Exploring PCR: Key Principles and Latest Innovations
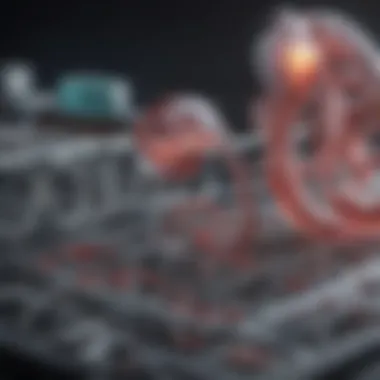
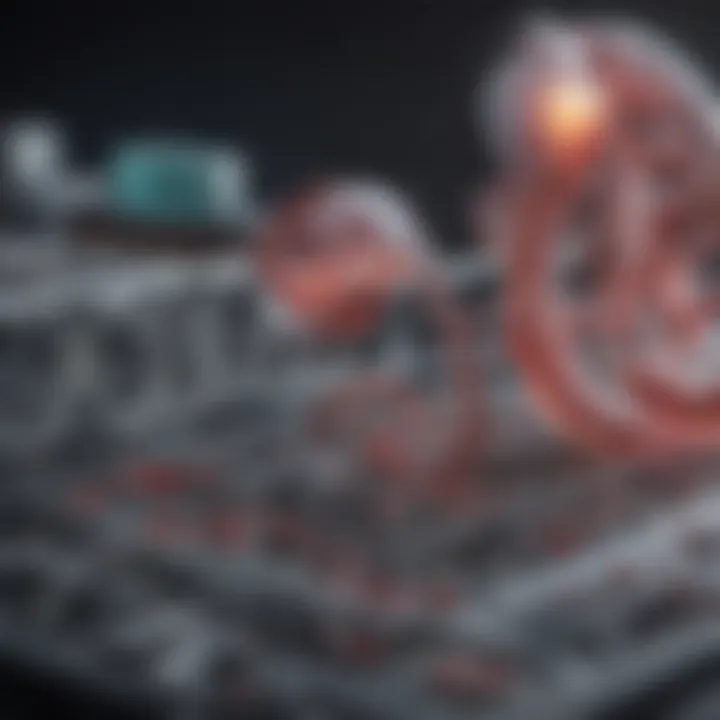
Intro
Polymerase Chain Reaction, often termed PCR, has revolutionized molecular biology since its inception in the 1980s. By enabling the robust amplification of specific DNA sequences, this technique opens doors to countless research avenues and practical applications. Understanding the fundamentals of PCR is not just important for scientists but also vital for students, educators, and professionals across various fields. This guide delves deep into the driving principles behind PCR, its multifaceted applications, and the exciting innovations that continue to progress the technology.
Research Methodology
To grasp PCR fully, it is essential to understand its underlying methodology—how researchers design experiments and the materials they utilize. This section lays the groundwork for appreciating the complex dance of enzymes and nucleotides that occurs during PCR.
Description of Research Design and Approach
The research design associated with PCR typically involves a systematic approach aimed at testing hypotheses within a controlled environment. Often, this engages the use of:
- Primers: Short DNA fragments that initiate the amplification process.
- DNA Template: The strand from which copies need to be made.
- Polymerases: Enzymes that synthesize new DNA strands.
In practical terms, scientists begin by selecting a target DNA sequence. They then design primers complementary to the sequences flanking that target. The entire process generally undergoes a series of cycles involving denaturation, annealing, and elongation, each playing a pivotal role in generating multiple copies of the desired DNA.
Materials and Methods Used in the Study
In performing PCR effectively, certain materials are non-negotiable. Key components include:
- Thermal Cycler: An essential device that automates the temperature changes needed for PCR.
- Nucleotide Mix: Contains the building blocks (dATP, dCTP, dGTP, and dTTP) for new DNA strand formation.
- Buffer Solutions: Maintain optimal conditions for the enzymes involved in the reaction.
Moreover, researchers often leverage specialized reagents such as DNA polymerases suited for particular applications. These could include high-fidelity enzymes for research that requires precision or hot-start polymerases that enhance specificity by preventing non-specific amplifications.
Understanding these methodologies equips readers with insights into how PCR operates at the molecular level, laying the foundation for its applications.
Discussion and Interpretation
As we examine PCR's results and techniques, it becomes clear that this method has vast implications on both existing literature and future research pathways. Here’s how we interpret the findings associated with PCR’s utility.
Interpretation of Results in the Context of Existing Literature
The repercussions of PCR are evident across a range of disciplines. In medical diagnostics, for instance, PCR has dramatically transformed the landscape by providing rapid and accurate detection of pathogens. Studies have illustrated how PCR can pinpoint the presence of viral DNA, a crucial step in managing diseases like HIV and COVID-19.
"PCR is not just a tool; it’s a bridge to understanding the genetic basis of diseases, paving the way for targeted therapies."
Furthermore, in forensic science, its use has revolutionized criminal investigations, enabling the analysis of minute DNA samples that were once unverifiable.
Implications for Future Research or Practical Applications
Given its power, the implications of PCR are vast, opening avenues for:
- Personalized Medicine: Tailoring treatments based on individual genetic makeup that PCR can elucidate.
- Genetic Engineering: Enhancing methods in vector design and genome editing.
- Environmental Studies: Monitoring biodiversity through the identification of species from environmental DNA.
The future of PCR technology seems bright. The ongoing innovations—like multiplex PCR and digital PCR—reflect a persistent evolution poised to enhance accuracy and expand applications further.
As we move forward, our grasp of PCR not only denotes a significant chapter in molecular biology but forms a cornerstone of modern scientific inquiry and advancement.
Prolusion to PCR
Polymerase Chain Reaction, commonly known as PCR, has changed the very fabric of molecular biology and genetics. It allows researchers to amplify specific segments of DNA with unparalleled accuracy and efficiency. Not only does this technology facilitate the exploration of genetic material, but it also becomes a cornerstone for numerous applications across diagnostics, forensic science, and agricultural biotechnology. Understanding PCR is essential for any student, researcher, or educator in the life sciences, as it lays the groundwork for innovations that continually reshape our approach to biology.
Historical Context
The journey of PCR began in the mid-1980s, where in the hands of Kary Mullis, a chemist working in the biotechnology sector, the initial spark was ignited. Mullis had a vision to simplify the process of DNA amplification, which was a considerable challenge at the time. Before PCR, scientists relied heavily on cloning methods which could be time-consuming and inefficient. With Mullis's insight into using cyclic temperature changes and a heat-stable enzyme called Taq polymerase, the first PCR experiment was conducted in 1985. This pivotal moment marked the beginning of a technology that would revolutionize genetic research and diagnostics. A few years later, Mullis received the Nobel Prize in Chemistry for his groundbreaking work, showcasing the immense significance of PCR in science.
Definition and Overview
At its core, Polymerase Chain Reaction is a technique used to create millions of copies of a specific DNA sequence from a small initial sample. The beauty of PCR lies in its simplicity; it often consists of just a few ingredients: the template DNA, primers that flank the target region, Taq polymerase to synthesize new DNA strands, and nucleotides to build the new DNA.
The process unfolds in three main stages: 1) Denaturation, where the double-stranded DNA melts open to single strands; 2) Annealing, where primers attach to each strand; and 3) Extension, where Taq polymerase adds complementary nucleotides to extend the DNA strands. Together, these steps repeat through multiple cycles, leading to exponential amplification of the target DNA.
This method's multifaceted applications make it a staple in modern research. From genetic cloning to clinical diagnostics, PCR paves the way for scientists to delve deep into genetic information, bringing forth new discoveries and technologies.
"Polymerase Chain Reaction is not just a method; it’s a revolution that paved the road for a new era in molecular biology."
The impact of PCR on fields such as medicine, forensics, and environmental science cannot be overstated. Understanding how it works and its applications allows one to appreciate its role as a fundamental tool in the molecular biologist's toolkit. As we delve deeper into the science and various techniques of PCR, the intricacies and potential of this technology will unravel in exciting ways.
The Science Behind PCR
The discussion around the science of PCR is pivotal to understanding its profound impact in various scientific domains. This section dives into both the principles that underpin PCR and how its components work in harmony to amplify DNA. A deep comprehension of this topic not only sheds light on the technical aspects but also illuminates the myriad applications that arise from these scientific principles.
Key Components of PCR
Template DNA
Template DNA is the starting material for any PCR reaction. Essentially, it serves as the blueprint from which copies are made. An important characteristic of template DNA is its specificity. Each sample contains distinct sequences that researchers aim to amplify, making the selection of the appropriate template essential. Its uniqueness is beneficial for a range of applications, be it detecting pathogens or studying specific genes in research.
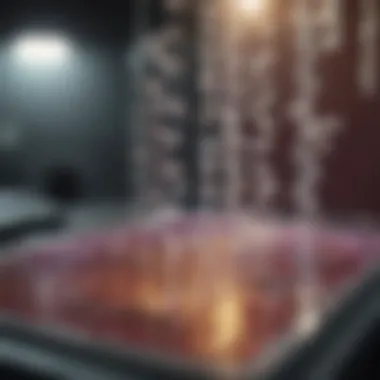
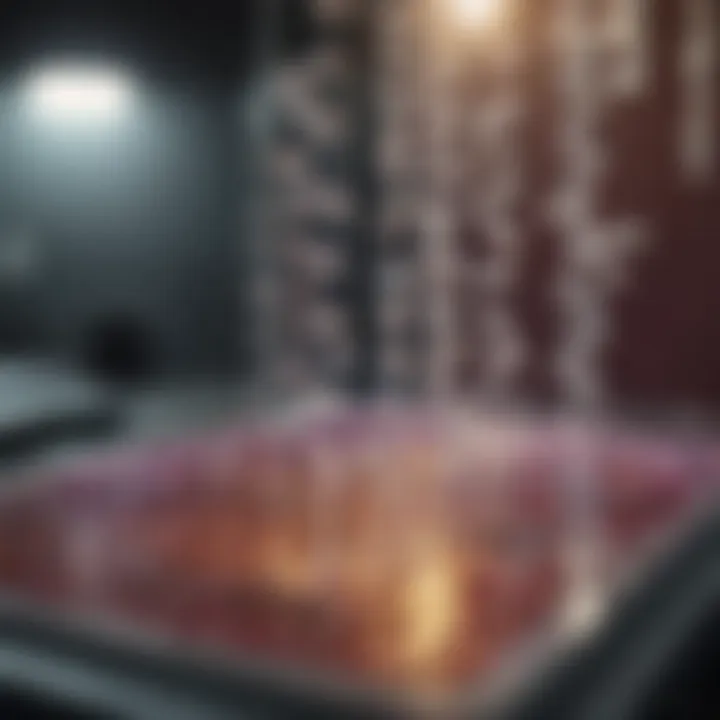
One notable advantage of template DNA is its versatility. It can be isolated from various sources—ranging from blood samples to environmental specimens—allowing for broad applicability across fields including clinical diagnostics and forensic analysis. However, the presence of degraded or compromised DNA may occasionally challenge accurate amplification, underscoring the need for careful handling and preparation.
Primers
Primers are short sequences of nucleotides that initiate the DNA synthesis process during PCR. They are arguably one of the most critical components. The key characteristic of primers is their design: they must match the sequences surrounding the target DNA region accurately. This specificity is fundamental for successful amplification, as mismatched primers can lead to non-specific products.
Primers are popular choices for PCR due to their manufacturability; custom primers can be designed for virtually any target sequence. A unique feature is their ability to guide the polymerase to the correct site on the template. However, the challenge lies in designing primers that avoid secondary structures, which can inhibit the reaction and lead to inefficiency.
Taq Polymerase
Taq Polymerase, derived from the bacterium Thermus aquaticus, is vital for PCR's robustness at high temperatures. Its key characteristic is thermostability, allowing it to endure the denaturation step of PCR, where double-stranded DNA separates into single strands. This property makes Taq Polymerase a popular choice, as it permits many cycles of denaturation and extension without losing activity.
One unique feature of Taq Polymerase is its ability to add a single adenine nucleotide to the ends of newly synthesized strands. This can be advantageous when cloning PCR products. However, a potential downside is that Taq Polymerase lacks proofreading ability, which may lead to errors in amplified sequences. This limitation can be critical, particularly when high fidelity is required.
Nucleotide Mixture
The nucleotide mixture, consisting of adenine, thymine, cytosine, and guanine, is fundamental for building the new DNA strands during PCR. One key component is its balanced ratio; ensuring an equal concentration of each nucleotide is essential for optimal synthesis. This mixture is important because it provides the building blocks required for the polymerase to extend the primers, facilitating the amplification of the target DNA.
A notable feature of the nucleotide mixture is that any deficiency or excess can hinder the reaction's efficiency. Too few nucleotides can lead to incomplete synthesis, while too many can cause a chaotic reaction. Therefore, achieving the right balance is crucial for successful PCR implementation.
Step-by-Step Process
Denaturation
Denaturation is the first step in the PCR cycle, where the double-stranded DNA melts, resulting in two single strands. This process occurs at high temperatures, typically around 94-98°C. The key characteristic of this step is its ability to disrupt hydrogen bonds between the base pairs, effectively opening the DNA for subsequent actions. Undoutbedly, this step is crucial, as it prepares the template for the primer annealing that follows.
One unique feature of denaturation is that it can affect the overall efficacy of the PCR process. If done insufficiently, the DNA may not separate completely, while excessive heating can denature the DNA or the polymerase prematurely. Thus, a careful balance of temperature and timing is necessary to ensure a successful reaction.
Annealing
During the annealing step, the temperature is lowered to allow the primers to bind to their complementary sequences on the single-stranded template DNA. Typically occurring at 50-65°C, this step's effectiveness is largely dictated by the melting temperature of the primers.
The characteristic aspect of annealing is that it sets the stage for amplification. If the temperature is too low, nonspecific binding may occur, while a high temperature may hinder primer attachment. By carefully optimizing this temperature, researchers ensure accurate and efficient amplification of the desired segment of DNA.
One notable point is the duration of the annealing process; it must be adequate for the primers to bind firmly, enhancing specificity yet balancing it with the overall cycle time.
Extension
The final phase involves the polymerase synthesizing new DNA strands complementary to the original template. This occurs at a temperature optimal for Taq Polymerase, usually around 72°C. The key characteristic of the extension step is its speed and fidelity; depending on the length of the target DNA, this step can vary from mere seconds to a few minutes.
A unique feature of this step is its reliance on the previous actions of denaturation and annealing. If templates are not adequately prepared, or if primers fail to anneal properly, extension will likely be compromised. Consequently, the accuracy of this step directly influences the yield and quality of the PCR product, clearly demonstrating how interconnected the steps of PCR are.
Types of PCR Techniques
In the vast landscape of molecular biology, PCR techniques have become indispensable tools, each serving distinct purposes in research and industry. Understanding the various types of PCR methods is crucial, as it offers insights into how these techniques can be effectively utilized for specific applications. In this section, we will delve into several prominent PCR techniques, exploring their unique features, advantages, and considerations.
Real-Time PCR
Real-Time PCR, often known as quantitative PCR (qPCR), is a method that allows for the simultaneous amplification and quantification of DNA. This technique employs fluorescent dyes or probes to measure the amount of DNA generated during the amplification process, with results viewable in real-time.
Importance and Benefits:
- Rapid Results: This methodology provides immediate feedback, making it valuable for time-sensitive studies.
- Quantitative Data: Unlike traditional methods, Real-Time PCR allows for precise quantification of DNA, which is essential in applications like gene expression studies.
- High Sensitivity: The technique is sensitive enough to detect low-abundance targets, which is particularly advantageous in clinical diagnostics, where early detection can be life-saving.
Real-Time PCR has transformed areas like cancer research by enabling the analysis of gene expressions that can serve as biomarkers, connecting laboratory findings with potential clinical applications.
Reverse Transcription PCR
Reverse Transcription PCR (RT-PCR) is a variation that combines reverse transcription and PCR amplification. It is primarily used to analyze RNA sequences. First, RNA is converted into complementary DNA (cDNA) using the enzyme reverse transcriptase. This cDNA is then amplified using standard PCR techniques.
Importance and Benefits:
- Gene Expression Analysis: RT-PCR is crucial for studying gene expressions in various conditions, enabling researchers to assess how genes are regulated over time or under different environmental influences.
- Viral Detection: During viral infections, RT-PCR serves as a powerful tool to identify the presence of RNA viruses in patients.
- Flexibility: This technique can be adapted for high-throughput screening, making it suitable for projects involving large numbers of samples.
By facilitating the study of gene expressions, RT-PCR has opened doors to understanding various diseases, including cancer and genetic disorders, thus enhancing the foundation of personalized medicine.
Quantitative PCR
Quantitative PCR is a refined approach to traditional PCR that focuses on quantifying the amount of DNA present in a sample. While similar to Real-Time PCR, it includes a broader array of methods that can also estimate DNA concentrations at the end of the amplification process.
Importance and Benefits:
- Consistent Results: This method provides reproducible results, which is key in clinical diagnostics and research settings.
- Wide Range of Applications: It's used for pathogen detection, genetic diversity studies, and validating results from other experimental methods.
- Cost-Effectiveness: By enabling quantification in low-cost setups, it offers researchers an accessible means to gather quantitative data without elaborate equipment.
Quantitative PCR has found widespread usage in fields from environmental sciences, where it assists in assessing microbial communities, to agriculture, enhancing the breeding of pest-resistant crops.
Multiplex PCR
Multiplex PCR is a technique that allows the simultaneous amplification of multiple targets in a single PCR reaction. By using multiple sets of primers, each specific to a different target, researchers can analyze several genes or fragments at once.
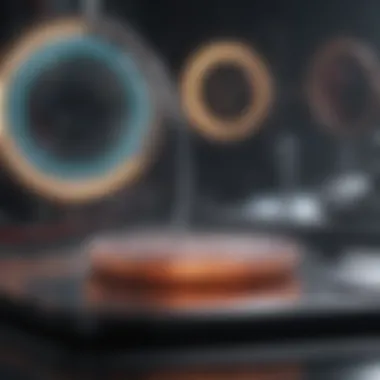
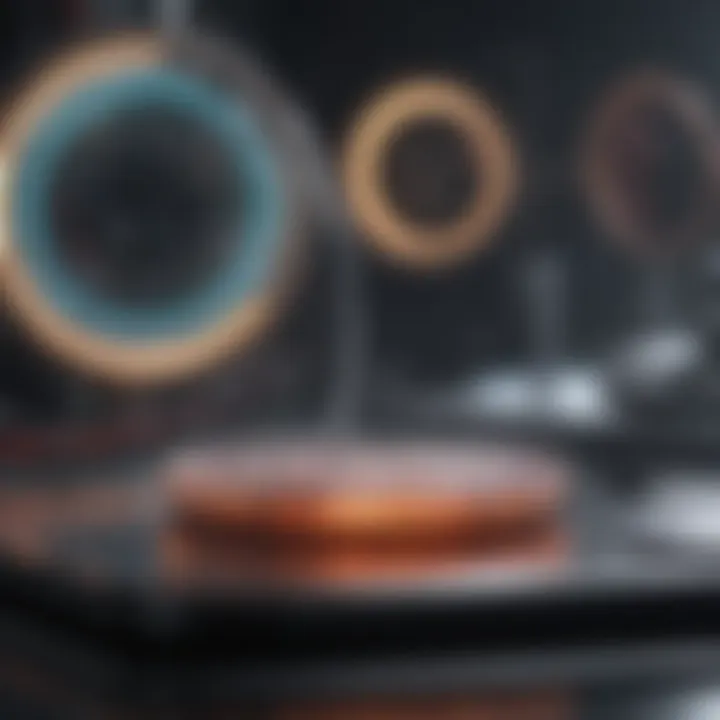
Importance and Benefits:
- Efficiency: Multiplex PCR significantly reduces the time and resources needed for experiments, as multiple targets are assessed in one go.
- Cost Reduction: This technique minimizes the costs associated with reagents and equipment by allowing simultaneous testing.
- Broadened Analytical Scope: It is particularly useful in applications such as clinical diagnostics, where several pathogens or genetic variants may need to be identified from the same sample.
For instance, in the realm of infectious disease testing, Multiplex PCR can assist in identifying different pathogens simultaneously, a critical advancement in managing outbreaks and public health responses.
"Understanding the various PCR techniques empowers researchers to select the appropriate method for their specific objectives, enhancing both efficiency and accuracy in molecular studies."
Applications of PCR
Polymerase Chain Reaction (PCR) serves as a cornerstone in the field of molecular biology, representing a potent technique with multifaceted applications that extend across diverse domains. Its versatility and efficiency are not just buzzwords; they signify a leap forward in how we diagnose diseases, conduct research, solve crimes, and enhance agricultural practices. By amplifying specific DNA sequences with precision, PCR is pivotal for both practical and theoretical advancements in science, making it indispensable in today’s technology-led society.
Clinical Diagnostics
Pathogen Detection
Pathogen detection through PCR has revolutionized clinical diagnostics. This method enables the identification of infectious agents with remarkable speed and accuracy, crucial for patient treatment. One of the key characteristics of pathogen detection is its dramatically shorter turnaround time compared to traditional culturing techniques. While culturing can take days or weeks, PCR can yield results in mere hours, proving its value in emergency medical situations.
A unique feature of pathogen detection is its ability to identify even minute quantities of DNA, allowing it to catch infections at very early stages. This early detection is beneficial because it helps in promptly administering treatment, thus improving patient outcomes. However, the sensitivity of PCR can also be a double-edged sword; it may lead to false positives, where contamination or non-viable pathogen DNA can mislead results. Despite this, its overall impact on patient care is significant and undeniable.
Genetic Disorders
In the realm of genetic disorders, PCR plays a vital role in diagnosing and understanding hereditary conditions. The high specificity of this technique allows for the pinpointing of mutations that may cause various genetic diseases. This is particularly beneficial in pre-natal screening, where expectant parents can be informed about potential genetic risks.
What sets genetic disorder analysis apart is its capacity for multiplexing—simultaneously testing for multiple mutations using a single PCR reaction. This efficiency can save time and resources in clinical settings. That said, the interpretation of these results often requires careful consideration by genetic specialists to avoid misdiagnosis, making it both a powerful and complex option in genetic medicine.
Research Applications
DNA Cloning
DNA cloning is another domain where PCR shines brightly, enabling the amplification of specific DNA fragments to create multiple copies. This characteristic makes it an essential tool in molecular cloning. Researchers can insert cloned genes into vectors for further studies or therapeutic uses, contributing significantly to advancements in genomics.
What makes DNA cloning so advantageous is its ability to simplify the manipulation of genetic material. A unique aspect is that it allows researchers to generate large quantities of specific DNA sequences without the need for host cells, which can often be time-consuming. However, one limitation is that PCR-amplified DNA must undergo subsequent steps for successful integration into vectors, which can introduce challenges related to the overall efficiency of cloning endeavors.
Gene Expression Analysis
Gene expression analysis, facilitated by PCR, provides valuable insights into cellular functions and behavior. By quantifying mRNA levels, researchers can assess how genes respond under various conditions—such as stress, disease, or developmental stages. This capability underscores the significance of PCR in expanding our understanding of biological processes.
The critical characteristic here is the accuracy with which PCR can measure changes in gene expression. Real-time PCR, particularly, allows for the monitoring of these levels in real time, providing dynamic insight into gene activity. However, this can sometimes lead to results that may not fully represent the biological complexities of gene regulation, necessitating a cautious approach when interpreting data.
Forensic Science
DNA Profiling
DNA profiling is a standout application of PCR in forensic science. It enables law enforcement to analyze biological samples from crime scenes to identify suspects or victims. A key characteristic of DNA profiling is its capability to provide highly discriminatory results, even from degraded samples. This is vital when working with evidence that might not be pristine.
What distinguishes DNA profiling is its reliance on specific loci that are known to vary greatly among individuals. This specificity ensures a high degree of accuracy in matching biological samples. The downside, however, is the potential for ethical concerns, especially regarding privacy and ownership of genetic information. Balancing the advantages of PCR in solving crimes with these ethical considerations is an ongoing dialogue in the field of forensics.
Evidence Analysis
In the context of evidence analysis, PCR offers investigative teams the ability to amplify DNA from trace quantities found at crime scenes. This can include hair, saliva, or even skin cells. The ability to work with these small sample sizes is a game-changer for many cases, providing leads that would otherwise be missed.
A unique strength of evidence analysis through PCR is its capacity to elucidate complex mixtures of DNA, which often occurs in scenarios involving multiple contributors. Despite its strengths, the analysis can be intricate, requiring significant interpretative skills to discern the origins of mixed DNA profiles. This complexity adds a layer of challenge to the already demanding nature of forensic work.
Agricultural Biotechnology
GM Crop Development
In agricultural biotechnology, GM crop development stands out as a key application of PCR. This technique allows for the precise modification of genetic material in crops, aiming to enhance desirable traits such as pest resistance, nutrient content, and yield. The ability to specifically target genes for modification can lead to crops that better withstand environmental stresses.
One of the notable benefits of PCR in this context is its efficiency in screening and characterizing transgenic plants, giving researchers quicker insights into the impact of genetic alterations. However, the technology used can spark substantial debate about food safety and biodiversity, placing ethical considerations at the forefront of GM crop discussions.
Plant Breeding Techniques
PCR's role in plant breeding extends beyond GMOs to assist in traditional breeding programs as well. It allows breeders to identify and select plants with desired genetic traits more effectively. This characteristic not only accelerates the breeding process but can also significantly improve crop resilience and adaptability.
Nonetheless, while PCR enhances precision in breeding, there are challenges. It requires a solid understanding of genetics, and misapplications can lead to unintended consequences in plants. Therefore, while the benefits are considerable, the breeding process remains a careful balance of science and nature, demanding thorough research and ethics where necessary.
Recent Advancements in PCR Technology
Recent advancements in PCR technology have shifted the paradigm of molecular biology, allowing for enhanced precision and broader applications. Staying at the forefront of scientific development is crucial because the relevance of PCR extends well beyond mere amplification of DNA sequences. The enhancements in this arena come loaded with benefits, from increased sensitivity to multi-faceted applications, bringing new life into old methodologies and opening doors that were previously shut.
Step-Field PCR
Step-Field PCR ushers in a fresh approach to how we view thermocycling. Traditional PCR, although effective, often grappled with limitations such as temperature inconsistencies and time consumption. Step-Field PCR introduces a clever way to mitigate these issues by utilizing electric fields, which propel the movement of DNA molecules during the process.
- Mechanism: Unlike its predecessor, this technique segments segments of the cycling process into stages. This segmentation not only enhances efficiency but also increases the specificity of primers binding.
- Benefits:
- Rapid Processing: The process can finish significantly quicker due to the reduction of the time spent in each cycle.
- Greater Yield: Users often report higher yields of amplified DNA, which can make a significant difference in downstream applications, especially when starting with scant amounts of template.
- Reduced Contamination Potential: With shorter cycles and more controlled conditions, the chances of contamination via exogenous DNA reduce substantially.
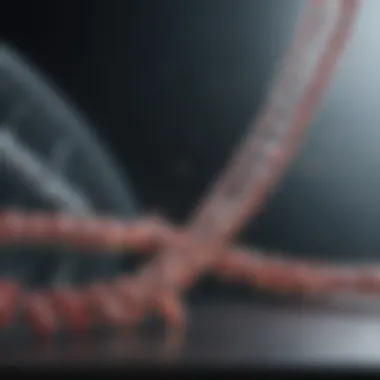
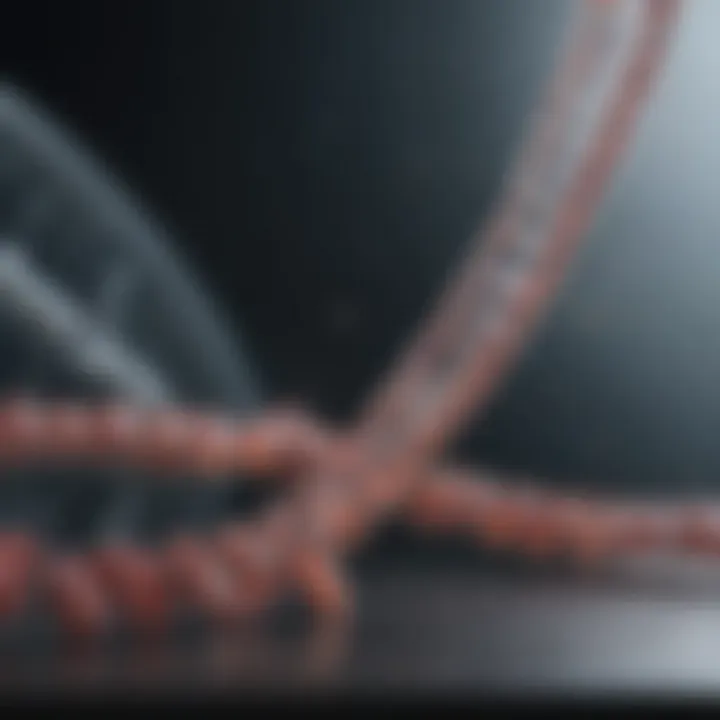
"Advancements such as Step-Field PCR exemplify how creativity can breathe life into established techniques, allowing researchers to extend the boundaries of what’s possible in diagnostics and research."
Digital PCR
Digital PCR takes a bold leap from conventional methods by employing a partitioning strategy. This allows for absolute quantification of nucleic acid targets, a game-changer in its essence. Through this technique, biologists can get precise measurements without the need for standard curves that traditional quantitative methods often require.
- Working Principle: Here, the sample is segregated into numerous smaller partitions, each subjected to PCR. By quantifying the number of positive reactions within these partitions, one can derive the exact concentration of the DNA target in the original sample effectively.
- Significance:
- Sensitivity: This method dramatically enhances sensitivity, enabling the detection of rare mutations in pooled samples, critical in cancer diagnostics or pathogen detection.
- Reproducibility: The digital approach reduces variability often encountered in traditional quantification methodologies, making results more reliable.
- Application Diversity: From clinical diagnostics to environmental monitoring, the versatility of digital PCR extends its usability into realms not traditionally associated with PCR, such as quality control in manufacture processes or pathogen checks in food safety.
The recent advancements in PCR technology not only refine existing practices but also encourage explorations into innovative realms. As scientists continue to push the envelope, PCR stands ready to evolve dynamically, reflecting the needs and challenges of modern molecular biology.
Challenges and Limitations of PCR
Polymerase Chain Reaction (PCR) has transformed the landscape of molecular biology, providing researchers with tools that have remarkably enhanced our ability to study genetic material. However, despite its vast potential and widespread applications, PCR is not without its challenges and limitations. Understanding these barriers is vital for any practitioner in the field, as it allows for the identification of practical hurdles in experimental design and interpretation.
Contamination Issues
One of the most significant hurdles faced during PCR is contamination. The very nature of the technique, which amplifies extremely small DNA samples, makes it incredibly sensitive to any foreign DNA that may inadvertently be introduced to the reaction mix. This sensitivity is a double-edged sword. On one hand, it enables detection of trace amounts of nucleic acids; on the other hand, it also raises the risk of false positives due to the presence of contaminating materials.
To mitigate contamination risks, several practical measures can be implemented:
- Dedicated workspace: Allocate specific areas for pre- and post-PCR processes to minimize cross-contamination risk.
- Use of barrier pipette tips: These can help prevent aerosols from transferring unwanted materials into reactions.
- Regular cleaning of surfaces: Employing detergents and UV light can significantly reduce the presence of extraneous DNA.
In real-world scenarios, contamination has caused significant setbacks. For example, during the testing processes for pathogens, contamination could lead to mistaken diagnoses that not only waste resources but could also have dire health consequences. A precautionary approach is therefore essential to uphold the integrity of the PCR results.
Sensitivity and Specificity Concerns
While PCR's sensitivity is often touted as one of its greatest advantages, it is also a source of concern regarding the specificity of the results. High sensitivity implies that PCR can detect even the slightest signal, but this can sometimes be misleading. The challenge is ensuring that the amplified product is indeed what researchers want to identify, rather than a nonspecific product that could appear due to suboptimal conditions or primer design.
Two main factors contribute to the concern of sensitivity and specificity in PCR:
- Primer Design: If the primers are not designed carefully, they may bind to similar, but non-target sequences, leading to the amplification of unintended products.
- Cycle Numbers: During the amplification, if too many cycles are executed, there is a higher likelihood for non-target sequences to be amplified alongside the actual target.
Researchers often employ various strategies to enhance specificity:
- Optimization of annealing temperatures: Finding the right temperature can improve binding accuracy of primers to the target DNA.
- Using a hot-start PCR method: This helps prevent the formation of non-specific products. In hot-start PCR, DNA polymerase is activated only at higher temperatures, thus reducing the chances of unspecific amplifications during setup.
In summary, while PCR remains a powerful tool in genetic research and diagnostics, the awareness and strategies for overcoming its limitations are crucial. Understanding contamination issues and sensitivity-specificity balances not only improves experimental outcomes but also fosters greater trust in the results yielded by PCR methodologies.
"Overcoming the barriers to PCR's effectiveness is essential for harnessing its true potential in scientific exploration."
By delving into these challenges, researchers can refine their approaches, ensuring accurate and reliable results in their work.
Future Prospects of PCR
The future of Polymerase Chain Reaction (PCR) technology holds significant promise, offering a glimpse into the enhancements that might shape molecular biology and diagnostics in the coming years. PCR has already become a corner store of genetic research, but its evolution suggests that we have just scratched the surface of its potential.
One key area of importance is how innovative applications are emerging, pushing the boundaries of what PCR can achieve. As scientists and researchers seek to solve complex biological puzzles, PCR is set to become an even more reliable tool. Among its many potential applications, using PCR for rapid diagnostics stands out. Imagine a world where infectious diseases can be identified within hours, rather than the traditional sharp-focus lens of days. For instance, in remote areas with limited access to laboratory facilities, enhanced PCR technology could lead to quicker identification of pathogens, thus enabling targeted treatments and potentially saving lives.
Innovative Applications
The innovative applications of PCR are manifold, reaching far beyond the familiar realms of diagnostics and research. In agriculture, for instance, scientists are using PCR to accelerate the development of pest-resistant crops. This technique allows for the rapid screening of plants for desirable traits, significantly reducing the time frame compared to traditional breeding methods.
Moreover, environmental scientists are making strides by employing PCR to monitor biodiversity. By detecting trace amounts of DNA from various organisms in a given environment, researchers can assess the presence of endangered species or track invasive populations with remarkable accuracy. This kind of monitoring can lead to more focused conservation efforts and a deeper understanding of ecosystem health.
In another layer of innovation, PCR is also getting a makeover with the introduction of CRISPR technology. This combination could amplify the precision in gene editing, allowing researchers not just to identify, but also to manipulate genetic material with unparalleled finesse.
Integration with Next-Generation Sequencing
The integration of PCR with next-generation sequencing (NGS) is an area garnering enormous focus. NGS has revolutionized genomics by enabling high-throughput sequencing of vast amounts of DNA. However, it relies on efficient library preparation, where PCR plays a critical role. The synergy between these two technologies can enhance the quality and quantity of data obtained from genomic studies.
By streamlining the PCR step, researchers can achieve higher fidelity in sequencing results. The ability to amplify targeted regions of DNA before sequencing not only increases the efficiency of the process but also reduces costs.
Furthermore, the merging of these technologies has implications for metagenomics, allowing for a comprehensive analysis of complex microbial communities. This can furnish scientists with insights into microbial diversity and its impact on human health and the environment. As an example, one could conceive a future where the personalized treatment of diseases, tailored to a patient's microbiome, could become standard practice.
"While PCR has given us the tools to unlock the secrets of DNA, its future may very well redefine how we understand biology itself."
As we look forward, it is critical to foster research and development in this field to fully harness PCR's potential, ensuring it remains at the forefront of scientific innovation.
Finale
In wrapping up this exploration of PCR, it's vital to underscore not just its mechanics but the profound impact it holds across various domains. The polymerase chain reaction is more than a laboratory technique; it's a cornerstone of modern molecular biology that has revolutionized diagnostics, research, and even forensic science. By understanding PCR in detail, we see how it bridges the gap between basic science and its practical application in the real world.
Summary of Key Points
- Mechanism: PCR employs thermal cycling to amplify target DNA sequences, relying on components like template DNA, primers, and Taq polymerase.
- Diverse Applications: From clinical diagnostics in pathogen detection to innovative gene editing and environmental monitoring, the applications are as vast as they are significant.
- Recent Advancements: Innovations such as digital PCR and step-field PCR offer increased precision and sensitivity, tailoring PCR to meet the demands of advanced research.
- Challenges: Issues like contamination and specificity remain concerns, urging continual assessment and adaptation of techniques used in PCR.
Call to Explore Further
The potential of PCR is continually expanding, inviting keen minds to delve deeper into its intricacies. Those interested in molecular biology should not only stay updated with emerging techniques but also engage with broader discussions around gene editing and diagnostics. Here are a few avenues to further investigate:
- Current Research Trends: Following journals like the Journal of Molecular Diagnostics can provide insights into cutting-edge applications of PCR.
- Online Communities: Platforms like reddit.com host discussions that can widen understanding and application-related inquiries.
- Networking Opportunities: Attend workshops and seminars focusing on PCR advancements to connect with professionals and researchers passionate about the topic.
The field of molecular biology is ripe for exploration, and a deeper understanding of PCR lays the groundwork for endless possibilities.