Thermal Energy: Principles, Applications, and Future Prospects
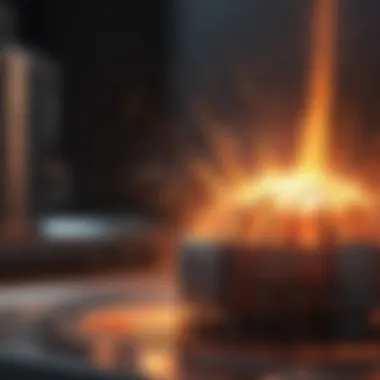
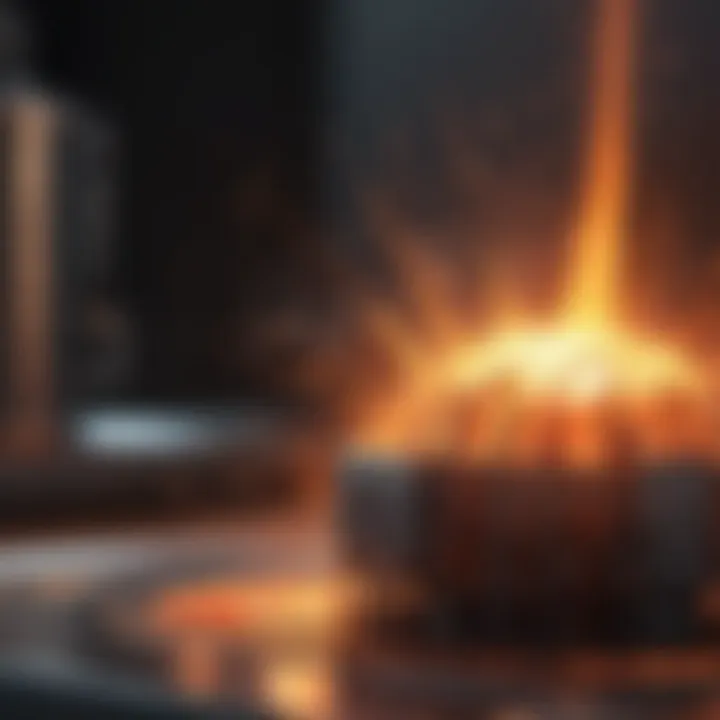
Intro
Thermal energy is a fundamental aspect of physics and engineering, representing heat energy that can be converted into other forms. Its principles govern processes from simple heating systems in homes to complex industrial operations. This article aims to analyze the significance of thermal energy in today's world. It will explore the basic principles, diverse applications across various sectors, and how emerging technologies are reshaping the future landscape of thermal energy.
Understanding thermal energy is crucial for students, researchers, educators, and professionals to grasp its impact on sustainability and the environment. This exploration will delve into key thermal energy concepts and their role in innovative solutions for energy challenges. Ultimately, the goal is to provide a comprehensive framework that illustrates thermal energy's importance in both societal and environmental contexts.
Research Methodology
To analyze the principles and applications of thermal energy, a structured approach was utilized. The research design involved qualitative and quantitative methods to gather relevant data. The data sources included academic literature, industry reports, and expert interviews.
Description of Research Design and Approach
The research began with a literature review, identifying crucial studies on thermal energy. Key areas included
the conversion of thermal energy, efficiency metrics, and advancements in thermal energy technologies. Interviews with industry professionals supplemented this literature. This dual approach enabled a robust understanding of current practices and potential future trends.
Materials and Methods Used in the Study
Data collection relied on primary and secondary resources. The following materials were integral:
- Academic journals and publications from trusted sources such as en.wikipedia.org and britannica.com
- Industry reports highlighting recent developments in thermal energy
- Expert interviews for practical insights into application and technology
This methodology ensured the information presented is comprehensive and relevant to the evolving thermal energy landscape.
Discussion and Interpretation
The findings indicate that thermal energy plays a vital role in numerous industries, including manufacturing, construction, and renewable energy. Its conversion into electricity and other useful forms has immense implications for energy sustainability.
Interpretation of Results in the Context of Existing Literature
Existing literature supports that advancements in thermal energy technologies are significant. For instance, innovations in geothermal energy and heat recovery systems have shown promising results. Studies highlight that effective thermal management can lead to efficiency enhancements in various operations.
Implications for Future Research or Practical Applications
Future research may focus on optimizing thermal energy conversion processes and sustainability practices. The integration of thermal energy systems with renewable sources like solar and wind is essential for addressing growing energy demands.
As thermal energy evolves, its importance in achieving sustainability goals cannot be overstated.
Continuous exploration in this field is necessary to adapt to changing energy needs and environmental challenges.
Prelims to Thermal Energy
Thermal energy plays a vital role in the realms of science and industry. Understanding this concept is essential for grasping how energy systems function and how they impact our daily lives. The significance of thermal energy cannot be overstated, as it is a cornerstone in numerous applications, from heating our homes to powering engines. Knowledge about thermal energy allows us to innovate and improve efficiency across several sectors.
Definition and Importance
Thermal energy refers to the internal energy present in a system due to the temperature of its molecules. It is critical as it facilitates heat transfer, enabling various natural phenomena and engineered systems to operate efficiently. One core principle is that thermal energy moves from hotter to cooler bodies, which underlies the operation of products like refrigerators, heaters, and numerous industrial processes.
The implications of understanding thermal energy extend beyond mere academic interest. It affects energy efficiency, cost savings, and environmental impact. Therefore, comprehending this concept deeply influences our ability to create sustainable solutions in energy production and consumption.
Historical Context
The study of thermal energy stretches back centuries. Early civilizations harnessed heat from fire, but it was not until the scientific revolution that systematic studies emerged. For instance, in the 17th century, scientists like Galileo and Bacon laid groundwork by exploring heat and its nature. In the 19th century, the establishment of thermodynamics marked a pivotal point, leading to significant advancements.
The development of technology capitalizing on thermal energy has shaped modern society. The invention of steam engines in the 18th century catalyzed the industrial revolution, demonstrating how thermal energy could be converted into mechanical energy. This understanding was gained through meticulous experimentation and refinement of existing theories. As we look at the historical evolution, it is clear that thermal energy has influenced economic and social structures throughout history.
As thermal energy technologies continue to evolve, their historical significance provides insights that inform current practices and future innovations.
The ongoing exploration and exploitation of thermal energy remain crucial as new challenges arise, notably in the context of climate change and sustainability. Policies and research endeavors are increasingly directed towards optimizing thermal energy in a way that meets contemporary demands, illustrating its enduring importance.
Fundamental Principles of Thermal Energy
Understanding the fundamental principles of thermal energy is crucial to grasp how this form of energy operates and influences various systems. At its core, thermal energy revolves around the movement of particles within matter, leading to temperature changes and heat transfer. This has significant implications across numerous sectors, from industrial processes to everyday applications in homes and vehicles.
Thermal Equilibrium
Thermal equilibrium is a state where two or more bodies at different temperatures come into contact and eventually reach a common temperature. This principle is vital for understanding energy flow and the efficiency of thermal systems. The ability to predict how systems behave at thermal equilibrium allows scientists and engineers to design better heat management solutions.
Essentially, when objects are in thermal contact, heat will flow from the hotter object to the cooler one until thermal balance is achieved. Establishing this state is foundational in applications like heating systems, where maintaining a steady temperature is necessary for optimal performance.
Heat Transfer Mechanisms
Heat transfer mechanisms play a central role in thermal energy management. They explain how heat moves from one location to another, influencing system design and efficiency. There are three primary methods of heat transfer: conduction, convection, and radiation.
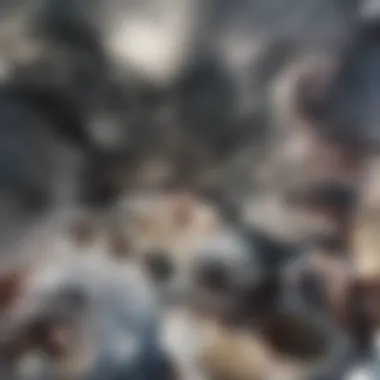
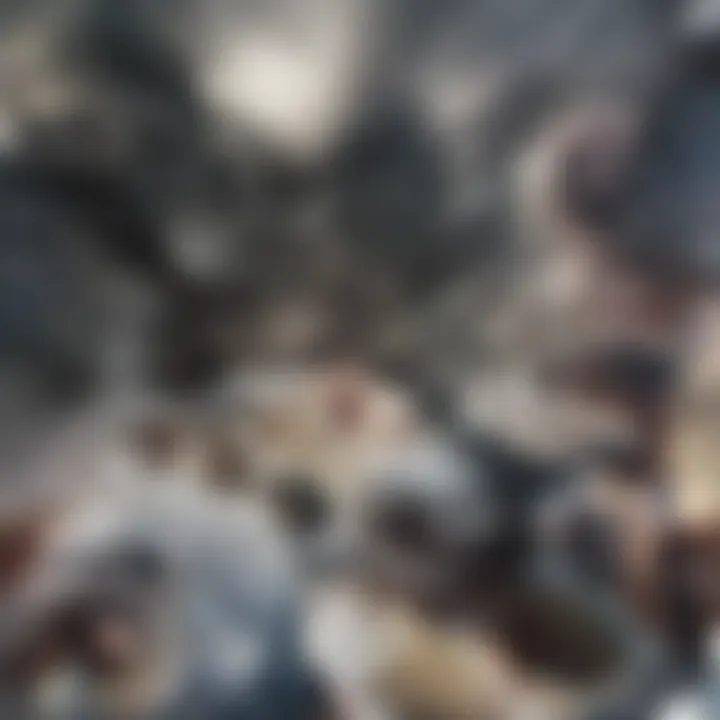
Conduction
Conduction involves the direct transfer of heat through a material, relying on particle interaction. The effectiveness of conduction depends on the material's properties, such as thermal conductivity. Metals like copper and aluminum are known for their high thermal conductivity, making them ideal for applications requiring efficient heat transfer, such as cooking utensils and heat exchangers.
A key characteristic of conduction is that it occurs primarily in solids. Once heat is applied to one end of a conductor, thermal energy moves to the cooler end without the physical movement of the material itself. This aspect makes conduction popular for applications in thermal insulation, where minimizing heat loss is essential.
Advantages of conduction include its efficiency in solid materials and its straightforward application in various technologies. However, its main disadvantage is limited heat transfer rate in insulators, which can pose challenges in certain situations.
Convection
Convection is the transfer of heat through a fluid (liquid or gas) due to the motion of the fluid itself. This can happen naturally or be forced by external means, such as fans or pumps. In a natural convection process, heated fluid rises while cooler fluid descends, creating a continuous flow that facilitates energy transfer.
A notable characteristic of convection is its ability to enhance heat transfer in systems such as heating and cooling reactors, where fluid motion improves efficiency. This mechanism is commonly used in home heating systems, where warm air rises and mixes with cooler air, achieving a uniform temperature distribution.
While convection significantly enhances heat transfer rates, the complexity of fluid dynamics can lead to challenges in control and maintenance. Additionally, the efficiency of convection is influenced by fluid properties and flow patterns, making optimization essential.
Radiation
Radiation is the transfer of energy in the form of electromagnetic waves. Unlike conduction and convection, radiation does not require a medium; it can occur in a vacuum. This characteristic makes radiation interesting in the context of technologies such as solar panels, which convert sunlight directly into usable energy.
The key feature of radiation is its dependence on temperature. Objects at higher temperatures emit more radiant energy than cooler ones. Thus, managing thermal radiation is crucial in applications like thermal insulation and passive solar building designs, where minimizing energy loss is critical.
Despite its benefits, such as remote energy transfer and the ability to work in a vacuum, radiation can lead to inefficiencies in certain engineering contexts, requiring careful consideration in system design.
"Understanding these heat transfer mechanisms is pivotal for developing efficient thermal energy systems and technologies."
In summary, the fundamental principles of thermal energy, including thermal equilibrium and heat transfer mechanisms, are essential for effectively utilizing this energy form. They provide the framework for engineers and scientists to innovate in thermal management solutions, impacting various industries.
Sources of Thermal Energy
Understanding the sources of thermal energy is crucial for grasping the overall landscape of thermal energy applications and technologies. These sources can be broadly categorized into natural and artificial origin. Each type presents unique benefits and challenges that shape its use in various sectors. The shift towards sustainable practices highlights the importance of utilizing diverse energy sources, ensuring we can meet current and future energy needs effectively.
Natural Sources
Geothermal Energy
Geothermal energy derives from the heat stored beneath the Earth's surface. This source is significant due to its reliability and consistency. Unlike solar or wind energy, geothermal can produce a continuous energy supply regardless of weather conditions. A key characteristic of geothermal energy is its low emissions profile; it releases far fewer greenhouse gases than fossil fuels.
One unique feature is the ability to harness geothermal energy for both electricity generation and direct heating applications. Thermal energy from geothermal sources can be used for district heating systems, enhancing energy efficiency in urban areas. However, the initial investment and specific location requirements can pose challenges. Regions with geological activity are ideal, but these are not universally accessible.
Solar Thermal Energy
Solar thermal energy involves capturing sunlight to produce heat, often used in residential and industrial applications. Its importance lies in its renewable nature, which allows it to contribute significantly to reducing carbon emissions. A fundamental characteristic of solar thermal energy is its ability to be stored, making it versatile for various heating needs.
This source offers unique advantages such as lower operational costs and scalability. Solar thermal systems can be tailored to fit small homes or large industrial units. However, factors such as geographical location and daylight hours influence its efficiency. Therefore, while it presents a powerful solution for sustainable energy, careful site evaluation is necessary for effective implementation.
Artificial Sources
Fossil Fuels
Fossil fuels remain among the most prevalent sources of thermal energy globally. They encompass coal, oil, and natural gas, which are integral to meeting energy demands in many industries. A primary characteristic of fossil fuels is their energy density. They provide high amounts of energy per unit, making them an attractive option for electricity generation and heating.
Despite this efficiency, several disadvantages accompany their use. The combustion of fossil fuels contributes significantly to air pollution and greenhouse gas emissions. These environmental impacts are a growing concern, driving the push towards cleaner energy alternatives. Moreover, the finite nature of fossil fuel reserves raises questions about long-term sustainability.
Nuclear Power
Nuclear power serves as a potent source of thermal energy, characterized by its ability to generate large amounts of energy from a small amount of fuel. By utilizing controlled nuclear reactions, this method stands out for its efficiency and low greenhouse gas emissions during operation. Nuclear power can provide base-load energy, meaning it supplies a continuous and stable energy output.
The unique feature of nuclear energy lies in its high energy yield and the ability to reduce reliance on fossil fuels. However, challenges exist, such as the management of nuclear waste and concerns about nuclear accidents. Public perception around nuclear safety is an ongoing issue, influencing its adoption in new energy policies. This balance of benefits and risks contributes to the ongoing debate regarding its role in sustainable energy strategies.
"The transition to cleaner and more sustainable energy forms starts with understanding our current sources and their impacts."
In summary, both natural and artificial sources of thermal energy provide essential contributions to the global energy mix. Their evaluation leads to a better understanding of how we can optimize their benefits while addressing their challenges. By advancing these technologies and selecting appropriate sources, we can enhance thermal energy applications across different sectors.
Thermal Energy Conversion Technologies
Thermal energy conversion technologies play a vital role in harnessing and utilizing heat energy from various sources. This section explores the technologies leveraged to convert thermal energy into usable forms, which are essential in both industrial and residential settings. Understanding these technologies helps us appreciate their potential in energy management and sustainability.
Heat Engines
Heat engines are a crucial component in the conversion of thermal energy. They take heat energy and transform it into mechanical work. The efficiency and type of the heat engine determine the overall energy conversion process. Different types of heat engines have unique operational characteristics that suit various applications.
Types of Heat Engines
There are several types of heat engines, mainly classified as internal combustion engines and external combustion engines. Internal combustion engines operate by burning fuel within the engine itself, leading to a rapid buildup of pressure that drives pistons. This type is commonly used in vehicles. On the other hand, external combustion engines, like steam engines, use heat from an external source to produce steam that drives a piston or turbine.
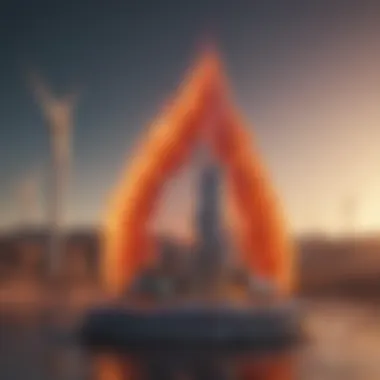
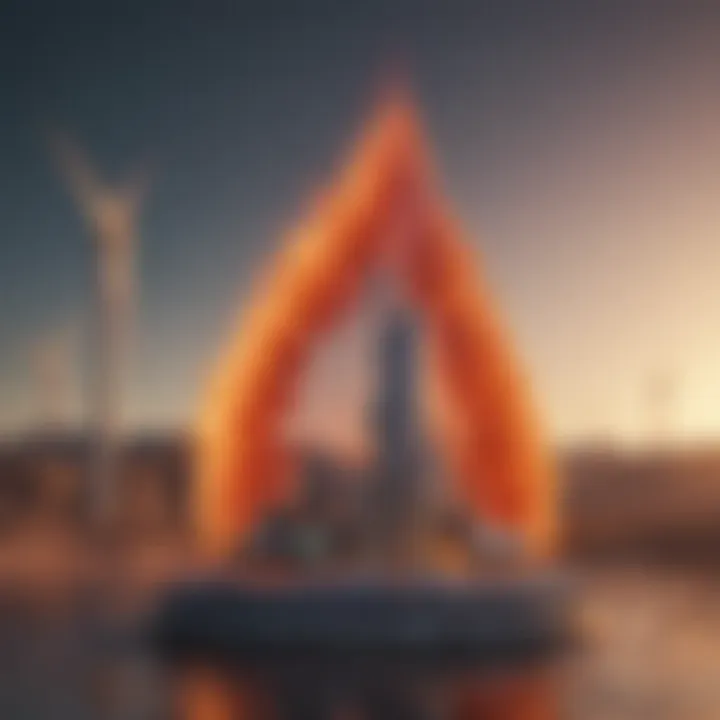
Key characteristics of heat engines include their efficiency and the types of fuel they can use. Internal combustion engines are popular due to their compact size and ease of use. However, they do have environmental drawbacks, such as emissions. External combustion engines can burn a variety of fuels and are generally more environmentally friendly, but they are often larger and less efficient compared to internal combustion counterparts.
Efficiency Considerations
Efficiency is a significant factor in the design and operation of heat engines. The efficiency of a heat engine is defined as the ratio of useful work output to the heat energy input. High efficiency is desirable as it reduces fuel consumption and minimizes environmental impact. Certain designs, like the Rankine cycle for steam engines, can enhance efficiency.
A common characteristic of efficiency considerations in heat engines is that they vary significantly with temperature differences. The greater the temperature difference between the heat source and the sink, the higher the potential efficiency. Despite these advantages, achieving high efficiency while complying with environmental regulations remains a challenge.
Heat Pumps
Heat pumps are another essential technology for thermal energy conversion, as they can transfer heat from one place to another, effectively moving thermal energy instead of converting it entirely into mechanical work. This technology is increasingly relevant in both cooling and heating applications.
Working Principle
The working principle of heat pumps is based on the refrigeration cycle. They utilize a refrigerant that absorbs heat from the environment and transfers it indoors. This process can be reversed for cooling purposes. One unique feature of heat pumps is the ability to provide heating and cooling from the same unit, allowing for flexibility in thermal management.
Heat pumps are energy-efficient since they move heat rather than generating it. However, their efficiency is highly influenced by the source temperature, particularly in colder climates. This can lead to decreased performance in extremely low temperatures.
Applications
The applications of heat pumps are diverse. They are widely used for residential heating and cooling systems, domestic hot water production, and even in industrial processes for specific heating needs. This versatility is a key characteristic that makes heat pumps an appealing choice.
However, the installation of heat pumps may require upfront investment, which can be a limitation for some users. Additionally, their optimal performance may vary based on the local climate conditions, which needs careful consideration in regions with extreme temperatures.
"Thermal energy conversion technologies are critical in promoting energy efficiency and sustainability. Their proper implementation can contribute significantly to reducing our carbon footprint."
In summary, both heat engines and heat pumps represent crucial technologies in the realm of thermal energy conversion. They not only enable the effective utilization of thermal energy, but also facilitate the transition toward more sustainable energy practices.
Applications of Thermal Energy
The applications of thermal energy are critical in many aspects of our daily lives and various industries. This section explores the significant roles played by thermal energy in industrial, residential, and transportation sectors. This exploration highlights how thermal energy contributes not only to efficiency and cost-effectiveness but also to sustainability efforts in addressing environmental concerns. Understanding these applications aids in appreciating the vast potential of thermal energy in modern society.
Industrial Applications
Manufacturing Processes
Manufacturing processes heavily depend on thermal energy for production. The conversion of raw materials into finished products often involves heat to facilitate chemical reactions and physical changes. A key characteristic of such processes is the controlled application of heat, which allows for precise manipulation of material properties. This makes it a beneficial choice for industries such as metallurgy, food processing, and plastics.
One unique feature of manufacturing processes is that they can significantly affect the quality of end products. For instance, inadequate heat treatment in metalworking can lead to weakened materials. However, the main disadvantage is the high energy consumption associated with these processes, which can sometimes lead to increased operational costs.
Process Heating
Process heating is a fundamental aspect of numerous industries, providing the necessary thermal energy for various applications. This includes dairy pasteurization, glass melting, and many other sectors. A primary characteristic of process heating is its ability to deliver heat directly where needed, enhancing efficiency and productivity. This technique is particularly beneficial for operations that require consistent and precise temperature control.
The unique feature of process heating systems is their versatility. They can use electric, gas, or other forms of thermal energy. However, the main drawback is the dependency on the energy source, which can be a limitation in places where certain fuels are not available or are costly, impacting overall sustainability.
Residential Applications
Heating Systems
Heating systems in residential settings are essential for comfort during colder months. This sector primarily utilizes thermal energy generated through various means, including fossil fuels and solar power. A key characteristic that makes heating systems popular is their effectiveness in maintaining a stable and comfortable indoor climate.
These systems can be centralized or decentralized, which allows flexibility in design and installation. However, a disadvantage is the potential for high energy costs, especially if the system relies on less efficient energy sources.
Hot Water Production
Hot water production is another critical application of thermal energy in residential homes. Households require hot water for bathing, cleaning, and cooking. The primary characteristic of these systems is the ability to heat water quickly and efficiently, often through systems like boilers or solar hot water collectors.
The unique feature of hot water systems is their integration with both heating and plumbing systems, ensuring hot water is available as needed. However, the disadvantages can include high maintenance costs and dependence on the energy source used for heating.
Transportation
Thermal Management in Vehicles
In modern vehicles, thermal management refers to the systems that regulate temperature for optimal performance. Engine cooling systems, climate control, and battery temperature management all fall under this category. A major characteristic of thermal management is its critical role in maintaining vehicle efficiency and safety.
One unique feature is the use of advanced materials and technologies to enhance thermal management efficiency. However, the disadvantages can include increased complexity, which may raise repair costs and affect the longevity of vehicle components.
Rail and Marine Applications
Rail and marine applications also utilize thermal energy effectively. In rail, electric locomotives rely on thermal energy for powering systems. In marine applications, ships often employ thermal energy for propulsion and onboard operations. A key characteristic is the reliance on diesel or biofuels to generate the required thermal energy.
The unique feature of these applications is the scale at which thermal energy can operate, often enabling large payload capacities and long-distance travel. However, the environmental impact of emissions from traditional fuels remains a significant disadvantage, pushing the industry toward exploring greener alternatives.
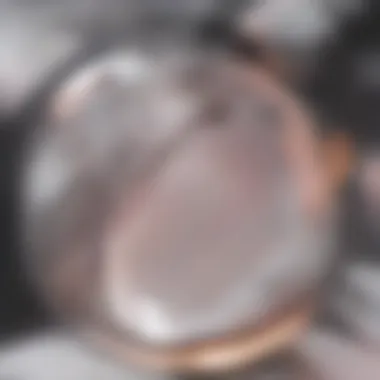
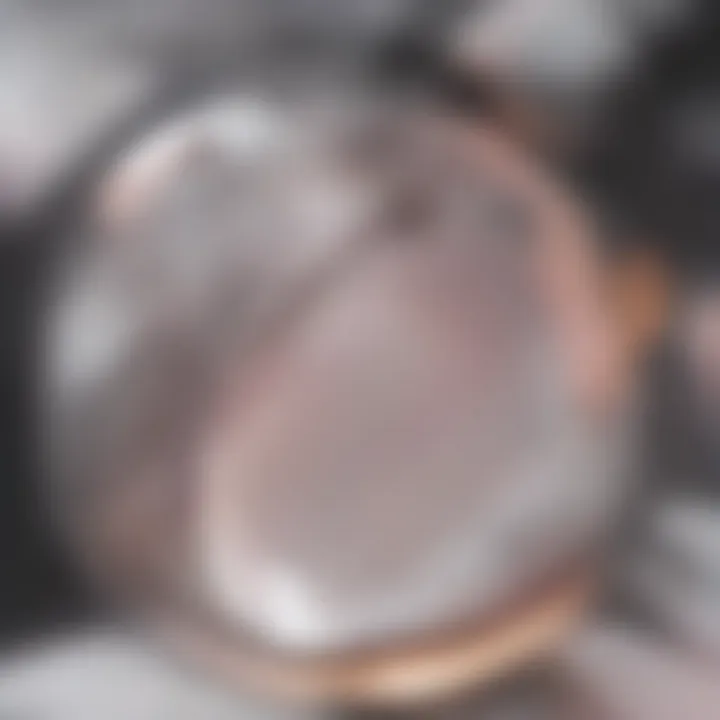
Understanding the diverse applications of thermal energy paves the way for innovations that can enhance efficiency and reduce environmental impact.
Challenges and Limitations of Thermal Energy Use
Understanding the challenges and limitations of thermal energy is crucial in the broader context of energy discourse. While thermal energy plays a pivotal role in various industrial and residential applications, several barriers limit its effective utilization. These challenges are not only of theoretical interest but have practical implications on the way thermal energy is generated, converted, and employed in modern society. Addressing these issues is vital for enhancing energy sustainability and optimizing thermal energy technologies.
Environmental Impacts
The environmental impact of thermal energy generation is significant. This aspect enhances the urgency to assess how thermal energy contributes to ecological challenges. While certain forms of thermal energy, like geothermal energy, are much cleaner, traditional methods like burning fossil fuels release considerable greenhouse gases and pollutants.
For instance, the combustion of coal and natural gas emits carbon dioxide, a major contributor to climate change. Furthermore, thermal power plants generate waste heat, which can harm aquatic ecosystems when discharged into rivers and lakes. The resultant thermal pollution leads to a decrease in water quality, affecting aquatic life.
Another environmental concern arises from land use changes associated with thermal energy production. Oil drilling, coal mining, and extensive geothermal installations may lead to habitat destruction or alteration. Such ecological displacements can trigger a chain reaction, ultimately threatening biodiversity.
In summary, while thermal energy is fundamental to modern energy systems, its environmental footprint cannot be overlooked.
Efficiency Gaps
Another critical limitation is the efficiency gaps in thermal energy systems. Despite advancements in technology, thermal conversion processes often suffer from inefficiencies. For example, traditional steam turbines used in thermal power plants typically exhibit efficiencies around 30%-40%. This means a significant portion of the energy generated is wasted as heat and not converted into useful work.
An interesting aspect of these inefficiencies is their historical context. The laws of thermodynamics dictate that no energy conversion can be 100% efficient. However, modern innovations strive to close these efficiency gaps through various methods. Enhanced heat exchangers and advanced materials have shown promise in improving heat transfer and minimizing losses.
Moreover, in processes such as heating systems in homes, there are varying degrees of efficiency. Many older heating systems, such as furnaces or boilers, work at lower efficiency rates compared to newer technologies like condensing boilers or heat pumps. The transition to these more efficient systems requires investment, but the long-term savings and environmental benefits can be significant.
Finale
The challenges and limitations of thermal energy use highlight the need for continued research and development. By addressing environmental issues and closing efficiency gaps, society can move towards a more sustainable energy future. Innovative solutions are necessary to tackle these important concerns while maximizing the potential of thermal energy in an ever-evolving technological landscape.
Future Trends in Thermal Energy Research
The field of thermal energy is evolving rapidly. Advances in technology and research are pushing the boundaries of what is possible. The importance of understanding future trends in this area cannot be overstated. As societies shift towards sustainable energy solutions, thermal energy plays a vital role. It is crucial to explore advancements that can lead to more efficient energy use and conversion.
Advancements in Material Science
Material science is at the forefront of thermal energy research. Innovative materials are being developed to enhance energy efficiency. For instance, improved insulation materials significantly reduce heat loss in buildings, which helps in energy conservation. Another development includes phase change materials (PCMs) that store and release thermal energy as they change states. These materials can maintain temperature stability, especially in residential and industrial settings.
Additionally, nanomaterials are being studied for their heat transfer properties. Their unique structures allow for increased thermal conductivity while being lightweight. This can lead to more efficient thermal energy systems. Researchers are also focusing on developing smart materials. These materials can respond dynamically to temperature changes, optimizing energy performance.
"Advancements in materials directly contribute to the efficiency and sustainability of thermal energy systems, influencing diverse applications from industrial processes to energy management in buildings."
Integration with Renewable Energy Sources
Integrating thermal energy with renewable sources is gaining momentum. Solar thermal energy systems, for example, capture sunlight to generate heat. This heat can be used for various applications, including residential heating or industrial processes. By combining solar thermal systems with conventional boilers, energy efficiency can be improved.
Wind and hydropower also present opportunities for thermal energy integration. Waste heat from these power plants can be harnessed for district heating systems, which distribute thermal energy to multiple buildings. This approach maximizes resource use and minimizes waste.
Moreover, research is focusing on hybrid systems that combine thermal energy technologies with storage solutions, such as molten salt storage. This ensures a consistent energy supply even when the sun isn't shining or the wind isn't blowing. As a result, such integration fosters energy reliability and sustainability.
Closure
In this article, the exploration of thermal energy reveals its significance across various sectors. The conclusion underscores the accumulated insights into fundamental principles, diverse applications, and emerging technologies related to thermal energy. By clarifying how thermal energy serves as a critical component in both industrial and residential contexts, the article highlights its essential role in modern energy systems.
Summary of Key Points
- Definition and Principles: Thermal energy operates based on principles of heat transfer, which include conduction, convection, and radiation. Each mechanism facilitates energy exchange in distinct ways, impacting efficiency and functionality.
- Sources and Technologies: Various natural and artificial sources, such as geothermal energy and fossil fuels, contribute to thermal energy generation. Advanced technologies, including heat engines and heat pumps, showcase conversion techniques vital for broader energy applications.
- Applications: The utilisation of thermal energy spans multiple sectors, from manufacturing processes to residential heating systems, demonstrating its versatility.
- Challenges: Environmental impacts and efficiency gaps present ongoing concerns, necessitating careful consideration in the development and implementation of thermal energy solutions.
- Future Trends: Ongoing research in material science and the integration of renewable energy are trends shaping the future landscape of thermal energy.
Call for Continued Research
The field of thermal energy warrants ongoing investigation. Future research should focus on:
- Innovative Technologies: Developing more efficient thermal energy conversion technologies could enhance sustainability.
- Material Innovations: Research into new materials that improve heat transfer effectiveness can lead to significant advancements in efficiency.
- Environmental Assessment: Understanding the ecological impacts of thermal energy use remains crucial. Evaluating these impacts can inform better energy policy and practice.
- Interdisciplinary Approaches: Collaborating across disciplines, such as engineering and environmental science, can provide holistic solutions to thermal energy challenges.
"Continued research is vital to harnessing thermal energy's potential responsibly and sustainably."
Through collective efforts in research and development, the growing role of thermal energy can address energy demands while mitigating environmental impacts.
Specific Elements
The references included in this article cover a wide range of topics related to thermal energy:
- Historical developments in thermal energy technologies.
- Current trends and innovations in thermal energy applications.
- Academic studies that delve into environmental impacts and efficiency considerations.
- Collaboration reports between researchers and industrial stakeholders highlighting best practices.
Benefits of Proper Referencing
- Enhanced Credibility: Academic rigor increases trust in the material presented.
- Facilitated further reading: Readers can pursue original research for more detailed understanding.
- Encouragement of scholarly dialogue: Proper citations promote discussions within the academic community.
Considerations About References
When compiling references, it is crucial to consider:
- The reliability of the sources.
- The relevance of the information to the current research landscape.
- Balancing between classical texts and contemporary studies to provide a well-rounded view.