Exploring Stem Cells and Tissue Engineering Innovations
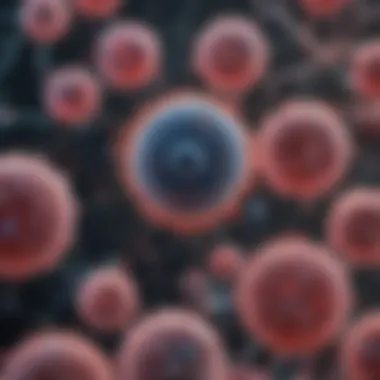
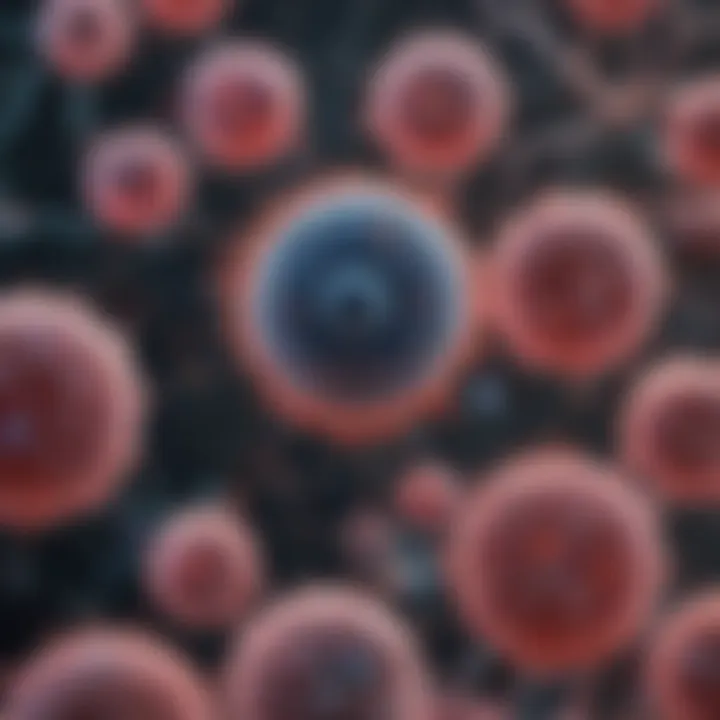
Intro
The convergence of stem cell research and tissue engineering represents a significant frontier in regenerative medicine. These two fields, while distinct, share a common goal: to repair or replace damaged tissues and improve the quality of life for patients with various medical conditions. Stem cells hold unique properties that allow them to differentiate into varied cell types, making them essential resources in tissue engineering endeavors.
In the evolving landscape of biotechnology, understanding how these fields interact is crucial. This article will examine the elemental principles that underscore both domains while providing insight into recent advancements and persistent challenges. Furthermore, ethical considerations that arise from such innovative research will be discussed, ensuring a well-rounded perspective of the topic.
Research Methodology
This exploration utilizes a comprehensive qualitative research design, drawing upon numerous scholarly articles, clinical trials, and case studies that address advancements in stem cell research and tissue engineering. Materials for this study include peer-reviewed journals, laboratory reports, and ethical guidelines that inform both fields.
Description of Research Design and Approach
The research approach is largely descriptive, aimed at synthesizing existing literature to present a cohesive view of how stem cells can enhance tissue engineering applications. Key areas of focus are:
- Stem cell types: Embryonic stem cells, adult stem cells, and induced pluripotent stem cells.
- Tissue engineering techniques: Scaffolding methods, bioprinting, and cell sourcing.
- Ethical considerations: Informed consent, potential for exploitation, and regulatory frameworks.
Materials and Methods Used in the Study
The study relies on secondary data, primarily historical and contemporary literature. Several methodologies are integrated to ensure thorough analysis:
- Literature reviews are conducted to contextualize findings within the existing body of knowledge.
- Comparative analyses are utilized to assess various tissue engineering techniques across different studies.
Discussion and Interpretation
Interpretation of Results in the Context of Existing Literature
The integration of stem cell research within tissue engineering practices is gaining momentum, as demonstrated by multiple studies evidencing successful tissue regeneration in preclinical models. Recent findings suggest significant improvements in outcomes when utilizing patient-derived stem cells, offering personalized treatment avenues. This advancement not only aids in enhanced therapeutic efficacy but also reduces the risks of immune rejection, a common concern in organ transplantation.
"The intersection of stem cell science and engineered tissues has opened new avenues for regenerative medicine, prompting both optimism and critical evaluation in ethical contexts."
Implications for Future Research or Practical Applications
The confluence of these fields anticipates several promising implications for future research:
- Enhanced therapeutic strategies for chronic diseases.
- Improved understanding of tissue development and function.
- Innovations in scaffold design that can mimic natural tissue characteristics more closely.
In terms of practical applications, the potential for individualized medicine becomes clearer. Personalized treatments tailored to an individual's cellular makeup could revolutionize how we approach medical conditions traditionally deemed resistant to standard therapies.
Preamble to Stem Cells and Tissue Engineering
The exploration of stem cells and tissue engineering is a vital subject in modern biomedical research. The synergy between these two fields has the potential to revolutionize medical treatments and greatly improve patient outcomes. Understanding stem cells provides insight into how tissues can be regenerated, repaired, or replaced. This perspective opens pathways to innovations in regenerative medicine.
Stem cells are unique due to their ability to differentiate into various cell types, making them invaluable for repairing damaged tissues. Their pluripotent nature allows researchers to explore numerous possibilities in treatment strategies. Conversely, tissue engineering focuses on the creation of biological substitutes that can restore, maintain, or improve tissue function. By merging these two areas, scientists can develop new therapies that harness the inherent capabilities of stem cells in constructing viable tissues.
- Advancements in understanding stem cell biology.
- Strategies for effectively integrating stem cells into engineered tissues.
- Ethical considerations related to stem cell sourcing and use.
- Challenges faced in real-world applications.**
By diving deeply into these elements, this article aims to provide a comprehensive guide that highlights current advancements, ongoing challenges, and future directions in this exciting field. A well-rounded understanding is essential for students, researchers, and professionals to navigate the complexities and potential of stem cell applications in tissue engineering.
Defining Stem Cells
Stem cells are unspecialized cells capable of self-renewal and differentiation into various specialized cell types. Their defining characteristics include:
- Self-renewal: The ability to undergo multiple divisions while maintaining their undifferentiated state.
- Differentiation potential: The capacity to transform into specialized cells (like muscle, nerve, or skin cells).
Stem cells can be categorized into different types, each with unique properties and sources. These include embryonic stem cells, which are derived from early-stage embryos, and adult stem cells, found in various tissues throughout the body.
Understanding Tissue Engineering
Tissue engineering is an interdisciplinary field that focuses on developing biological substitutes that restore, maintain, or improve tissue function. This discipline combines principles from biology, medicine, and engineering to create effective solutions for tissue repair. Key components of tissue engineering include:
- Cell sources: Choosing the right cells to use, which may include stem cells, to generate functional tissue.
- Scaffolds: Structures that provide support for growing new tissues. They help direct cell growth in a three-dimensional space.
- Bioreactors: Devices that simulate the biological environment to support cellular growth and tissue development.
Tissue engineering aims to create functional replacement tissues or organs to treat various diseases and injuries. Its relevance continues to grow with the increasing demand for organ transplants and the limitations of donor organs.
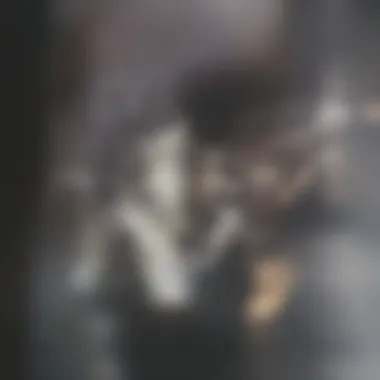
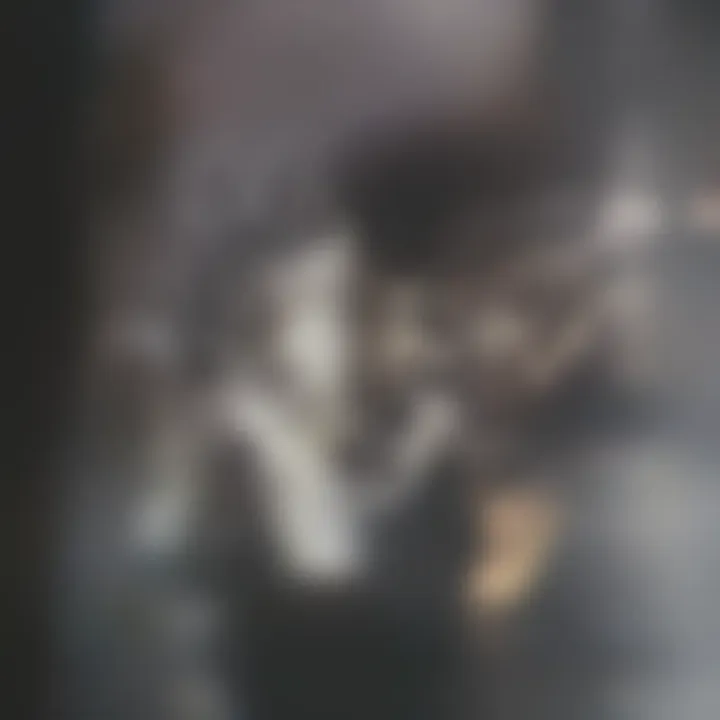
The Intersection of Stem Cells and Tissue Engineering
Combining stem cells with tissue engineering creates a powerful approach to regenerative medicine. This intersection provides multiple advantages, such as:
- Enhanced tissue regeneration: Stem cells can improve the quality and speed of tissue formation.
- Customization: Tissue engineered with stem cells can potentially be tailored to match a patient’s specific needs, thus fostering personalized medicine.
- Versatility: Stem cells can be manipulated in vitro (in a lab environment) to develop various tissue types for specific applications.
This integration allows for the development of advanced therapeutic strategies that aim to repair or regenerate damaged tissues functionalizating the body's natural healing processes.
Overall, the cooperation between stem cells and tissue engineering stands to significantly influence future medical practices. As research progresses, the understanding and application of these technologies will likely pave the way for groundbreaking treatments.
Types of Stem Cells
Understanding the different types of stem cells is fundamental for recognizing their various applications in tissue engineering and regenerative medicine. Each type of stem cell has distinct characteristics, abilities, and potential uses. This knowledge is crucial because it can guide researchers in choosing the proper stem cells for specific therapies. The advancements in these fields heavily rely on the unique properties of each stem cell type, allowing for tailored approaches that can target many medical challenges.
Embryonic Stem Cells
Embryonic stem cells (ESCs) are derived from early-stage embryos. These cells possess the remarkable ability to differentiate into any cell type in the body, making them pluripotent. This characteristic allows for extensive research in regenerative medicine since these cells can generate tissues and organs that are genetically compatible with patients. However, the use of embryonic stem cells raises ethical concerns, primarily about the source of the cells and the moral status of the embryos. The debate surrounding these issues has led to restrictions on ESC research in many regions, impacting how this valuable resource can be utilized in medicine. Despite the challenges, the potential of embryonic stem cells remains significant, especially in studies involving cellular development and disease modeling.
Adult Stem Cells
Adult stem cells, also known as somatic or tissue-specific stem cells, are found in various tissues in the body. Unlike embryonic stem cells, adult stem cells are typically multipotent, meaning they can develop into a limited range of cell types relevant to the tissue they reside in. For example, hematopoietic stem cells found in the bone marrow can transform into various blood cells but cannot become nerve or muscle cells. The relative ease of harvesting adult stem cells from patients greatly facilitates research and therapeutic applications. However, their limited differentiation potential poses challenges compared to embryonic stem cells. These limitations highlight the need for innovative methods to maximize the potential of adult stem cells in tissue engineering, such as through the development of specialized culture techniques or genetic modifications.
Induced Pluripotent Stem Cells
Induced pluripotent stem cells (iPSCs) are a breakthrough in stem cell research, created by reprogramming somatic cells to behave like embryonic stem cells. This process involves introducing specific genes that confer pluripotency to differentiated cells, allowing them to generate any cell type. The key advantage of iPSCs is their potential to produce patient-specific cells without the ethical dilemmas associated with embryonic stem cells. They offer a significant opportunity for personalized medicine, as researchers can derive these cells from a patient's tissues, creating a perfect genetic match for therapeutic applications. Studies continue to explore their possibilities in drug development, disease modeling, and even organ generation. Challenges remain in ensuring the safety and efficiency of using iPSCs in clinical settings, but their growing use is reshaping the landscape of regenerative medicine and tissue engineering.
Key Principles of Tissue Engineering
Tissue engineering bridges biology and engineering to develop functional substitutes for damaged tissues or organs. This field relies on understanding key principles, which include the selection of proper cell sources, scaffold design, and the use of bioreactor technologies. Each of these principles plays a vital role in ensuring the successful creation and functionality of engineered tissues.
Cell Sources for Tissue Construction
In tissue engineering, the origin of cells significantly impacts the end product. Different types of cells have varying capabilities to differentiate and proliferate.
- Autologous Cells: These are sourced from the same individual who will receive the treatment. They minimize the risk of immune rejection, but are limited by their availability and regenerative capacity.
- Allogenic Cells: Sourced from a donor of the same species, these cells can lead to complications if the immune system recognizes them as foreign.
- Xenogenic Cells: Derived from different species, these cells may offer broader options but come with significant ethical and biological challenges.
A careful choice among these cell sources is necessary, as it affects both the integration of engineered tissues and therapeutic outcomes.
Scaffold Design and Materials
A scaffold serves as a framework for cells to grow and organize. The design and materials used can influence cellular behavior profoundly.
Key considerations include:
- Biocompatibility: Materials must be compatible with biological tissues to avoid adverse reactions.
- Porosity: A highly porous scaffold allows for nutrient and waste exchange, essential for cell survival.
- Mechanical Properties: The scaffold should mimic the native tissue's mechanical environment to support cell adhesion and growth.
Common materials for scaffolds include natural polymers, synthetic polymers, and ceramics. Each material type presents distinct advantages and limitations, impacting the overall performance of engineered tissues.
Bioreactor Technologies
Bioreactors play a crucial role in maintaining the optimal environment for tissue development. They provide the necessary conditions for nutrient supply, waste removal, and mechanical stimuli, which can enhance cell behavior.
Several types of bioreactor systems are used:
- Static Bioreactors: Simple and cost-effective; these are suitable for small-scale tissue engineering but might not provide adequate oxygenation or nutrient distribution.
- Dynamic Bioreactors: Offer continuous flow of nutrients and oxygen, supporting larger tissue constructs and improving cellular function.
- Perfusion Bioreactors: Allow for enhanced nutrient transport, mimicking the physiological conditions of blood flow in vivo.
Choosing the right bioreactor system is essential for the success of tissue engineering projects.
Understanding these principles of tissue engineering not only advances science but also opens new avenues for treating diseases that currently have limited options.
Applications of Stem Cells in Tissue Engineering
The integration of stem cells in tissue engineering allows for significant advancements in various medical fields. The applications of stem cells in this domain have emerged as a pivotal area of research with real-world implications. By harnessing the properties of stem cells, researchers can seek innovative solutions to complex medical issues. This section elucidates the crucial aspects of these applications, detailing their benefits and critical considerations.
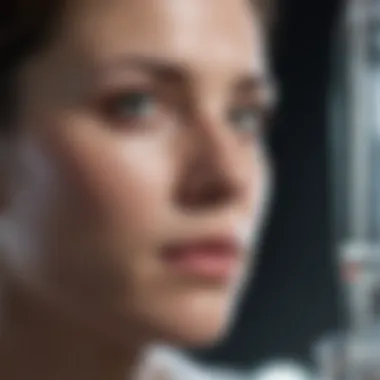
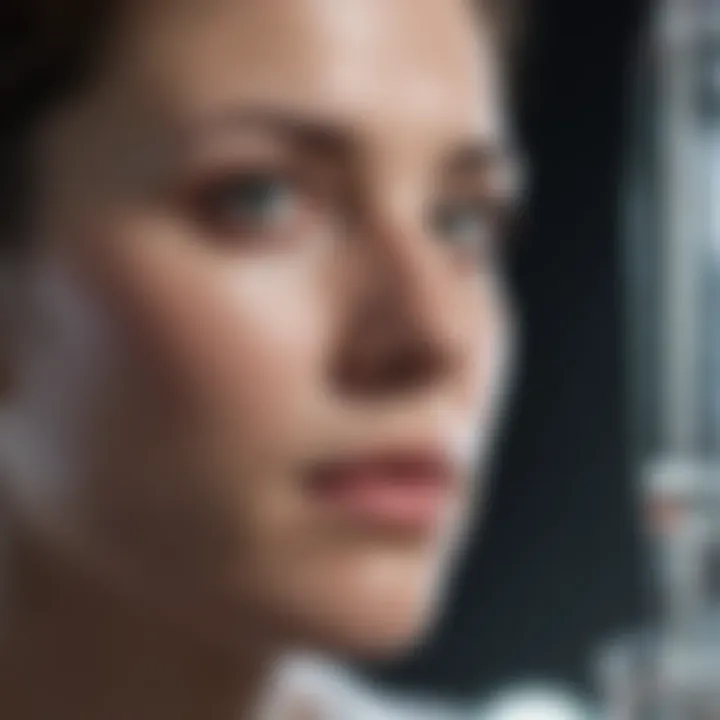
Regenerative Medicine
Regenerative medicine stands at the forefront of utilizing stem cells for tissue engineering. This approach focuses on repairing or regenerating damaged tissues and organs. One key benefit is the potential to restore functionality to compromised areas of the body without the need for invasive surgeries. For instance, the use of stem cells can lead to effective treatments for conditions such as heart disease and spinal cord injuries.
Research has shown that stem cells can differentiate into various cell types, thereby optimizing healing processes. The sustained availability of stem cells allows for ongoing replenishment of damaged tissues. However, complexities arise in ensuring the proper integration of these cells within existing tissue structures.
Organ Transplantation Alternatives
With the growing shortage of organ donors, stem cells offer hope as viable alternatives for organ transplantation. The ability to generate organs from stem cells can alleviate long waiting times for patients in need. Using patient-derived stem cells can minimize the risk of organ rejection because the cells are genetically identical to the patient.
The potential for designing functional organ replacements directly from stem cells requires robust biotechnological approaches. The field is advancing rapidly, achieving initial success in developing miniature organoids. These organoids mimic the functions of real organs, paving the way for future applications in personalized medicine. Careful consideration of ethical implications remains crucial as this technology evolves.
Chronic Disease Treatments
Chronic diseases often lead to progressive tissue damage, making treatment a persistent challenge. Stem cell applications in this realm show promise. For example, in diabetes care, researchers are looking at ways to regenerate pancreatic cells through stem cell therapy. This could replace the need for insulin injections for some patients.
In addition, spinal cord injuries, arthritis, and cardiovascular conditions present opportunities where stem cells can play a role in restoring function and quality of life. Adopting a multidisciplinary approach, incorporating both stem cells and advanced biomaterials, can enhance treatment efficacy.
In summary, the applications of stem cells in tissue engineering not only hold significant potential in advancing regenerative medicine, organ transplantation, and chronic disease treatment but also raise vital ethical and practical considerations. It is essential to balance the excitement of these advancements with responsible research practices to ensure the best outcomes for patients.
Challenges in Stem Cell and Tissue Engineering Research
The relationship between stem cell research and tissue engineering presents significant promise for the future of medicine. However, several challenges impede progress in integrating these fields effectively. Understanding these challenges is crucial for researchers, practitioners, and policymakers to navigate the complexities of advancing medical treatments.
Technical Limitations
One of the most pressing issues is the technical limitations in both stem cell research and tissue engineering. Researchers are still uncovering the most effective methods for expanding stem cell populations while maintaining their pluripotency and functional capabilities. Considerable variability exists in the growth conditions, which can lead to inconsistent results. Additionally, techniques for differentiating stem cells into specific cell types often yield mixed results. This variability may compromise the viability of engineered tissues and their integration into existing biological systems.
Furthermore, the complexity of human tissues and organs poses additional technical hurdles. Unlike simpler models, engineering complex structures that mimic multifaceted biological functions is considerably challenging. Current scaffolding materials and designs may not support the intricate cellular interactions necessary for functional tissues. Novel approaches such as 3D bioprinting and organ-on-a-chip technologies are being explored, yet practical applications still face multiple hurdles regarding scalability and functional efficacy.
Ethical Considerations
Ethical considerations play a central role in stem cell research and its application in tissue engineering. These concerns range from the sourcing of stem cells to the implications of genetic modifications. For instance, the use of embryonic stem cells raises ethical dilemmas related to the status of the embryo. Various countries have different regulations governing this area, complicating international research collaboration.
Moreover, when it comes to induced pluripotent stem cells, the potential for genomic instability remains a concern. Researchers must grapple with ethical implications regarding how these techniques are developed and applied, particularly when they involve modifying human genomes. An ongoing dialogue is vital to ensure that ethical standards advance alongside scientific advancements, fostering a responsible research environment.
Regulatory Hurdles
Regulatory hurdles are another substantial barrier to progress in this field. The integration of stem cells and tissue engineering into clinical practice requires navigating a complex landscape of health and safety regulations. Approval processes can be lengthy, often delaying the transition from laboratory research to practical application.
The lack of standardized protocols across jurisdictions further complicates the regulatory environment. This inconsistency can lead to discrepancies in safety evaluations and therapeutic efficacy across different regions. Therefore, harmonizing regulations and fostering collaboration among international regulatory bodies are essential steps to streamline the approval processes for emerging technologies in stem cell research and tissue engineering.
As the fields continue to evolve, addressing these challenges will be key to unlocking the full potential of stem cell applications in medicine.
Future Directions in Stem Cell and Tissue Engineering
The field of stem cell research and tissue engineering is rapidly evolving, holding immense potential for medical advancements. Understanding future directions allows stakeholders to anticipate innovations, refine methodologies, and address challenges effectively. This section outlines key elements shaping the future landscape and benefits of advancements within both fields.
Advancements in Genetic Engineering
Genetic engineering is set to play a pivotal role in the future of stem cell research and tissue engineering. Techniques such as CRISPR-Cas9 offer precision in genome editing, enabling researchers to correct genetic defects in stem cells. This advancement can lead to the production of cells tailored for specific patient needs, minimizing the risk of rejection during transplantation. Additionally, genetic modifications can enhance the capabilities of stem cells, increasing their regenerative properties.
- Benefits of Advancements:
- Targeted therapies for genetic disorders.
- Customization of stem cells for individualized treatment plans.
- Improved efficacy of tissue engineering applications.
The future lies in the meticulous control of genetic outcomes, ensuring that stem cells behave predictably within engineered tissues. Ethical considerations surrounding genetic manipulation must be continuously evaluated to uphold standards while pursuing innovation.
Integration of Nanotechnology
Nanotechnology represents another significant frontier in the intersection of stem cell research and tissue engineering. The ability to manipulate materials at the nanoscale can lead to the development of scaffolds that more closely mimic natural tissues, enhancing biocompatibility and functionality.
- Key Applications of Nanotechnology:
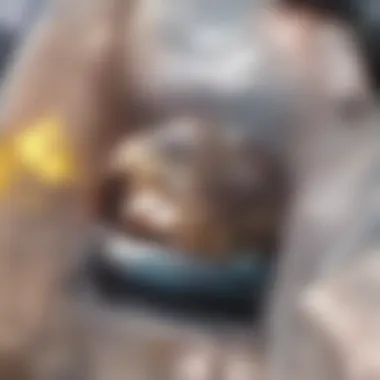
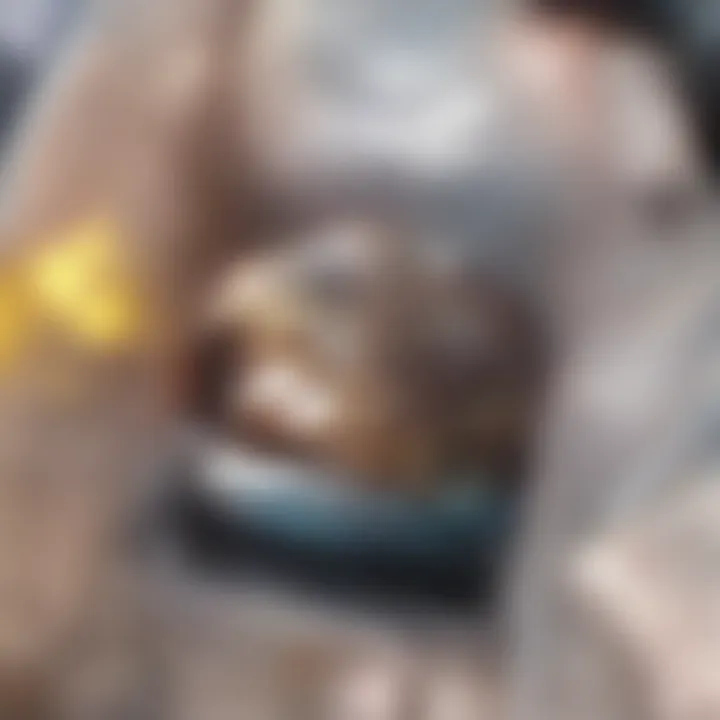
- Controlled drug delivery systems utilizing nanocarriers.
- Nanoscale scaffolds that promote stem cell attachment and differentiation.
- Sensors that monitor cellular responses in real time.
This integration paves the way for more effective regenerative strategies, with engineered tissues that are not only structurally sound but also responsive to physiological changes. Emphasizing safety and efficiency will be crucial as research progresses into real-world applications.
Potential for Personalized Medicine
Personalized medicine is a concept gaining traction, especially in the realm of stem cells and tissue engineering. By utilizing a patient’s own cells, researchers can tailor therapeutic approaches to individual needs, increasing treatment effectiveness while reducing complications.
- Advantages of Personalized Medicine Include:
- Reduced immune response and rejection rates when using autologous stem cells.
- Enhanced patient outcomes through customized treatments.
- Data-driven approaches that inform specific medical interventions based on genetic information.
With ongoing advancements in both stem cell technologies and bioinformatics, the potential for creating custom-engineered tissues that align perfectly with a patient's biology becomes more feasible. This trend indicates a shift towards holistic patient care, emphasizing the unique biological identity of individuals.
"The future of stem cell and tissue engineering will hinge on our ability to merge disciplines and create solutions that are not only innovative but also ethical and sustainable."
Case Studies in Stem Cell Applications
Case studies in stem cell applications are vital for understanding the practical impact of stem cell research on tissue engineering and regenerative medicine. They provide real-world examples that showcase the potential benefits, challenges, and complexities involved in translating scientific discoveries into clinical practice. Case studies serve several purposes: they offer insights into successful methodologies, help identify best practices, and inform future research directions. By closely examining these cases, researchers and practitioners can learn how to enhance patient outcomes and refine techniques for cell therapy and tissue regeneration.
Clinical Trials and Their Outcomes
Clinical trials are fundamental in assessing the efficacy and safety of stem cell therapies. Recent trials have explored various applications, such as treating spinal cord injuries, heart diseases, and neurodegenerative disorders. For example, studies involving mesenchymal stem cells have shown promising results in treating conditions like multiple sclerosis and arthritis.
Outcomes from these trials provide essential data:
- Efficacy in restoring lost functions
- Safety profiles, including possible side effects
- Long-term impacts on patients' quality of life
These outcomes help establish a foundation for wider adoption of stem cell-based therapies in clinical settings.
Successful Regeneration Cases
Numerous successful cases illustrate the transformative potential of stem cell applications. For instance, a notable case involved a patient with severe burns who underwent stem cell treatment leading to substantial skin regeneration. The use of autologous stem cells, derived from the patient’s fat tissue, prompted remarkable healing in damaged areas.
Other successful cases include patients who recovered mobility after receiving treatment for spinal cord injuries. For example, in some studies, patients shown improvements in motor function and sensation after stem cell implantation in the injured areas. These results highlight the capabilities of stem cells to regenerate tissues that were previously thought to be irreparably damaged.
Lessons Learned from Failures
While numerous successes exist, failures also provide crucial insights that drive advancements in stem cell research and tissue engineering. Failures may stem from various factors:
- Inadequate understanding of stem cell biology: Lessons from these setbacks often lead to more rigorous preclinical models that better mimic human conditions.
- Improper delivery methods: Some trials faced challenges with cell delivery systems that did not effectively guide cells to the target area.
- Unexpected immune responses: Certain cases revealed complications arising from the immune system’s reaction to stem cell therapies, prompting researchers to refine their approaches.
By analyzing these failures, the scientific community can develop more effective methods and strategies for future interventions. The overall goal remains clear: to enhance the safety and efficacy of stem cell applications for patient treatments.
Summary and Culminations
In this section, we reflect on the essential findings discussed throughout the article. The intersection of stem cell research and tissue engineering presents a promising frontier in medical science. Through a careful analysis of various principles and applications, it becomes evident that each contributes significantly to advancements in regenerative medicine.
The primary takeaway from this comprehensive exploration is the robust potential for stem cells to enhance tissue engineering practices. By understanding the unique properties of different stem cell types, such as embryonic, adult, and induced pluripotent cells, researchers can leverage their abilities to regenerate tissues effectively. Additionally, the integration of advanced scaffolding techniques and bioreactor technologies has paved the way for creating complex tissue structures that mimic natural organs.
Another crucial aspect highlighted in this article is the interplay between technical innovation and ethical considerations. As the field progresses, it is paramount to balance scientific advancement with respect for ethical standards. Ongoing dialogue among scientists, ethicists, and regulatory bodies is vital to navigate the complexities that arise in stem cell and tissue engineering research.
"The successful application of stem cells in tissue engineering will require continuous collaboration across diverse fields, ensuring both scientific and ethical integrity."
Moreover, the challenges outlined, including technical limitations and regulatory hurdles, serve to remind stakeholders of the work still needed in these domains. Identifying and addressing these obstacles will be key to the future success of integrating stem cells into tissue engineering.
In summary, understanding the synergy between stem cell research and tissue engineering is crucial for developing innovative treatments that can lead to enhanced patient outcomes. This intersection not only showcases the scientific potential but also sets a framework for future exploration in regenerative medicine.
Recapitulating Key Findings
In reviewing key findings, we reiterate the notable points:
- Stem Cells as Versatile Tools: Stem cells are pivotal in creating replacement tissues. Their capacity for self-renewal and differentiation is unmatched in medical applications.
- Tissue Engineering Techniques: The merging of stem cells with advanced scaffold designs results in functional tissue constructs. These methods enable better integration into host systems.
- Ethical Considerations Are Vital: Addressing ethical concerns helps build public trust and aids in regulatory compliance.
- Confronting Challenges: Technical and regulatory issues must be confronted head-on to realize the full potential of these technologies.
Implications for Future Research
Future research in stem cell and tissue engineering holds vast potential. Key implications for the direction of this field include:
- Innovative Engineering Approaches: New techniques in bioengineering can lead to the creation of more sophisticated tissue models. Such advancements can enhance the efficacy of regenerative therapies.
- Personalized Medicine: The potential for personalized approaches based on an individual’s stem cell characteristics can revolutionize treatments for various diseases and conditions.
- Collaboration Among Disciplines: Greater collaboration between geneticists, engineers, and medical professionals will likely foster innovative solutions and overcome current limitations.