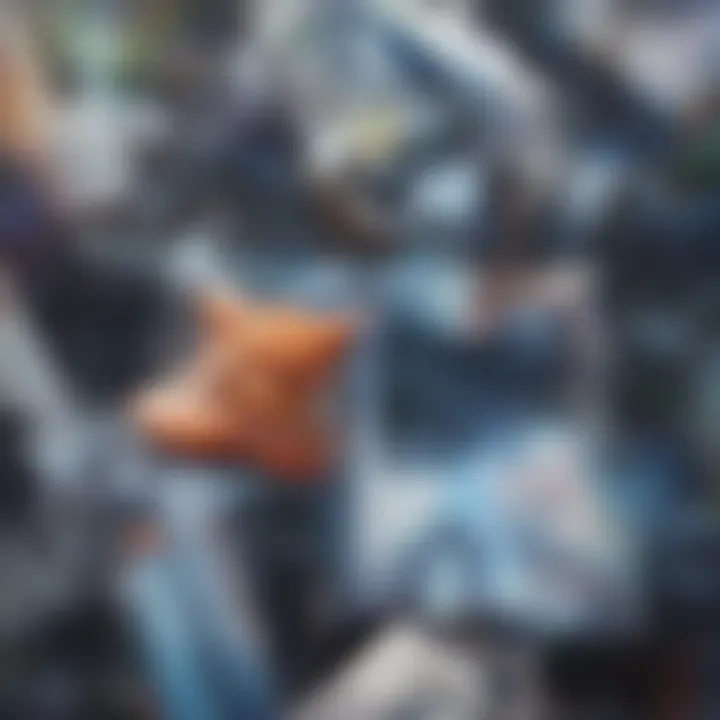
Photolithography is a fundamental process in creating MEMS mirrors. This technique involves transferring a pattern from a photomask to a photoresist material. It is critical for defining intricate structures on the micro-level. The process begins with coating a substrate, typically silicon, with a photoresist layer. Following this, ultraviolet light is used to expose specific areas of the photoresist through the photomask. The exposed areas undergo chemical changes, allowing selective removal of either the exposed or unexposed regions during the developing stage.
The precision offered by photolithography enables the production of microscale features with high fidelity and uniformity. Its ability to create complex geometries is essential in optimizing the performance of MEMS mirrors. Additionally, photolithography is adaptable to various materials, making it a popular choice in the fabrication of MEMS devices.
Etching and Deposition Processes
Etching and deposition are two interconnected processes that shape the actual MEMS mirror structures after the photolithographic step. They facilitate the removal of unwanted material and the addition of new layers, respectively.
Dry Etching
Dry etching involves the use of gases to etch away material in a controlled manner. This method is specific in targeting the design patterns without affecting surrounding areas. One key characteristic of dry etching is its high precision, which allows for a straight vertical profile in etched features, crucial for accurate optical and mechanical performance.
Dry etching is favored due to its ability to create complex patterns with minimal damage to the substrate and film. The technique can also be used to etch materials other than silicon, making it quite versatile. However, it may have limitations concerning surface roughness, which can be less favorable compared to wet etching. Nevertheless, its controlled process makes it a beneficial choice in precision applications for MEMS mirrors.
Wet Etching
Wet etching is a less controlled process than dry etching but is still widely used in MEMS fabrication. This method utilizes chemical solutions to dissolve material on the surface. One key characteristic of wet etching is its isotropic nature, meaning it etches uniformly in all directions. This property can be advantageous in some applications where uniform removal of material is necessary.
However, wet etching tends to create more undercuts, which could be problematic for precision applications. It is essential to choose the right chemicals and concentrations to ensure the etching process is effective while minimizing undesirable effects. Wet etching is often simpler and less expensive compared to its dry counterpart, making it a practical option in specific contexts.
"The choice between dry and wet etching significantly affects the performance and durability of MEMS mirrors."
In summary, both dry and wet etching techniques, alongside photolithography, are fundamental to the fabrication of MEMS mirrors. Their unique characteristics and advantages play a vital role in ensuring the quality and functionality of these sophisticated optical devices.
Performance Metrics
Understanding performance metrics is essential to evaluating the utility and effectiveness of MEMS mirrors in various applications. These metrics offer quantifiable data that reflects the performance and reliability of the mirrors. As MEMS technology evolves, performance metrics become pivotal in differentiating products and technologies in a highly competitive market.
Reflectivity and Optical Quality
Reflectivity is a primary performance metric for MEMS mirrors, directly influencing the optical quality of systems utilizing these devices. A higher reflectivity coefficient translates to better light transmission and overall imaging performance. MEMS mirrors manipulate light through microfabricated surfaces, and maintaining high reflectivity is crucial.
Optical quality is assessed through several factors, including surface roughness and the extent of scattering losses in the mirror design. This reflects the coherence of light and can significantly impact systems reliant on precision imaging, such as medical imaging devices or telecommunications equipment. Effective methods like advanced coating techniques can enhance reflectivity and achieve higher optical quality. The significance of these factors cannot be overstated, as they are fundamental for applications such as sensors and instruments that demand precision.
Switching Speed and Response Time
Switching speed and response time are other critical performance metrics for MEMS mirrors. These characteristics measure how quickly a mirror can move from one position to another. This rapid response is vital for applications like optical switching in telecommunications, where delays can lead to data loss or degradation of service.
Performance can be quantified in milliseconds, and optimizations in design, such as actuator modifications, can improve these metrics. A quick Switching speed enhances system responsiveness, providing seamless operation in dynamic conditions. Therefore, engineers focus intensely on optimizing these metrics to ensure devices meet the rigorous demands of modern technological environments.
Thermal Stability
Thermal stability is crucial for the long-term performance of MEMS mirrors. Fluctuations in temperature can lead to distortion and drift in mirror alignment, affecting both reflectivity and response time. Stability under varying thermal conditions is vital because applications often operate in diverse environmental settings.
Materials such as silicon or specialized polymers can improve thermal stability. An analysis of a mirror's performance through varying temperature ranges can indicate its reliability and durability, especially in sectors like automotive and aerospace where high-temperature resistance is essential. Consequently, understanding and engineering for thermal stability enables MEMS mirrors to perform effectively in challenging conditions.
In summary, performance metrics like reflectivity, switching speed, and thermal stability are integral to the functionality of MEMS mirrors, significantly influencing their applicability across diverse industries. Understanding these metrics provides insights into the design and optimization of MEMS technologies.
Applications of MEMS Mirrors
The applications of MEMS mirrors reveal their versatility and importance in various technological sectors. MEMS mirrors, due to their compact size and efficient operation, are paving the way for innovative solutions across telecommunications, medical imaging, and consumer electronics. Each application showcases specific characteristics and benefits that underscore the role of MEMS technology in modern advances.
Telecommunications Sector
Switching in Optical Networks
Switching in optical networks is a critical function enabled by MEMS mirrors. This technology allows for rapid reconfiguring of optical connections, essential for handling the data demands of high-speed networks. The ability to switch light signals quickly and accurately gives MEMS mirrors a distinct advantage in maintaining robust connectivity and minimizing latency in communication systems.
A key characteristic of switching in optical networks is its agility. MEMS mirrors facilitate dynamic routing of signals, adapting to changing network conditions with ease. This adaptability makes them a popular choice for service providers looking to enhance their network efficiency.
The unique feature of MEMS-based switches is their minimal power consumption compared to traditional electronic circuits. This energy efficiency can lead to reduced operational costs and lower environmental impact for telecommunication companies. However, challenges remain, such as the need for high reliability under fluctuating conditions.
Beam Steering in Free Space Optics
Beam steering technology plays a significant role in free space optics, where light transmission occurs through the atmosphere rather than through optical fibers. MEMS mirrors provide precise control of beam direction, which is crucial in applications like laser communications and surveillance. The ability to steer beams accurately enhances signal integrity and expands the operational range of optical systems.
A notable characteristic of beam steering using MEMS mirrors is their rapid response time. This allows for real-time adjustments and is beneficial in dynamic environments where conditions fluctuate. The versatility of these mirrors enables applications ranging from directed energy systems to advanced imaging setups.
Moreover, the compact nature of MEMS mirrors means that they can be easily integrated into existing systems, making them a favored solution in the optics community. Despite these advantages, factors such as cost and integration complexity can pose challenges when adopting this technology.
Medical Imaging Technologies
Endoscopic Visualization
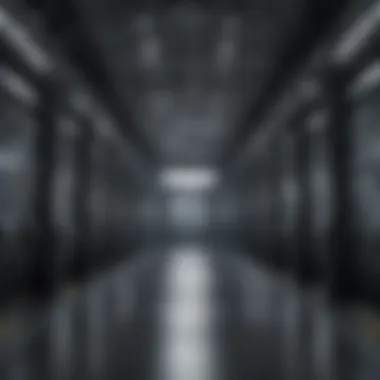
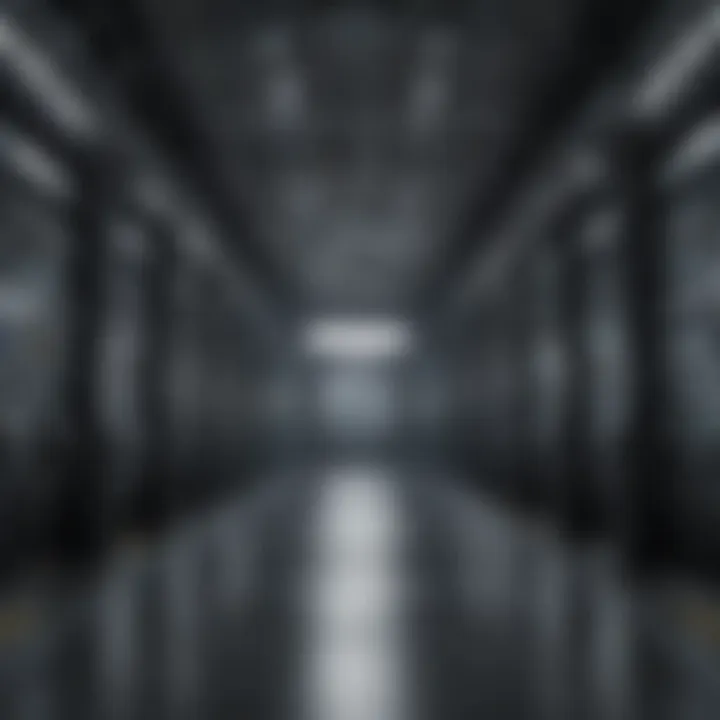
Endoscopic visualization represents a major advancement in medical imaging, allowing for less invasive examinations of internal body structures. MEMS mirrors enhance endoscopes' functionality by enabling high-resolution imaging and improved maneuverability. This technology allows physicians to obtain clearer images while navigating complex anatomical pathways more effectively.
The efficiency and precision of MEMS mirrors in endoscopic visualization contribute to their rising popularity. The small size of MEMS components leads to lighter instruments, ultimately benefiting patient comfort during procedures.
Furthermore, the ability to integrate multiple MEMS mirrors into a single device can create versatile imaging systems. One disadvantage, however, is the high demand for miniaturization and the associated manufacturing complexities that could hinder broader implementation.
Optical Coherence Tomography
Optical coherence tomography (OCT) is another critical application of MEMS mirrors in medical imaging. This non-invasive imaging technique provides detailed cross-sectional images of biological tissues, making it invaluable in ophthalmology and other areas of medicine. MEMS mirrors allow for rapid scanning and improved resolution, which enhances the overall diagnostic capabilities of OCT systems.
A key feature of OCT utilizing MEMS mirrors is its ability to deliver real-time imaging. This is paramount in clinical settings where timely information can greatly influence patient outcomes. Moreover, as accessibility to advanced imaging technologies increases, MEMS mirrors are becoming integral in routine medical examinations.
On the downside, ensuring the reliability and stability of MEMS components in the medical environment presents ongoing challenges. Variations in temperature and humidity can affect performance, necessitating further research and development.
Consumer Electronics
Projectors and Displays
MEMS mirrors are revolutionizing projectors and displays, as they contribute to higher brightness and better image quality in compact formats. These mirrors facilitate the micro-projection of images, making them ideal for small and mobile display devices. The incorporation of MEMS mirrors in projectors allows for a wider range of applications from home entertainment to professional presentations.
A characteristic advantage of using MEMS mirrors in projectors is their ability to create dynamic visuals without significant size increases. This compactness allows manufacturers to integrate advanced projection capabilities into devices that are thin and lightweight.
The unique feature of MEMS projectors is their versatility in image projection. They can easily switch between different display modes and formats, catering to diverse user needs. However, the challenge remains in maintaining affordability while ensuring high-quality output, which is vital for consumer electronics.
Smartphone Cameras
Smartphone cameras are increasingly becoming sophisticated, and MEMS mirrors play a critical role in this evolution. By enabling features such as autofocus and optical zoom, MEMS mirrors enhance imaging capabilities without compromising the overall device size. This progress aligns with consumer demand for high-quality images on mobile devices.
One key characteristic of MEMS technology in smartphone cameras is rapid focus adjustment. This allows users to capture clear images quickly, essential for fast-paced snapping situations. Additionally, MEMS mirrors enable more compact designs, preserving the elegance of smartphones while enhancing their functionality.
Nevertheless, MEMS integration in smartphone cameras poses challenges related to manufacturing costs and durability. The technology must withstand the wear and tear of daily use, requiring continual innovation to improve reliability.
Recent Innovations
Recent innovations in MEMS mirror technology are foundational to its evolution and expansion in various fields. These advancements not only improve the functionality of MEMS mirrors but also broaden their applications across several industries. The two prominent areas of innovation include advancements in materials and enhanced actuation mechanisms.
Advancements in Materials
Nanomaterials
Nanomaterials bring a unique advantage to the structural design of MEMS mirrors. They exhibit extraordinary properties, such as high strength-to-weight ratio and superior optical characteristics. One specific aspect of nanomaterials is their ability to be engineered at a molecular level, allowing for precise control over their physical and chemical properties. This flexibility makes them a desirable choice for improving the performance of MEMS mirrors.
A key characteristic of nanomaterials is their scalability. This allows manufacturers to optimize the materials for various applications, from biomedical devices to advanced imaging systems. One unique feature of nanomaterials is the potential for integration with electric and magnetic fields, which leads to enhanced precision in mirror positioning.
However, the use of nanomaterials is not without challenges. The synthesis and processing of such materials can be complex and costly. Additionally, their long-term reliability under operational stress needs thorough assessment. Nonetheless, the advantages often outweigh the disadvantages, making them a pivotal element in the development of MEMS mirrors today.
Flexible Substrates
The introduction of flexible substrates in MEMS mirror technology provides significant versatility. Flexible substrates are crucial for developing lightweight and portable devices. Their adaptability allows for integration into a wider range of applications that require varying form factors, such as wearable technology and compact imaging systems.
A key characteristic of these substrates is their ability to retain functionality under mechanical stress. This is especially valuable in applications that demand high durability alongside optical precision. One unique feature of flexible substrates is their potential for large-area integration, allowing for a greater number of MEMS devices to be manufactured on a single substrate.
On the downside, the manufacturing process for flexible substrates can be intricate, requiring advanced techniques that may not be universally accessible. Furthermore, maintaining the integrity of optical quality while using flexible substrates can present challenges. Despite these concerns, their benefits in terms of enhanced adaptability and lightweight design make flexible substrates an appealing option for future MEMS mirrors.
Enhanced Actuation Mechanisms
Enhanced actuation mechanisms in MEMS technology significantly boost performance levels. These mechanisms improve the responsiveness and precision of MEMS mirrors, which is crucial for applications such as telecommunications and advanced imaging. The ongoing exploration of different actuation methods, including electromagnetic and thermal actuation, is leading to more efficient designs that allow for quicker adjustments to the light path.
The developments in actuation technology reflect an industry trend towards achieving higher degrees of functionality in compact forms. As these mechanisms continue to evolve, they will play an essential role in expanding the capabilities of MEMS mirrors. The interplay between materials innovation and actuation advancements points to a future where MEMS technology becomes even more integral to various sectors.
Challenges in MEMS Mirror Technology
The development of MEMS mirror technology has brought many advancements. However, there are significant challenges that impact its performance and applicability. Addressing these challenges is vital to ensure the continued growth and reliability of MEMS mirrors in various sectors. This section focuses on two main aspects of those challenges: manufacturing limitations and performance issues.
Manufacturing Challenges
Yield Issues
Yield issues in MEMS mirror production refer to the percentage of functional devices produced compared to the total devices fabricated. This aspect is critical as it influences production costs and potential profitability. High yield rates are essential for making MEMS technology commercially viable. A significant characteristic of yield issues is the sensitivity of the fabrication processes to minute defects. These defects can occur during the photolithography or etching stages of manufacturing.
The need for stringent quality control protocols is apparent. A higher yield can result in reduced costs and improved market competitiveness. However, achieving consistent yield rates can be challenging since it requires an intricate balance of various parameters like material properties and processing conditions.
The unique feature of yield issues is its relationship with overall technology adoption. When yield is low, it discourages investment in MEMS mirror technology. This emphasizes the importance of improving production techniques, to enhance both the yield and the overall reliability of MEMS mirrors.
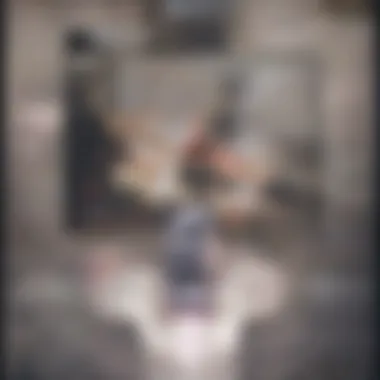
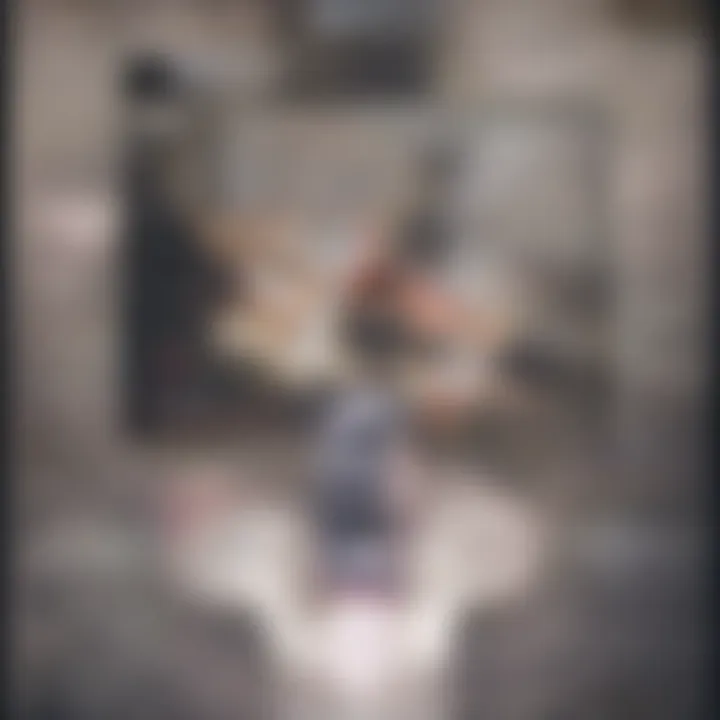
Scalability
Scalability refers to the ability to increase production volume without compromising quality or performance. This element is pivotal in making MEMS technology accessible for widespread use in industries. A key characteristic of scalability is the need for standardized processes that can maintain efficiency at larger scales.
One of the beneficial impacts of scalability is its potential to meet growing market demands. As more industries recognize the benefits of MEMS mirrors, high scalability can lead to faster adoption and integration into products.
However, scalability comes with disadvantages. When adjusting production lines for higher volumes, maintaining quality control can be challenging. Any lapse in quality can affect not just individual products but also damage the credibility of MEMS technology as a whole. Thus, developing scalable production methods is essential for the long-term viability of MEMS mirrors.
Performance Limitations
Reliability Under Varying Conditions
Reliability under varying conditions highlights how MEMS mirrors perform in different environments, such as changes in temperature, humidity, and vibration. This aspect is crucial to understand if MEMS mirrors can be effectively utilized across multiple applications. A key characteristic of this reliability is its direct influence on user trust and product longevity.
Ensuring consistent performance increases user confidence in MEMS technology. When MEMS mirrors demonstrate reliability, they can be deployed in critical applications like medical imaging or telecommunications without concerns regarding failure.
However, varied environmental conditions can affect the performance of MEMS mirrors. The unique feature of this reliability is its dependency on material selection and design. Advanced materials can improve resilience, but they may increase production costs. Therefore, striking a balance between performance and cost continues to be a challenge in the MEMS mirror technology landscape.
Fatigue Over Time
Fatigue over time refers to the gradual loss of performance in MEMS mirrors due to continuous operation. This issue can severely impact their longevity and operational efficiency. A significant characteristic of this fatigue is the wear that occurs in the moving parts, which can lead to degradation of performance.
Understanding fatigue is beneficial for any long-term application of MEMS mirrors. Creating designs that mitigate this issue can extend the lifespan of these devices.
The unique feature of fatigue over time highlights the necessity for rigorous testing and quality assessments throughout the product lifecycle. Manufacturers must focus on developing solutions to reduce wear effects, but this often involves high costs and complex engineering tasks.
Addressing these challenges is not only about improving technology but also about enhancing the overall value delivered by MEMS mirrors in the market.
Future Directions
The exploration of future directions in MEMS mirrors is critical for understanding the ongoing innovations and implications within the field. As technology develops, new applications emerge, highlighting the need for adaptability and growth. This section specifically addresses emerging trends and potential market growth.
Emerging Trends in MEMS Research
Adaptive Optics
Adaptive optics represents a significant evolution in optical systems, characterized by its ability to correct distortions in real time. This technology leverages MEMS mirrors to adjust the phase of light waves dynamically. The importance of adaptive optics lies in its application in various fields, including astronomy and ophthalmology, where precise image quality is essential. For MEMS technology, the adaptability allows it to respond to changing conditions, enhancing performance.
The key characteristic of adaptive optics is its feedback mechanism, which continually evaluates and corrects optical wavefronts. This responsiveness is advantageous as it provides clearer images by counteracting aberrations. However, a potential drawback is the complexity in implementation, requiring sophisticated algorithms and constant calibration.
Integration with Artificial Intelligence
Integrating artificial intelligence with MEMS mirror technology opens new frontiers in automation and precision. AI systems can analyze vast amounts of data to optimize mirror positioning and functionality. This incorporation allows for smarter systems that can predict necessary adjustments based on environmental conditions or operational demands.
The main advantage of using AI is the enhanced efficiency and reduced human intervention. MEMS mirrors integrated with AI can autonomously adapt to changes, making them increasingly valuable in applications like autonomous vehicles and advanced imaging systems. However, reliance on AI may lead to vulnerabilities, such as system failures if the AI makes incorrect predictions.
Potential Market Growth
Industry Forecasts
Industry forecasts indicate a robust growth trajectory for MEMS mirror technologies. This growth is driven by increasing demand in sectors such as telecommunications and consumer electronics. Market analysts predict that advancements in manufacturing processes and materials will continue to expand the application of MEMS mirrors.
An essential characteristic of industry forecasts is their reliance on data analytics to project trends. Accurate forecasts allow stakeholders to make informed decisions regarding investments and resource allocation. The unique feature of these forecasts is their ability to assess the impact of global trends on local industries, which is valuable for strategic planning.
Investment Opportunities
Investment opportunities in MEMS mirror technology are expanding, prompting interest from various sectors. As demand grows for applications like smart devices and imaging systems, both startups and established companies are seeking to capitalize on this trend.
The significance of these investment opportunities lies in their potential return. Technology advancements promise not only innovation but also profitability in emerging markets. The downside, however, is the inherent risk associated with investing in new technologies, where the market is still developing.
The intersection of innovation and investment in MEMS mirror technology highlights its transformative potential across industries, making it a focal point for future growth.
Finale
The conclusion of this article brings together the various threads discussed throughout, emphasizing the substantial impact of Micro-Electro-Mechanical Systems (MEMS) mirrors on multiple sectors. The importance of MEMS technology is not limited to a single application but is evident across telecommunications, medical imaging, and consumer electronics. Each application showcases the adaptability and efficiency that MEMS mirrors offer, transforming the way these technologies operate and improving end-user experience.
Recap of Key Insights
Reviewing the key insights provides a clear understanding of MEMS mirrors and their dynamics. Significant points include:
- Technological Underpinnings: MEMS mirrors operate based on electrostatic and piezoelectric principles. Their design and fabrication techniques lay the foundation for their successful application in various fields.
- Broad Applications: From telecommunications networks using MEMS mirrors for beam steering, to enhancing medical imaging technologies like optical coherence tomography, the applications are vast and varied, proving the relevance of MEMS in everyday life.
- Recent Innovations: Advancements such as the introduction of nanomaterials and enhanced actuation mechanisms showcase the ongoing evolution of MEMS technology, promising improved performance and new functionalities.
- Challenges Ahead: While potential is significant, challenges such as manufacturing yield and performance limitations still need addressing to fully realize the capabilities of MEMS mirrors.
Implications for Future Research
The exploration of MEMS technology prompts several avenues for future research. Key areas that require attention include:
- Adaptive Optics: Research can focus on the development of MEMS mirrors that incorporate adaptive optics, which would significantly enhance imaging quality across various applications.
- Integration with Artificial Intelligence: Integrating AI may lead to smarter systems capable of real-time adjustments and optimizations, thereby increasing the efficiency and effectiveness of MEMS mirrors in practical applications.
- Market Analysis: Understanding the market dynamics and investments in MEMS technology can guide future innovations and penetration in new sectors.
In summary, the conclusion reflects on the transformative nature of MEMS mirrors, highlighting their contributions and the promising paths that future research may take.