Exploring Stem Cell Lines: Their Significance and Use
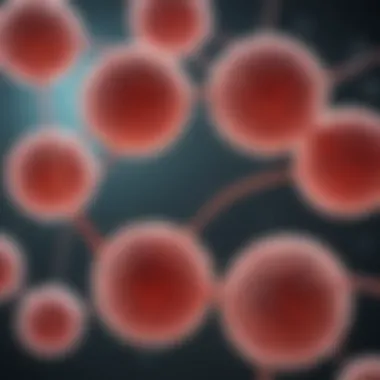
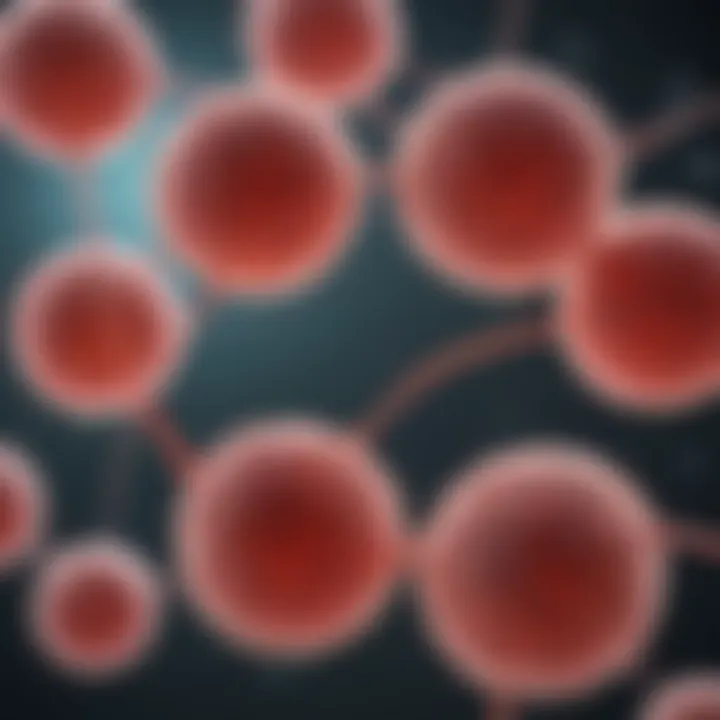
Intro
Stem cell lines represent a cutting-edge frontier in biological research, navigating the sometimes tumultuous waters of scientific innovation and ethical introspection. Their potential is immense, as they can differentiate into various cell types, offering valuable insights into development, disease mechanisms, and possible therapies. Understanding stem cell lines not only illuminates their complexities and varieties but also positions researchers to harness their possibilities for medical advancements.
The significance of exploring stem cell lines such as embryonic, induced pluripotent, and adult stem cells cannot be overstated. Each type serves unique purposes and is derived through different processes, contributing to a broader understanding of their capabilities and limitations. In this journey, we will uncover how researchers develop these lines, discuss the ethical currents that swirl around them, and highlight their critical roles in regenerative medicine and disease modeling.
Research Methodology
In delving into the world of stem cell lines, a structured research methodology is key. This consists of a systematic design and approach that captures the essence of current understanding while opening doors to examine past and future implications.
Description of Research Design and Approach
The research design typically adopts a multi-faceted approach, combining both qualitative and quantitative methods. Qualitative analyses help in understanding the ethical considerations surrounding stem cell usage, while quantitative studies assess the effectiveness of various stem cell types in regenerative applications. By intertwining these methodologies, researchers can draw a clearer picture of the subject.
Materials and Methods Used in the Study
In this exploration of stem cell lines, multiple materials and methods are employed:
- Cell culture equipment for growing and maintaining cell lines.
- Biochemical assays to examine cellular functions such as differentiation and proliferation.
- Gene editing tools, like CRISPR, to manipulate genes within stem cells, enhancing their study.
- Ethical review boards to ensure compliance with regulations surrounding the use of stem cells.
These tools and methods are fundamental in navigating the complexities associated with stem cell research.
Discussion and Interpretation
The implications of stem cell lines are vast, with significant repercussions for both ethical philosophy and scientific advancement.
Interpretation of Results in Context of Existing Literature
When contrasting findings from recent literature, the interpretations reveal a trend toward more personalized medicine. Studies have shown that stem cells can provide tailored treatments for diseases such as diabetes and Parkinsonās by generating specific cell types (like insulin-producing cells or dopamine-producing neurons). This not only highlights the potential impact on patient care but also brings to light varying methodologies across studies.
Implications for Future Research or Practical Applications
The future is ripe for exploration. As researchers gain more proficiency in creating and manipulating stem cell lines, they could uncover novel therapies for previously untreatable conditions. Moreover, the ongoing debates on ethical considerations may necessitate new policies to govern stem cell research. This growing recognition sets the stage for dynamic discussions and advancements in the field.
"The potential of stem cell lines to revolutionize medicine is matched only by the ethical considerations they evoke."
Prelude to Stem Cell Lines
The world of stem cell lines is a complex yet fascinating arena that lies at the intersection of biology, medicine, and ethics. As research in this field progresses, understanding the nuances of stem cell linesāwhat they are, how they are derived, and their implicationsābecomes increasingly essential. The importance of the study of stem cell lines cannot be overstated, especially as they hold promise for revolutionizing therapies and treatments for a variety of conditions.
The significance of stem cell lines in todayās scientific landscape encompasses several key elements. They serve as invaluable tools for disease modeling, enabling researchers to mimic aspects of biological processes and diseases, which can facilitate the testing of new drugs and therapies. Furthermore, these lines are foundational in the development of regenerative medicine, where the potential to replace damaged tissues or cells is on the horizon.
In this section, we will delve into the precise definition of stem cell lines to lay the groundwork for our discussion. Additionally, we will explore their historical context, examining how the understanding and utilization of stem cells have evolved over time to reach the current state of research and application.
Overall, this exploration of stem cell lines is critical for students, researchers, educators, and professionals, providing them with a solid foundation to appreciate the implications and applications of stem cells in modern medicine and biological research.
Definition of Stem Cell Lines
Stem cell lines are populations of cells that originate from stem cells through the process of culturing. These lines can be maintained for an extended period while retaining the characteristics of the original stem cells. In layman's terms, think of a stem cell line as a clone of the original stem cell that has been nurtured and allowed to grow in a lab.
Generally, stem cell lines are classified as either embryonic or adult, each varying distinctly in their properties and applications. Embryonic stem cell lines, for example, have the ability to differentiate into any cell type in the body. On the other hand, adult stem cell lines possess a more limited differentiation potential but are crucial for the maintenance and repair of tissues.
"Stem cell lines provide the foundation for understanding complex biological systems and developing innovative therapies."
A significant advantage of stem cell lines is their ability to be standardized. This standardization allows for reproducibility in experiments, which is vital for scientific integrity. Furthermore, they enable large-scale screenings for drug development. Thus, stem cell lines form a crucial backbone in the realm of biotechnology and medical research.
Historical Context
The story of stem cell research dates back several decades, but major milestones have shaped its current trajectory. In the late 20th century, the isolation of human embryonic stem cells marked a turning point, sparking intense interest and subsequent research into the mechanisms of stem cells.
During the 1980s, researchers at the University of Wisconsin successfully derived the first human embryonic stem cells, which laid the groundwork for future studies. This breakthrough not only enhanced our understanding of developmental biology but also ignited ethical debates that continue to this day. The moral implications surrounding the use of embryonic stem cells remain contentious, influencing policy and funding decisions worldwide.
Later advancements, particularly the development of induced pluripotent stem cells (iPSCs) in 2006, further shifted the landscape. Researchers discovered that by reprogramming somatic cells, they could create pluripotent stem cells similar to embryonic stem cells without the associated ethical dilemmas of using human embryos. This opened new avenues for research and therapeutic applications, ultimately providing greater accessibility to stem cell technology.
Overall, the historical context highlights the dynamic evolution of stem cell lines, underscoring how scientific breakthroughs can reshape our understanding of biology and medicine. The past informs the future, and in the realm of stem cell research, we stand on the threshold of exciting possibilities.
Types of Stem Cells
Stem cell lines are pivotal to advancements in biological research and medical therapies. Understanding the different types of stem cells allows researchers to leverage their unique properties effectively. The primary categories we explore are embryonic stem cells, adult stem cells, and induced pluripotent stem cells. Each type has distinct characteristics, advantages, and ethical considerations that shape their applications in science and medicine.
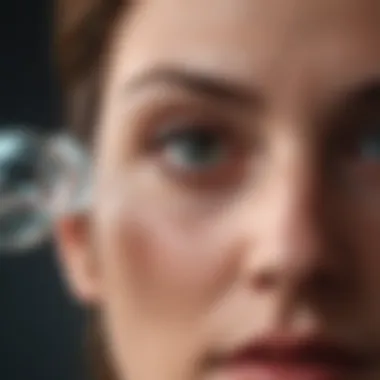
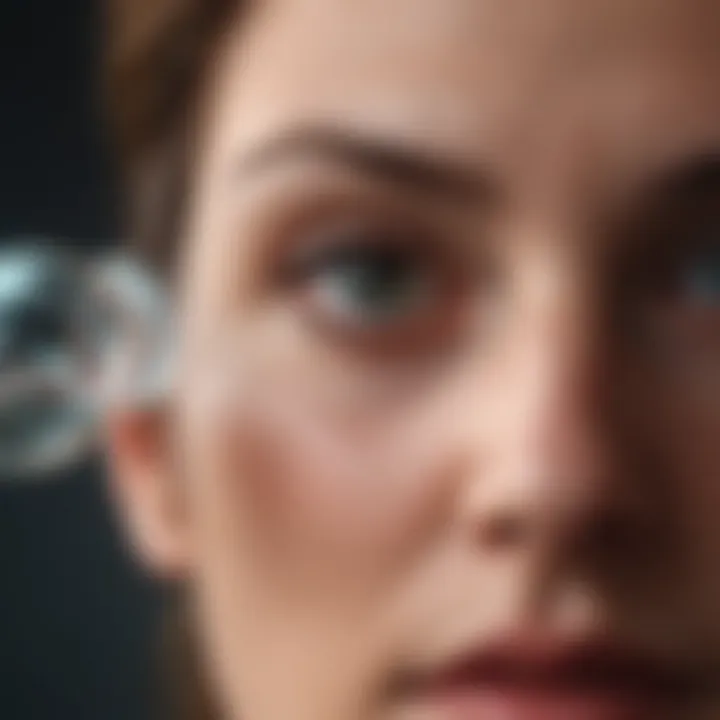
Embryonic Stem Cells
Embryonic stem cells (ESCs) are derived from early-stage embryos, typically from the blastocyst stage, which is around five days post-fertilization. These cells stand out for their pluripotency, meaning they can differentiate into nearly any cell type in the body. This attribute holds immense promise for regenerative therapies, as scientists can potentially create any tissue needed for repair or replacement.
However, working with embryonic stem cells is not without controversy. Ethical concerns stem from the source of these cells, as their extraction often involves the destruction of embryos. This raises significant moral questions, and debates surrounding the use of ESCs can influence funding and research direction. As a result, researchers must navigate these ethical waters carefully while aiming to harness the full potential of embryonic stem cells.
Adult Stem Cells
Adult stem cells, also known as somatic or tissue-specific stem cells, are undifferentiated cells found in various tissues of fully developed organisms. Unlike embryonic cells, adult stem cells are typically multipotent, indicating that they can develop into a limited range of cell types specific to the tissue from which they originate. For instance, hematopoietic stem cells from bone marrow can give rise to various blood cells, which is crucial for treating blood-related diseases.
The use of adult stem cells may offer advantages in terms of ethical acceptability as they can be harvested without harming the donor. Furthermore, adult cells are less likely to lead to tumor formation compared to other types; however, they often have more limited capacity for self-renewal and differentiation. To tap into their regenerative potential, researchers are investigating the mechanisms that govern their behavior and how to enhance their regenerative properties.
Induced Pluripotent Stem Cells
Induced pluripotent stem cells (iPSCs) are a breakthrough in stem cell research, generated by reprogramming somatic cells to regain a pluripotent state akin to that of embryonic stem cells. This innovation allows scientists to create stem cells from adult tissues, thereby sidestepping many ethical concerns associated with embryonic cells. The process typically involves introducing specific genes that are active in embryonic stem cells into the somatic cells, reverting them to an embryonic-like state.
The beauty of iPSCs lies in their versatility and ability to differentiate into any cell type while bypassing ethical dilemmas. These cells open doors for developing patient-specific therapies, as they could be derived from the individual requiring treatment, minimizing rejection risks. Nonetheless, challenges exist, including ensuring the cells do not retain any mutations from their original somatic state, which could lead to unforeseen complications.
Closure
In summary, the exploration of different types of stem cells sheds light on the potential trajectories of stem cell research and therapy. Each type brings its own strengths, limitations, and ethical implications, influencing their application in various biomedical arenas. As research progresses, understanding these types will be paramount to advancing not only our scientific knowledge but also genuine therapeutic options for patients.
The Process of Deriving Stem Cell Lines
The process of deriving stem cell lines lays the groundwork for much of modern biomedical research. Obtaining these cell lines not only requires technical expertise but also a nuanced understanding of ethical considerations and biological implications. This section unpacks the critical steps involved in deriving stem cell lines, highlighting the significance of precise techniques for isolation, skilled culturing methods, and rigorous quality control measures.
Isolation Techniques
Isolation is the first andoften the most crucial step in creating a stem cell line. This process involves separating stem cells from their parent tissue, whether it's an embryo or adult tissue. Various techniques come into play here. The most widely accepted include:
- Mechanical Disaggregation: This involves physically breaking down tissue into smaller parts. While this method is straightforward, it can sometimes lead to the loss of vital cellular characteristics crucial for stem cell identity.
- Enzymatic Digestion: Here, enzymes such as collagenase or trypsin are used to digest extracellular matrices, allowing for smoother extraction of stem cells. This method tends to yield a higher quantity of viable cells compared to mechanical methods alone.
- Fluorescence-Activated Cell Sorting (FACS): This sophisticated technique enables researchers to isolate specific cell types based on unique markers. Although it is expensive and requires precise equipment, it allows for a targeted approach, which is especially useful when working with heterogeneous tissue.
These isolation methods draw attention to the importance of context; the choice of technique can significantly influence the properties of the derived stem cells. In practice, the goal is to obtain cells that maintain their pluripotency, enabling differentiation into various cell types in future applications.
Culturing Methods
Once stem cells are isolated, the next step is culturing them. This process involves providing suitable conditions where cells can grow and thrive. Culturing methods vary, and different environments can lead to distinctive characteristics in stem cells. Hereās a glimpse at the common methods:
- 2D Cultures: This traditional method involves growing stem cells on flat surfaces. While it's cost-effective and easy to manage, it can limit cell interactions and fail to accurately mimic in vivo environments, leading to altered behavior in the cells.
- 3D Spheroid Cultures: This technique attempts to overcome the limitations of 2D by forming cell aggregates that more closely resemble natural tissue structures. It has been shown to promote cell survival and maturation but can be more challenging to control.
- Bioreactors: Advanced methodologies utilize bioreactors to replicate dynamic environments that stem cells would typically encounter in vivo. This method can support large-scale production of stem cells, making it ideal for therapeutic applications.
Each culturing method presents unique benefits and challenges, making it essential for researchers to choose one that aligns with their specific goals.
Quality Control Measures
Quality control in the derivation process is essential to ensure that the stem cell lines produced are authentic, stable, and ready for application in research or therapy. Several measures come into play:
- Genetic Profiling: This involves sequencing DNA to verify the identity and ensure the stability of stem cells over passages. Itās vital for detecting any genetic drift that might occur during culturing.
- Mycoplasma Testing: Contaminants such as mycoplasma can alter cell behavior and affect experimental outcomes. Regular testing is crucial to maintain the integrity of cell lines.
- Differentiation Assays: Conducting experiments to see if cells can differentiate into specific cell types is another critical measure. Successful differentiation indicates that the stem cells maintain their pluripotency and are not drifting away from their original characteristics.
"Quality assurance is not just a box to check; it's the very foundation upon which reliable stem cell research rests."
In summary, the process of deriving stem cell lines is far from simple. It requires a blend of well-chosen techniques, careful cultivation, and steadfast quality control. Understanding these factors enhances not only the significant benefits of stem cells in research but also the ethical considerations that accompany their use. Through careful attention to each stage, researchers can unlock the potential that stem cell lines hold for transformative advances in medicine.
Characteristics of Stem Cell Lines
The characteristics of stem cell lines are truly crucial in shaping the potential and applications of stem cell research. Understanding these defining traits can provide insight into their usability in various fields, particularly in regenerative medicine and disease modeling. These distinctive features give stem cells their remarkable versatility and pave the way for groundbreaking advancements in science and healthcare.
Self-Renewal
Self-renewal is a cornerstone characteristic of stem cell lines. This ability allows stem cells to undergo numerous cycles of cell division while maintaining their undifferentiated state. Essentially, a stem cell can replicate itself indefinitely, which is vital for expanding cell populations for research purposes.
Without this unique trait, the viability of stem cell lines would be significantly compromised. For example, it allows researchers to create ample amounts of stem cells for experimentation, ultimately leading to insights into cellular behaviors and potential therapies. When stem cells demonstrate robust self-renewal capability, they provide a stable source for research and clinical applications, fostering the hope of developing new treatment options for a variety of diseases.
Differentiation Potential
Another paramount characteristic is differentiation potential. This speaks to a stem cell's ability to develop into specialized cell types. Depending on the stem cell type, the range can be extensive. For instance, embryonic stem cells are pluripotent, meaning they can transform into any cell type in the human body. On the other hand, adult stem cells are typically multipotent, meaning they can only develop into a limited range of cell types, usually related to their tissue of origin.
The differentiation potential of stem cell lines is significant for applications in regenerative medicine. If a stem cell can differentiate into the appropriate cell type, it can potentially be used to replace damaged or diseased tissues. For instance, creating neural cells from stem cells holds promise for treating conditions like Parkinson's disease or spinal cord injuries. When considering the therapeutic aspects, the clearer the differentiation pathways are understood, the more effectively stem cells can be utilized in practical applications.
Genetic Stability
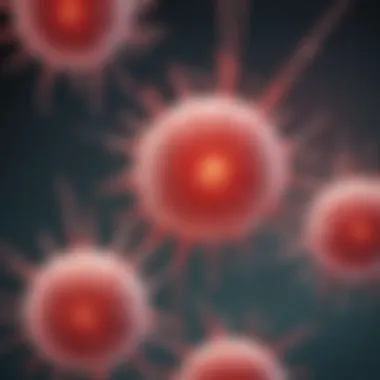
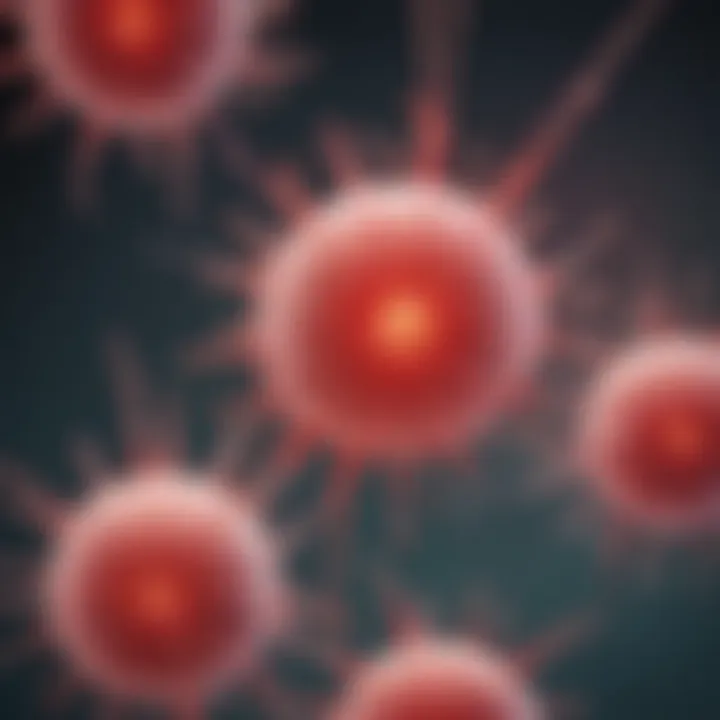
Genetic stability is another major aspect to consider when discussing stem cell lines. This characteristic refers to the ability of stem cells to maintain their genomic integrity over time. It's quite important because any genetic alterations can lead not only to unpredictable behavior but also to potential risks, such as tumorigenesis, which can arise if the cell transforms in unregulated ways.
Ensuring genetic stability involves rigorous quality control measures throughout the stem cell lineās cultivation and expansion. By consistently monitoring for any chromosomal aberrations or mutations, researchers can mitigate risks and confirm that stem cell lines are safe to use. Genetic stability thus becomes a protective factor in advancing stem cell research, enabling researchers to strengthen the foundation for future therapies and applications.
"The intricate nature of stem cell properties, including self-renewal, differentiation potential, and genetic stability, underscores the complexity and promise of stem cell research."
In summary, the characteristics of stem cell lines are fundamental to understanding their potential roles in research and medical advancements. From their remarkable self-renewal capabilities to their functional ability to differentiate into various cell types, coupled with the necessity of maintaining genetic stability, these traits collectively determine how stem cell lines can be harnessed in practical applications and innovative therapies.
Applications of Stem Cell Lines in Research
Stem cell lines represent a remarkable tool in modern scientific research. They not only capture unique biological properties but also provide a window into complex processes of human development and disease. Understanding these applications allows researchers to harness the potential of stem cells, particularly in areas that intersect with health and disease. The core benefits of using stem cell lines in research include, but are not limited to, advancements in disease modeling, drug development, and tissue engineering.
Disease Modeling
One of the foremost applications of stem cell lines is disease modeling. Stem cells can be derived from patients with specific conditions, allowing scientists to create in vitro systems that closely mimic the natural disease environment. For instance, researchers can generate neurons from stem cells obtained from individuals with neurodegenerative diseases like Alzheimerās. This approach enables them to study disease progression in a controlled setting and scrutinize various cellular responses to different stimuli.
Key advantages of using stem cell lines in disease modeling include:
- Personalization: Each stem cell line maintains the genetic characteristics of the donor, which means researchers can investigate patient-specific responses.
- Discovery of Mechanisms: Researchers can look at disease mechanisms more closely at the cellular and molecular levels, leading to potential discoveries of underlying causes.
- Evaluation of Treatments: Scientists can test new drugs or therapies on these models before they proceed to clinical trials, thus ensuring a step that may catch unforeseen issues early.
Drug Development
Drug development is another vital application of stem cell lines in research. The ability to create human cells in the lab enhances the drug discovery process significantly. Stem cell lines provide a relevant biological context that can be used to screen potential drug compounds. For instance, using induced pluripotent stem cells (iPSCs), researchers can model the effects of pharmaceutical agents on specific cell types, such as muscle or heart cells.
The benefits of utilizing stem cell lines in drug development encompass:
- Increased Efficiency: These models help streamline the testing process, eliminating the need for extensive animal testing in the earlier stages.
- Human Relevance: Results obtained from human-derived stem cell lines often yield better predictive value compared to traditional models.
- Toxicity Testing: Researchers can assess potential toxicity and side effects of drugs using stem cell-derived tissues, ultimately improving safety profiles before human trials.
Tissue Engineering
Tissue engineering is a frontier where stem cell lines have shown tremendous promise. The hope is to create functional tissues or organs for transplantation. In this realm, stem cell lines serve as a foundational resource for creating various cell types, which can integrate into engineered tissues.
Some notable aspects of using stem cell lines in tissue engineering include:
- Regeneration of Damaged Tissues: Stem cells can be guided to differentiate into specific cell types that may help repair or replace damaged tissues, offering solutions for injuries or degenerative diseases.
- Customization: They allow for the production of tissues tailored to individual patients, decreasing the risk of rejection after transplantation.
- Research and Development: By studying how stem cells interact within three-dimensional structures, scientists gain insights into natural tissue architecture and function, which can inform better engineering techniques.
"The journey of research in stem cell lines is not merely an academic exercise; it holds profound potential for revolutionizing medical treatment and understanding human biology."
Overall, these applications underscore the significant potential that stem cell lines have in reshaping our approach to many aspects of medicine and biology. With ongoing advancements, the horizon broadens for researchers, driving creativity and innovation in this pivotal field.
Ethical Considerations in Stem Cell Research
When it comes to stem cell research, ethical considerations are not merely an afterthought; theyāre at the very forefront of discussions in the scientific community. The complexity surrounding ethical dilemmas reflects an ongoing dialogue that impacts both the direction of research and public perception. Understanding these considerations is pivotal, as they shape the frameworks within which scientists operate while attempting to balance innovation with morality.
Embryonic Stem Cells and Moral Dilemmas
The use of embryonic stem cells often ignites heated debate. These cells, derived from embryos, hold remarkable potential for regenerative medicine, but their procurement raises significant moral questions. Critics argue that the process of extracting these cells equates to the destruction of potential life. In contrast, supporters promote the idea that advancing medical science could save countless lives, offering solutions to ailments that current treatments cannot tackle.
- Proponentsā Viewpoint:
- Opponentsā Viewpoint:
- Saving lives and improving medical outcomes.
- Potential to address degenerative diseases.
- Ethical concerns about the value of life.
- Alternative sources, such as induced pluripotent stem cells, exist.
This dichotomy creates a moral minefield. In academia and beyond, individuals grapple with weighing the potential benefits against the ethical costs. Whether for personal conviction or institutional policy, the methodology of using embryonic stem cells can invoke discomfort, pushing researchers to consider their implications carefully.
Informed Consent
Informed consent is a cornerstone of ethical research practices. For stem cell studies, obtaining informed consent from donors is essential, ensuring they understand the implications of their contributions. This process not only engenders trust but also enhances the credibility of research endeavors.
Many challenges arise in regard to informed consent:
- Complex Terminology: Donors may not fully grasp the scientific jargon involved.
- Long-term Implications: Participants need to know how their cells will be used, and the potential risks associated with that.
- Right to Withdraw: Ensuring donors know they can change their minds at any time adds another layer of ethical reassurance.
By actively working to ensure that donors are well-informed, researchers can navigate the ethical landscape more effectively, fostering a relationship based on mutual respect and transparency.
Regulations Governing Stem Cell Research
To navigate the ethical tangles associated with stem cell research, regulations are put in place at various levelsālocal, national, and international. These guidelines aim to protect both the participants and the integrity of scientific research itself.
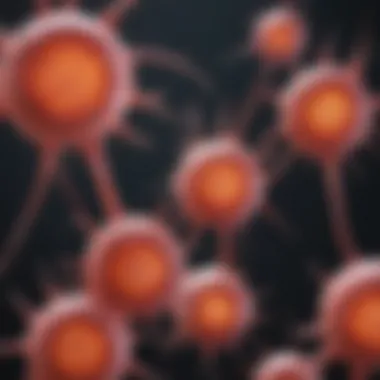
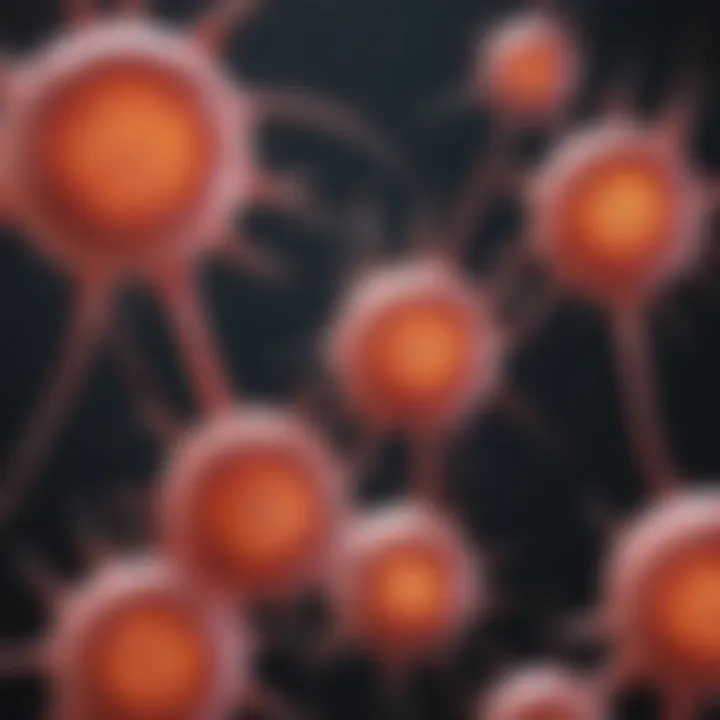
- Examples of Key Regulations:
- The National Institutes of Health (NIH) Guidelines in the United States.
- The European Unionās Charter of Fundamental Rights, emphasizing human dignity.
- The World Health Organization (WHO) recommendations on gene editing and stem cell usage.
Regulations often evolve in response to societal pressures and emerging scientific capabilities. Researchers must keep abreast of these evolving standards to maintain ethical compliance. The regulatory landscape serves as a guiding beacon, directing scholars towards responsible innovation that respects the sanctity of life while fostering scientific advancement.
"Ethics in stem cell research isn't just about compliance; it's about cultivating a culture of respect and responsibility in an era of rapid scientific breakthroughs."
Challenges Facing Stem Cell Lines
Stem cell lines harbor immense potential for transformative research; however, navigating the challenges they face is critical. These hurdles can hinder research progress and influence the practical applications of stem cells in medicine and therapeutics. Understanding these challenges allows researchers and practitioners to devise strategies that mitigate risks and enhance the effectiveness of stem cell therapies and research. Key aspects of these challenges include cell line heterogeneity and the risk of tumorigenesis.
Cell Line Heterogeneity
Cell line heterogeneity refers to the variations within a stem cell population that can arise from genetic, epigenetic, or environmental factors. This diversity is a double-edged sword. On one side, it provides a broader scope for studying different cellular behaviors, responses to treatments, and potential outcomes in regenerative medicine. Yet, on the other hand, it complicates standardization and reproducibility of results.
Different cell lines may exhibit distinct properties from one another, affecting how they grow, differentiate, or respond to specific stimuli. This inconsistency can skew experimental results, leading to difficulties in translating findings into clinical practice. For instance, a specific treatment may work effectively on one stem cell line but fail on another, complicating drug development and testing.
Moreover, heterogeneity may arise during the derivation and expansion of stem cells in culture. It means that experiments conducted in one laboratory may not be easily replicated in another, creating skepticism regarding the reliability of results. Researchers must employ rigorous quality control measures and detailed characterization of their stem cell lines to address these issues and ensure a more robust scientific foundation for future studies.
Risk of Tumorigenesis
The risk of tumorigenesis is a significant concern when using stem cell lines for research and therapeutic applications. Stem cells possess the unique property of unlimited self-renewal, which, while beneficial, can also lead to uncontrolled growth under certain conditions, thereby increasing the risk for tumor formation.
This phenomenon is particularly relevant for embryonic stem cells and induced pluripotent stem cells, which retain the ability to proliferate indefinitely. While their pluripotency allows for extensive applications in research, it also raises alarms regarding the potential for tumor development when these cells are introduced into a patientās body.
When exploring therapies involving stem cells, researchers must conduct thorough assessments and risk evaluations to ensure their safety. This might include limiting the number of cell divisions before transplantation or selecting for more stable cell lines with clearer differentiation profiles. Understanding how to monitor and control these risks is essential to advancing stem cell research responsibly.
"The prospect of fully harnessing the potential of stem cells rests not only on their characteristics but also on our ability to navigate the associated risks effectively."
By addressing these challenges, it's possible to enhance both the reliability and safety of stem cell research. Only through concerted efforts can we unlock the full potential of stem cell lines and their applications in medical science a head.
There is no doubting the importance of overcoming these obstacles as society moves toward a future where stem cell applications are expected to play a pivotal role in curing diseases, regenerating damaged tissues, and improving overall health outcomes.
Future Directions in Stem Cell Research
The realm of stem cell research is brimming with potential, and this section aims to illuminate the key avenues that could redefine medical and scientific landscapes. With rapid advancements in technology, the future seems ever more promising. Researchers are focusing on both practical applications and novel methodologies that amplify the capabilities of stem cell studies. Significant factors include the enhancement of safety standards, improvements in efficiency, and the ethical dimensions that guide future research.
Technological Advancements
Technological progress in stem cell research has skyrocketed in recent years, offering new methodologies and tools. Improved techniques for gene editing, such as CRISPR-Cas9, have become vital. These advancements allow scientists to create custom stem cell lines with specific genetic modifications, paving paths for personalized medicine.
Key Innovations
- In Vitro Models: Advances in organ-on-a-chip technology are allowing researchers to mimic human organs more effectively. This could lead to breakthroughs in drug testing and disease modeling.
- High-Throughput Screening: Enhanced computational tools enable massive screenings of stem cells for potential therapies, drastically cutting down the time and resources needed for research.
- Artificial Intelligence: Integrating AI with stem cell research can streamline data analysis and help predict stem cell behavior much more accurately.
These innovations not only enhance research efficiency but also help in exploring previously challenging areas in disease treatment and regenerative strategies.
Potential for Regenerative Medicine
Regenerative medicine stands at the crossroads of innovation and necessity. Stem cells have long been heralded for their ability to regenerate damaged tissue and offer solutions for chronic illnesses. The future promises a sharper focus on translating these scientific discoveries into real-world therapies that may remarkably improve the quality of life for countless patients.
Emerging Applications
- Tissue Restoration: Regenerative therapies have the potential to create or repair tissues for conditions such as heart disease and spinal cord injuries. Researchers are developing strategies to coalesce stem cells with scaffold materials, essentially growing new organs in the lab.
- Chronic Disease Management: Stem cells could be pivotal in managing diseases like diabetes. The ability to regenerate insulin-producing cells might turn the tide for patients who depend on lifelong treatments.
- Neural Regeneration: Advances in neural stem cell application are showing great promise for treating neurological disorders. Studies are exploring how to regenerate neurons damaged in conditions such as Alzheimerās and Parkinsonās disease.
"The future of regenerative medicine relies heavily on the progress we make with stem cell lines today, and the implications are nothing short of revolutionary."
In summary, the future directions in stem cell research are laden with robust potential fueled by technological advancements and expansive applications in regenerative medicine. By focusing on these pursuits, the scientific community is preparing to tackle health challenges that, just a generation ago, seemed insurmountable.
Epilogue
In summing up, the journey through stem cell lines reveals not just a scientific marvel but a critical linchpin in modern biomedical research. These cell lines, which can self-renew and differentiate into various cell types, bear immense potential that could alter the course of therapies and treatments across multiple medical landscapes. Understanding their implications ensures that we appreciate their role not only in the development of new medical technologies but also in our ethical considerations surrounding human development and research.
Recap of the Importance of Stem Cell Lines
Stem cell lines empower researchers with the tools to crack the codes of diseases, such as cancer and genetic disorders. By utilizing these cells, scientists are capable of creating disease models that mirror actual conditions more accurately. This capability allows for some impressive breakthroughs in drug testing and personalized medicine.
Moreover, they offer insight into human development and the processes that can lead to various malfunctions or diseases. The viability of stem cell lines therefore presents a broader view of cellular functions and mechanisms, catalyzing advancements that are not only groundbreaking but also essential for the continual evolution of medical knowledge.
"Stem cell lines hold the key to understanding complex diseases and engineering solutions that were previously out of reach."
Calls for Continued Research
Despite the advances made, the field of stem cell research is still littered with challenges, raising a clarion call for ongoing exploration. There remains a pressing need to develop more robust techniques for lineage tracing and genetic modifications that minimize the risks associated with tumorigenesis.
Research must also focus on elucidating the ethical implications and regulatory frameworks surrounding stem cell use. Engagement with diverse perspectives will pave the way for a more nuanced understanding of the potential benefits, risks, and societal impacts associated with stem cell technology.