RNA Sequencing and cDNA Libraries: A Deep Dive
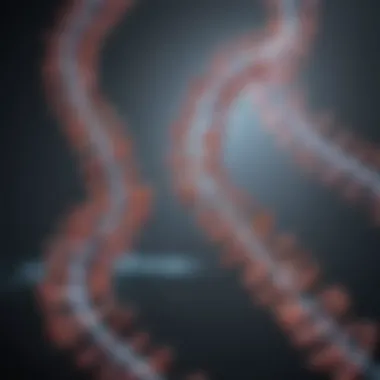
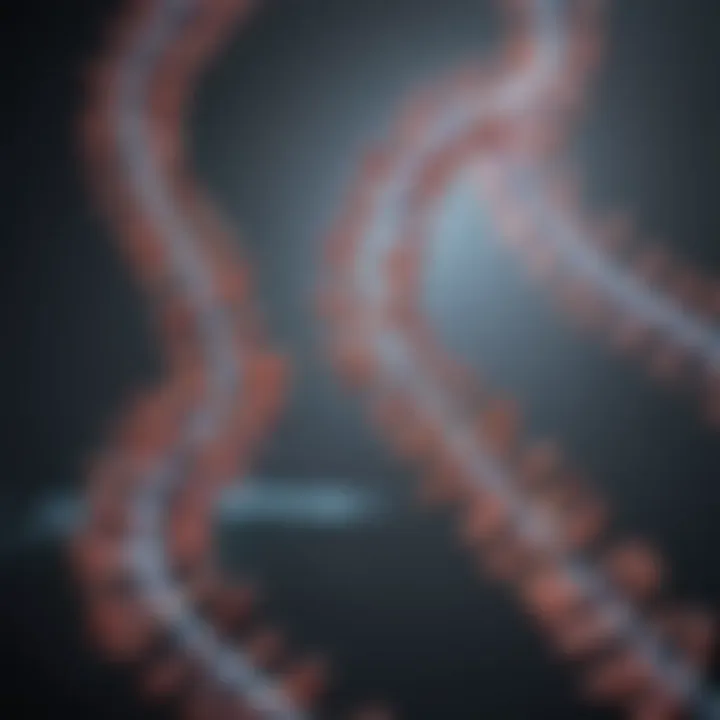
Intro
RNA sequencing and the construction of complementary DNA (cDNA) libraries have become essential techniques in modern molecular biology. These methodologies allow researchers to analyze gene expression at unprecedented depths and resolutions. An understanding of these tools is crucial for anyone involved in biological research, as they contribute significantly to our grasp of genetic information and its implications. This article seeks to provide an enlightening exploration of the techniques, their significance in research, and the diverse applications they offer.
In this discussion, we will delve into various aspects surrounding RNA sequencing and cDNA library techniques. Key points include the research methodologies employed, the materials and methods used in these studies, and the interpretations of results in the context of existing literature. By synthesizing this information, the article aims to equip students, researchers, educators, and professionals with a nuanced understanding of these critical tools in molecular biology.
Preface to RNA Sequencing and cDNA Libraries
RNA sequencing and the construction of complementary DNA (cDNA) libraries form a pivotal component of modern molecular biology. With the capacity to provide intricate details about gene expression patterns, these techniques are essential for understanding the molecular underpinnings of various biological processes. The burgeoning interest in these methodologies correlates with significant advances in technology that have made RNA sequencing more accessible and cost-effective.
In this exploration, we focus on several key aspects of RNA sequencing and cDNA libraries. First, we will discuss the overview of RNA sequencing, which serves as a foundation for this field. Understanding the types of RNA and their roles further contextualizes the importance of these techniques. We will then delve into the significance of cDNA libraries, a critical element in manipulating and studying genetic material. By elucidating these topics, we aim to provide insights that not only highlight the techniques' relevance but also their applications in contemporary research.
Overview of RNA Sequencing
RNA sequencing, or RNA-Seq, involves sequencing the transcriptome of a cell. This includes all the RNA molecules present in a biological sample at a given time. Through this method, researchers can obtain quantitative data on gene expression levels, alternative splicing, and post-transcriptional modifications.
The process typically begins with the extraction of RNA from cells. Next, the RNA is converted into cDNA. High-throughput sequencing technologies are then employed to read the cDNA fragments. The data generated can be analyzed to identify which genes are active, to what extent, and under what conditions. The applications of RNA-Seq are numerous, spanning from basic research to clinical diagnostics, offering a clearer picture of cellular functions.
Significance of cDNA Libraries
cDNA libraries are collections of cDNA clones that represent the mRNAs expressed in a particular cell type or under specific conditions. They serve several important functions in research.
- Gene Isolation: Researchers can isolate specific cDNA clones for studying gene functions, interactions, and regulation.
- Functional Studies: By expressing cDNA in various systems, scientists can examine the effects of specific genes in live organisms or cell cultures.
- Comparative Analysis: cDNA libraries make it easier to compare gene expression across different conditions or treatments.
The utility of cDNA libraries enhances the research landscape and aids in elucidating pathways of interest in various fields such as cancer research and developmental biology.
"cDNA libraries are remarkable tools that facilitate the exploration of gene function and expression, adapting rapidly to the evolving needs of researchers."
In summary, RNA sequencing paired with cDNA library construction offers profound insights that are transforming our understanding of genomics and molecular biology. As we venture deeper into the subsequent sections, the intricacies of these technologies will become clearer, revealing their critical roles and applications.
Understanding RNA: Structure and Function
Understanding RNA is essential for grasping its fundamental role in the processes that govern gene expression and cellular function. RNA molecules are dynamic and diverse, participating in various biological roles that extend beyond mere messengers in the central dogma of molecular biology. A deep dive into RNA's structure and function illuminates the critical places it holds in molecular biology and how it influences gene regulation and protein synthesis.
Types of RNA
mRNA
Messenger RNA (mRNA) is pivotal in conveying genetic information from DNA to the ribosome, where proteins are synthesized. The unique characteristic of mRNA is its role as a template for translation, carrying the genetic code necessary for protein assembly. What makes mRNA particularly beneficial for this discussion is its ability to be quantified and analyzed during RNA sequencing, allowing researchers to investigate gene expression levels directly. The relatively short lifespan of mRNA in cells presents an advantage in real-time studies, as it can provide insights into dynamic biological processes.
tRNA
Transfer RNA (tRNA) serves as the adaptor molecule, matching specific amino acids to the corresponding codons in the mRNA during translation. Its key characteristic lies in its structure, which includes an anticodon that base-pairs with mRNA codons, ensuring proper translation fidelity. The benefits of understanding tRNA in this context include its role in regulating translation speed and accuracy. However, tRNA's relatively stable presence in cells might limit its direct application in some RNA sequencing techniques due to its less variable expression in comparison to other RNA types.
rRNA
Ribosomal RNA (rRNA) is a fundamental component of ribosomes, the cellular machinery responsible for protein synthesis. Its primary role is to provide structural support and catalyze the formation of peptide bonds between amino acids. The significance of rRNA in RNA-seq arises from its vast abundance in cells, making it an excellent target for studying overall ribosomal activity and its relationship with mRNA translation. However, due to its consistent presence in all cells, rRNA may not yield the diverse insights needed for specific gene expression analysis.
Non-coding RNA
Non-coding RNAs (ncRNA) are a diverse class of RNA molecules that do not translate into proteins. They play crucial roles in gene regulation, chromatin remodeling, and RNA processing. Their key characteristic is that they remain underappreciated in many traditional RNA studies, often overshadowed by protein-coding genes. However, understanding their contributions to regulatory pathways can enhance our knowledge of cellular processes and disease mechanisms. The disadvantages of studying non-coding RNA involve their complex expression patterns and functions, which require more sophisticated analytical approaches in RNA sequencing studies.
Role of RNA in Gene Expression
RNA plays a vital role in the transcription and translation processes, orchestrating the flow of genetic information. It influences several stages of gene expression, from the initial transcription of DNA to the eventual synthesis of proteins. Understanding these roles is critical for unraveling the complexities of biological systems and for developing new therapeutic strategies. In specific scenarios such as disease diagnostics or treatment guidance, being able to assess mRNA, tRNA, rRNA, and non-coding RNA contributions can lead to innovative discoveries in molecular biology.
"RNA is not only a messenger but also a master regulator, guiding the intricate dance of cellular functions."
The Basics of cDNA Synthesis
The synthesis of complementary DNA (cDNA) is a pivotal step in the process of molecular biology, particularly in the study of gene expression. cDNA serves as a crucial intermediate in the conversion of RNA to DNA, facilitating various applications in research and diagnostics. Understanding the basic principles and methods of cDNA synthesis is essential for researchers and practitioners in genomics, transcriptomics, and related fields. Each step in the cDNA synthesis process is designed to ensure the integrity and quality of the resulting cDNA, which directly influences the outcomes of downstream applications, including sequencing and cloning.
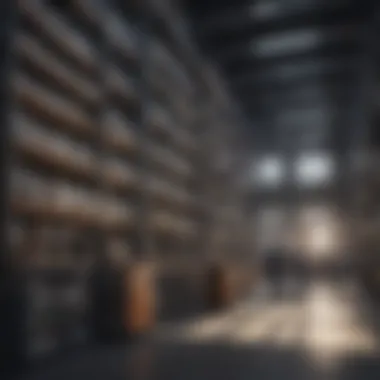
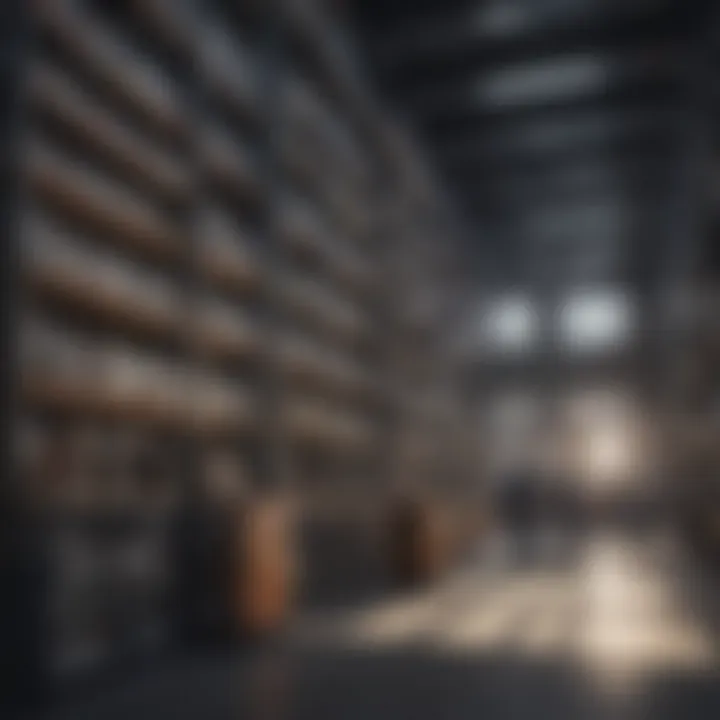
Reverse Transcription Process
The reverse transcription process is the initial phase in cDNA synthesis. This involves the enzyme reverse transcriptase, which catalyzes the conversion of RNA into complementary DNA. During this process, RNA serves as the template while nucleotides are added to form a complementary strand of DNA. The specificity of reverse transcriptase is key, as it determines how efficiently cDNA is synthesized from the RNA template. This step requires careful optimization, including considerations of temperature, buffer composition, and the concentration of reactants to achieve optimal yields. Moreover, factors such as the secondary structure of the RNA molecule may affect the efficiency of reverse transcription, underlining the importance of protocol adjustment based on the specific RNA species involved.
Selection of RNA Samples
The choice of RNA samples is critical for successful cDNA synthesis. The quality and integrity of the RNA directly impact the reliability of the cDNA generated. Thus, thoughtful selection ensures the utility of the cDNA in subsequent experiments.
Purity and Quality Assessment
When considering purity and quality assessment, it is essential to evaluate RNA for contaminants that can inhibit enzymatic reactions. This includes checking for residual proteins, phenols, or salts that may affect the reverse transcription process. The quantity and quality of RNA can be assessed by spectrophotometry or more advanced techniques such as capillary electrophoresis. High-quality RNA is typically defined by a high RNA integrity number (RIN), which indicates minimal degradation.
The high purity of RNA plays an important role. It enhances the reliability and reproducibility of results in experiments that utilize cDNA libraries. Researchers often choose RNA isolation methods optimized for certain tissue types to ensure maximum quality during assessment. While high-quality RNA is typically more reliable for analysis, it may also be more challenging to obtain from degraded samples. Ultimately, ensuring proper purity and quality is vital in the synthesis process and subsequent applications.
Types of Sample Sources
The type of sample source used for RNA extraction can significantly impact the quality and yield of RNA. Common sources include tissues, cultured cells, and biological fluids. Each source presents unique characteristics that researchers must consider.
For instance, tissues may have higher RNA quantities but can be more challenging to homogenize effectively. In contrast, cultured cells offer easier extraction but may provide less total RNA. Biological fluids, such as blood, can vary widely in their RNA content depending on the target of interest.
Construction of cDNA Libraries
The construction of cDNA libraries plays a significant role in molecular biology and gene expression studies. cDNA libraries are collections of complementary DNA that represents the expressed genes of a specific cell type or tissue. They provide a concise way to analyze mRNA transcriptomes by converting them into stable DNA forms. This specific process is crucial as it allows researchers to clone genes, study their expression, and discover new transcripts, providing insight into cellular functions and dynamics.
Steps in Library Construction
Amplification
Amplification is an essential step in constructing cDNA libraries. It involves increasing the amount of cDNA generated from RNA samples. This increase is necessary to ensure that there is enough material for subsequent analyses. The key characteristic of amplification is that it utilizes polymerase chain reaction (PCR) techniques to achieve this goal. Amplification is a popular choice because it allows for the amplification of specific sequences, increasing the likelihood of capturing rare transcripts during analysis.
However, the unique feature of amplification is also a drawback. While it enhances the quantity of cDNA, it can introduce amplification bias, favoring certain sequences over others. This bias may distort the representation of the mRNA pool, which can influence downstream applications and interpretations.
Normalization
Normalization aims to create a balanced representation of all mRNAs in a library. This process adjusts the abundance of different cDNA molecules to minimize variations caused by differences in transcript levels in the original RNA samples. A crucial aspect of normalization is that it allows for a more accurate comparison of gene expression levels across samples.
Normalization is beneficial because it can reduce bias against low-expressed genes. It ensures that all relevant transcripts are available for analysis. However, the unique feature here is that normalization methods can be complex and may not perfectly equalize all cDNA representations. This could lead to loss of some low-abundance transcripts during the process.
Vector Insertion
Vector insertion is the final step in cDNA library construction. This process involves incorporating the amplified and normalized cDNA into a cloning vector, which is a DNA molecule that can carry foreign DNA inside a host cell. The main contribution of vector insertion is that it allows for the stable maintenance of cDNA. The key characteristic here is that it enables the multiplication of the inserted cDNA in host organisms, transforming them into living witnesses of gene expression.
Vector insertion is a critical part of library construction because it ensures that cDNA is available for cloning and analysis. However, a unique feature of this step is its potential to introduce errors if not performed carefully. If the cDNA does not integrate properly into the vector, or if there is an error during the insertion, it may lead to loss or misrepresentation of the genetic information.
Types of cDNA Libraries
There are primary types of cDNA libraries that researchers utilize based on their objectives and the specific properties of the RNA samples they are working with.
Random cDNA Libraries
Random cDNA libraries involve the creation of cDNA from a diverse set of mRNA transcripts. This approach captures a broad representation of gene expression at a given time. The key characteristic of random cDNA libraries is that they are derived from total RNA without regard to specific transcripts of interest. This makes them a beneficial choice when the aim is to understand the overall gene expression landscape.
The unique feature of random cDNA libraries is their capacity to retrieve a wide range of transcripts, including those less frequently expressed. However, one disadvantage may arise in the depth of representation of high-abundance transcripts, which can sometimes overshadow the results from rare transcripts.
Normalized cDNA Libraries
Normalized cDNA libraries focus on providing a balanced view of gene expressions by reducing the overrepresentation of highly expressed genes. This method allows for a more nuanced understanding of differential gene expression. The key characteristic is that it prioritizes a more representative sample of low-abundance genes alongside the high ones, making it a beneficial approach for specific experimental designs.
The unique feature of normalized cDNA libraries is their potential to uncover low-expressed or absent genes that may hold biological significance. That said, they also come with challenges, such as complexity in normalization protocols and the risk of losing high-abundance cDNA during the process.
RNA Sequencing Technologies
RNA sequencing technologies are pivotal in modern molecular biology. They provide a window into the transcriptome, revealing insights about gene expression and regulation. These tools allow researchers to quantify RNA levels, discover new transcript variants, and assess the overall complexity of RNA populations in a given sample. As the demand for more accurate and comprehensive biological data grows, understanding these technologies is vital for advancing research across various fields.
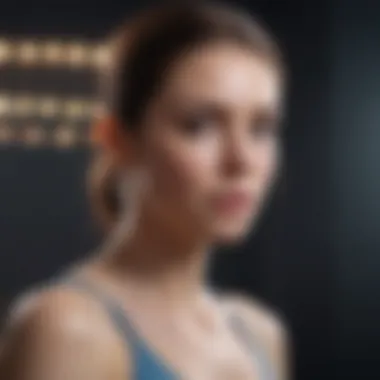
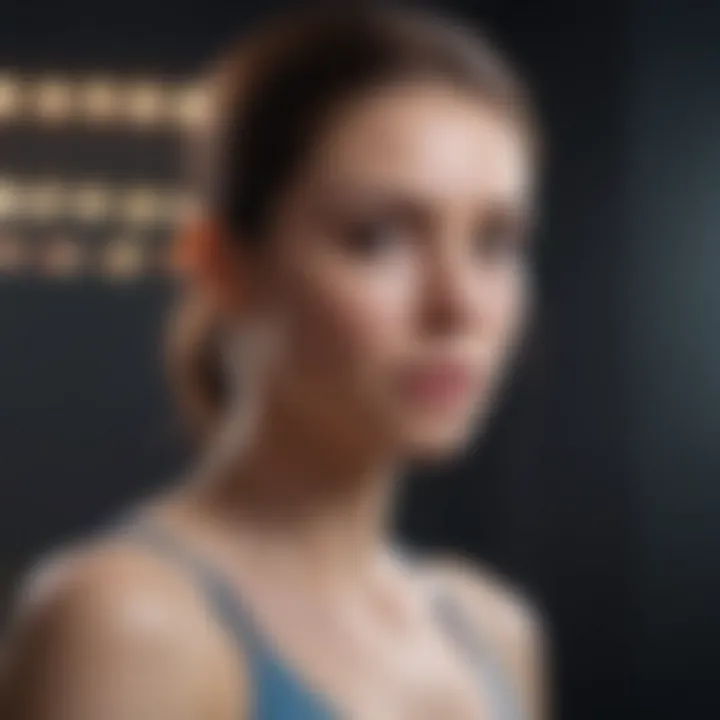
Overview of Sequencing Methods
Sanger Sequencing
Sanger sequencing is one of the oldest methods used for DNA sequencing and has made significant contributions to genomic research. It relies on selective incorporation of chain-terminating dideoxynucleotides during DNA replication. The key characteristic of Sanger sequencing is its high accuracy, which is particularly beneficial for sequencing small regions of DNA or validating results obtained from other methods.
A unique feature of Sanger sequencing is its simplicity and lower throughput compared to more recent technologies. While Sanger is highly reliable, its limitations include longer read lengths and the inability to scale efficiently for high-throughput applications. Consequently, it serves best as a verification tool rather than a primary method in large-scale sequencing projects.
Next-Generation Sequencing
Next-generation sequencing (NGS) has revolutionized the field of genomics by allowing massive parallel sequencing. This technology dramatically increases throughput, enabling the sequencing of millions of fragments simultaneously. The key characteristic that sets NGS apart is its speed and capability to produce large amounts of data quickly, making it an essential tool for transcriptome analysis and genomic projects.
A unique feature of NGS is the ability to generate short reads, which can lead to challenges in assembly and alignment compared to longer reads. However, its advantages include a more cost-effective approach for high-throughput sequencing, making it suitable for extensive studies of RNA expression across various samples.
Third-Generation Sequencing
Third-generation sequencing (TGS) technologies, such as those developed by PacBio and Oxford Nanopore, offer distinct advantages in sequencing long reads, providing even greater insights into complex genomic regions. The notable characteristic of TGS is the ability to capture full-length transcripts, which contributes to a better understanding of transcript isoforms and splicing events.
The unique feature of TGS is its real-time sequencing capability, allowing researchers to monitor the process as it happens. Nevertheless, TGS can be more expensive and has varying accuracy levels compared to NGS. Despite these challenges, it holds promise for enhancing RNA sequencing approaches, particularly in uncovering novel transcripts and studying long non-coding RNAs.
Comparison of Technologies
When comparing RNA sequencing technologies, several factors come into play:
- Accuracy: Sanger sequencing remains unmatched in high accuracy, while NGS offers a balance with excellent throughput. TGS is rapidly improving on accuracy but still has variations.
- Read Length: TGS leads in providing longer reads compared to both Sanger and NGS, making it advantageous for complex transcript analysis.
- Cost: NGS is the most cost-effective for large-scale projects, while Sanger is more economical for smaller experiments. TGS tends to be more expensive but is becoming more accessible.
- Throughput: NGS dominates in throughput and is well-suited for large biological studies, with TGS gaining ground in certain applications.
Each sequencing technology has its strengths and weaknesses, making it crucial for researchers to choose the suitable method based on their specific research needs and budget constraints.
Data Analysis and Interpretation
Data analysis and interpretation hold crucial importance in RNA sequencing and cDNA library techniques. The capability to analyze RNA-Seq data effectively is vital for drawing meaningful biological insights from the complex datasets generated during sequencing. It allows researchers to quantify gene expression, identify differentially expressed genes, and discern underlying biological processes. The process involves a variety of bioinformatics tools and algorithms that are essential in transforming raw sequencing data into interpretable outputs.
To navigate through the data complexities, one needs to understand both the tools available and the inherent challenges in the analysis. Bioinformatics not only empowers researchers to handle large volumes of data but also facilitates the integration of various experimental approaches. This comprehends how RNA-Seq data correlates with other genomic information, enhancing overall research accuracy and relevance.
Bioinformatics Tools for RNA-Seq Data
Alignment Tools
Alignment tools are fundamental in RNA sequencing analysis. They align raw sequencing reads to a reference genome or transcriptome. The primary goal is to accurately map each read to its corresponding location in the reference. A well-known alignment tool is STAR, which is praised for its speed and efficiency in handling large datasets.
A key characteristic of alignment tools is their ability to manage short reads effectively. This capability is essential due to the nature of RNA-Seq, where sequenced fragments can vary in length. The unique feature of these tools includes their algorithms that accommodate gaps and splicing events, which are common in eukaryotic RNA. However, they might struggle with highly repetitive regions, leading to ambiguities in read placement. This can impact downstream analyses if not properly accounted for.
Quantification Algorithms
Quantification algorithms play a significant role in RNA-Seq analysis as they determine the abundance of expressed genes. Tools such as FeatureCounts and HTSeq are commonly used for this purpose. These algorithms evaluate the number of reads that align with each gene, providing a quantitative measure of expression.
A notable characteristic of quantification algorithms is their ability to handle complex datasets. They support various counting approaches, including counts per million (CPM) and transcripts per million (TPM). Their unique features enable standardization across samples, making it easier to compare expression levels. However, they can introduce biases if the assumptions about gene length or read distribution are violated, which researchers should be mindful of during analysis.
Challenges in Data Analysis
Handling Noise
Handling noise in RNA-Seq data is a significant challenge that can distort results. Noise can emerge from various sources, such as technical variations during sequencing or low-quality reads. Addressing noise is essential to ensure reliable conclusions from the expression data.
The most impactful aspect of noise handling is its direct influence on data quality. Techniques such as normalization can help mitigate the effects of noise, ensuring the variability observed in the data truly reflects biological differences rather than technical artifacts. However, the methods used for normalization must be appropriate for the specific dataset at hand, and applying incorrect methods can lead to misinterpretations in gene expression analysis.
Batch Effects
Batch effects refer to systematic errors introduced when samples are processed in groups or batches. These effects may obscure the biological signals of interest. Identifying and correcting batch effects is crucial in ensuring that the data reflects true biological variation rather than artifacts related to the experimental setup.
The significant feature of batch effect management is its impact on the overall integrity of the study. Tools like ComBat and SVA are incorporated to adjust for these discrepancies, enhancing the reliability of findings. Yet, over-correction can lead to loss of genuine biological signals, so it is important to find a balance.
"Accurate data analysis in RNA sequencing is essential for drawing valid biological conclusions. Addressing challenges like noise and batch effects ensures the integrity of research outcomes."
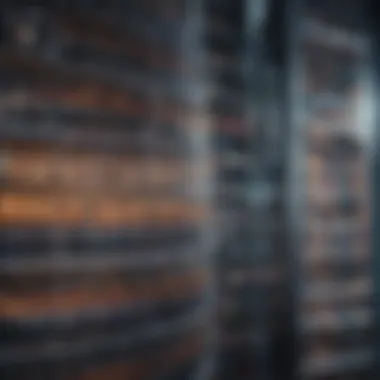
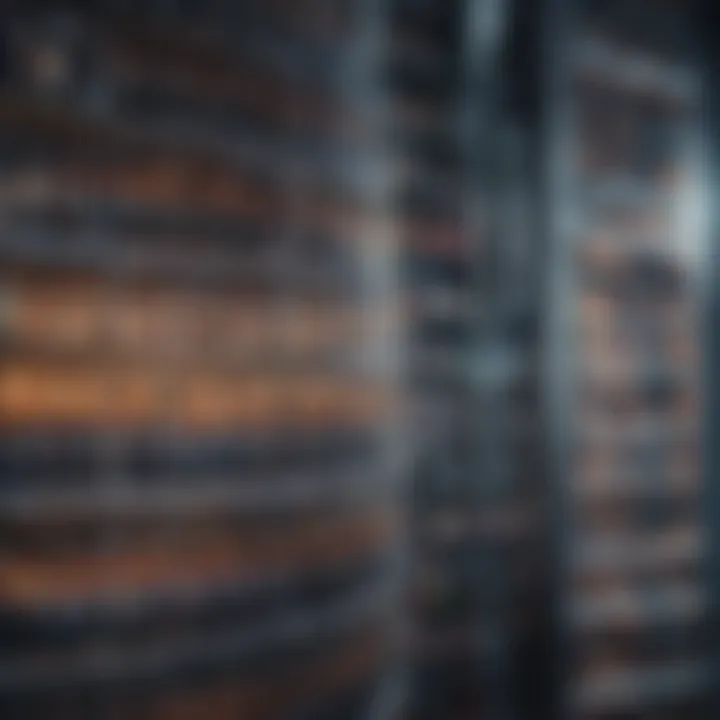
Engaging with these elements of data analysis and interpretation empowers researchers to unlock valuable insights from RNA sequencing. A thorough understanding of the bioinformatics landscape will facilitate the effective exploration of gene expression levels and cis-regulatory elements across various biological contexts.
Applications of RNA Sequencing and cDNA Libraries
The application of RNA sequencing and complementary DNA (cDNA) libraries has opened many new avenues in molecular biology. These technologies have evolved significantly, providing researchers with insights that were difficult to achieve using traditional methods. The ability to analyze gene expression patterns on a transcriptome-wide scale is transformative. RNA sequencing allows for the detection of both known and novel transcripts, making it invaluable in diverse research fields.
One major benefit of RNA sequencing is its sensitivity and accuracy. It enables the detection of low-abundance transcripts that might be overlooked by other methods. This sensitivity is particularly important in applications such as biomarker discovery and precision medicine. cDNA libraries complement these studies by preserving and amplifying expressed sequences, thereby facilitating subsequent experiments like cloning and functional analyses.
However, the use of these technologies also comes with considerations. The complexity of the data generated from RNA sequencing requires advanced bioinformatics tools for analysis. Moreover, careful sample preparation is crucial to avoid biases that can affect the outcome of the data interpretation.
Gene Expression Profiling
Gene expression profiling is one of the most prominent applications of RNA sequencing and cDNA libraries. It provides insights into the dynamic regulation of genes under various conditions. By examining expression levels across different tissues or developmental stages, researchers can identify key regulatory elements and pathways involved in specific biological processes.
Additionally, gene expression profiling can help in understanding the effects of treatments or environmental changes on cellular behavior. For instance, treatments that alter gene expression can serve as indicators of therapeutic efficacy, which is particularly important in drug development. This makes gene expression profiling an essential tool in both basic and applied research.
Disease Research and Diagnostics
RNA sequencing and cDNA libraries significantly contribute to disease research and diagnostics, offering critical insights into the molecular underpinnings of diseases.
Cancer Research
In cancer research, RNA sequencing reveals the complex genetic changes that underpin tumorigenesis. Researchers can identify differentially expressed genes between normal and cancerous tissues, leading to insights into tumor biology. This aspect of cancer research emphasizes how RNA sequencing can highlight specific alterations in the transcriptome that correlate with disease progression or response to therapy.
The ability to identify and characterize these changes in real time allows for personalized treatment strategies, enhancing therapeutic outcomes. It is particularly beneficial for investigating heterogeneous tumors where traditional methods may fail to capture the full spectrum of gene expression.
Infectious Diseases
RNA sequencing has also made significant contributions to the study of infectious diseases. It allows for the identification of pathogen RNA in host tissues and provides detailed profiles of host immune responses. This helps to differentiate between various pathogens and understand how they interact with the host immune system.
A unique feature of this application is its potential to reveal novel viral and bacterial targets. Some pathogens evolve quickly, complicating diagnostics. RNA sequencing can adapt to these changes, presenting a fluid solution to identify infectious agents. Its real-time application can also help inform treatment options, aiding in outbreak management and public health responses.
In summary, the applications of RNA sequencing and cDNA libraries span a wide range of fields, contributing to advancements in gene expression analysis, cancer research, and studies of infectious diseases. The ongoing evolution in these technologies continues to provide new insights that will influence future research paradigms.
Future Directions and Trends
The realm of RNA sequencing and cDNA library techniques continues to undergo rapid evolution. Understanding the future directions in this field is paramount for researchers, educators, and students alike. As technology advances and methodologies are refined, these innovations promise to enhance the capabilities in gene expression studies, diagnostics, and research at large. The ongoing trends indicate not only improvements in existing technologies but also the emergence of novel strategies and approaches that hold great potential for the future.
Emerging Technologies in RNA Sequencing
One of the significant trends in RNA sequencing is the rise of new technologies. These technologies aim to improve accuracy, reduce costs, and offer greater throughput. Recent advancements like single-cell RNA sequencing have transformed how scientists can investigate the complexity of tissues at an individual cell level. This provides a deeper understanding of cellular heterogeneity and gene expression. As researchers continue to adopt these innovative approaches, they will be able to glean insights into the genetic underpinnings of various biological processes and diseases more effectively. The drive towards miniaturization and automation may also result in portable RNA sequencing devices, expanding their use in field studies and point-of-care diagnostics.
Integration with Other Omics Approaches
Genomics
Genomics stands as a crucial complementary field to RNA sequencing. By analyzing the genome, researchers can better contextualize the RNA data obtained from sequencing. Genomics contributes significantly to understanding the functional relationship between genes and their expression levels. The integration of genomics with RNA sequencing also enables the identification of regulatory elements and genetic variations that can impact gene expression and disease susceptibility. This combined approach is increasingly seen as a beneficial strategy for comprehensive biological research, allowing for a holistic view of genetic and transcriptional landscapes. However, it is essential to be mindful of the complexities involved when pairing these two domains.
Proteomics
Proteomics further enriches the landscape of molecular biology by focusing on the protein expressions resulting from RNA transcripts. By understanding how expressed genes translate into proteins, researchers can offer insights into cellular functions and interactions that DNA and RNA analysis alone might miss. The key characteristic of proteomics is its ability to provide data on post-translational modifications and protein-protein interactions, which are vital for assessing cellular processes. This integration allows for a more profound understanding of biological systems but comes with challenges such as dynamic range and the complexity of protein samples, which need to be considered when synthesizing the information from these omics layers.
Culmination
The conclusion of this article synthesizes the vital role that RNA sequencing and cDNA libraries play in contemporary molecular biology. The significance of these techniques cannot be underestimated, as they have revolutionized genetic research and our understanding of gene expression.
Recap of RNA-Seq and cDNA Library Importance
RNA sequencing provides an unparalleled insight into the transcriptome, showcasing not only the expression levels of mRNA but also the complexities of splicing and alternative transcription. cDNA libraries serve as crucial tools for studying these transcripts, allowing researchers to isolate and analyze specific genes of interest. The combination of these technologies enables a more granular understanding of biological processes, paving the way for new discoveries and innovations in fields such as genomics, cancer research, and infectious disease studies.
"The advancement in sequencing technology has unlocked a new avenue for exploring the human genome."
Additionally, the integration of bioinformatics tools enhances data analysis, helping interpret vast amounts of information generated. Thus, understanding the importance of RNA sequencing and cDNA libraries is essential for anyone engaged in biological research. These concurrent analyses foster a deeper grasp of genetic mechanisms and their implications across various paths of inquiry.
Looking Ahead in Molecular Biology
Looking forward, the landscape of molecular biology is set to evolve further with the anticipated advancements in RNA sequencing technologies. Emerging methods promise increased accuracy and higher throughput. This not only expands the capabilities in research but also invites an amalgamation with other omics approaches.
Integrating RNA sequencing with genomics and proteomics could herald a new era in precision medicine, providing a comprehensive overview of cellular functions in health and disease. As researchers continue to refine these techniques, the insights gained from RNA sequencing and cDNA libraries will likely illuminate uncharted territories in biology, enabling targeted therapies and improving diagnostic frameworks.