Fluorescence Microscopy: Applications Across Disciplines
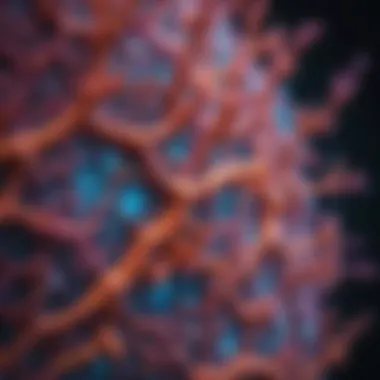
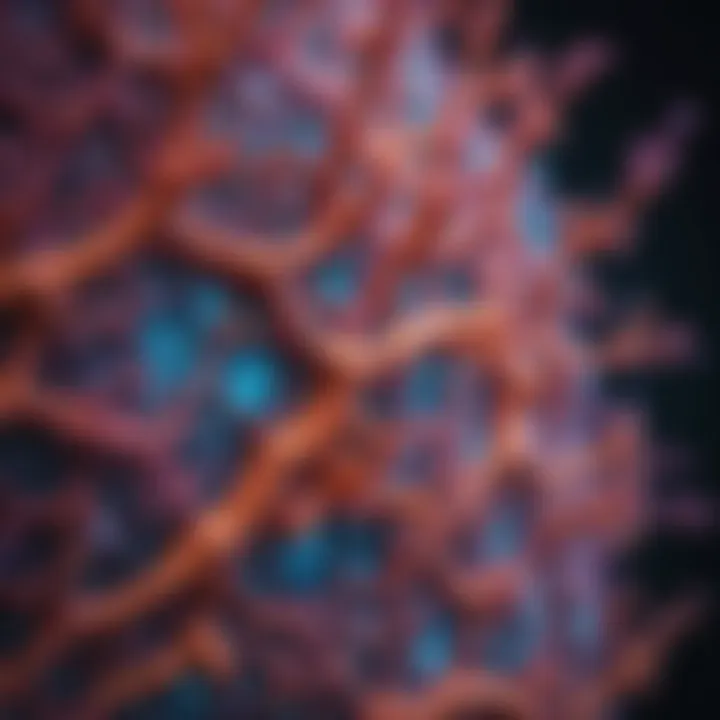
Intro
Fluorescence microscopy has sculpted a new frontier in scientific inquiry, allowing researchers to peer into intricate cellular landscapes and unravel the tapestry of life at the molecular level. Its applications stretch far beyond the walls of biology labs; this technique has become indispensable in fields ranging from materials science to environmental research. By harnessing fluorescence, scientists can illuminate specific components within cells, observe dynamic processes in real-time, and investigate the subtle interactions that characterize various materials and biological entities.
This exploration offers not just a glimpse into the operational mechanisms of fluorescence microscopy, but also showcases its versatility across disciplines. Understanding the practical applications of this powerful imaging technique is vital for students, researchers, educators, and professionals who seek to leverage its capabilities in their respective fields.
Research Methodology
Description of Research Design and Approach
A robust research design is critical when leveraging fluorescence microscopy. This methodology typically begins with the selection of fluorescent probes, which are specific to the target molecules or structures of interest. The process starts with defining a clear hypothesis or objective. For instance, if researchers aim to study the migration of certain proteins during cellular processes, appropriate fluorescent tags must be chosen to effectively visualize these proteins.
The design often entails both in vitro and in vivo studies, depending on the research focus. In vitro studies may involve cultured cells, where fluorescent markers illuminate cellular compartments. In contrast, in vivo examinations provide insights into physiological processes in whole organisms, delivering a more holistic view.
Materials and Methods Used in the Study
In the execution of fluorescence microscopy research, various materials and methods come into play:
- Fluorescent Dyes and Probes: These are crucial in selecting appropriate markers for labeling specific structures, such as nucleus or mitochondria. Common examples include DAPI for nuclei and GFP (Green Fluorescent Protein) for a variety of cellular components.
- Microscopy Techniques: Different microscopy setups, including confocal and two-photon microscopy, allow for enhanced imaging depth and resolution. Each method has its own strengths, tailored to particular research objectives.
- Data Acquisition Software: This software plays a vital role in capturing images and analyzing data, allowing researchers to draw meaningful conclusions from their observations.
In terms of practical implementation, researchers may utilize sample preparation techniques that involve fixing, permeabilizing, and staining cells. An example would be the preparation of bioengineered tissues where the structural integrity and functionality of cells are paramount during observation.
Discussion and Interpretation
Interpretation of Results in the Context of Existing Literature
The findings derived from fluorescence microscopy are often juxtaposed against existing literature to provide context. For example, when investigating the localization of a protein suspected to be involved in cellular signaling, it is crucial to compare observations with established studies that outline protein behavior and interactions. This comparative analysis not only validates results but can also highlight novel insights about cellular functions that were previously overlooked.
Implications for Future Research or Practical Applications
The implications of research findings are wide-ranging. From advancing medical diagnostics to informing environmental policy through the study of pollutant interactions, fluorescence microscopy has applications that can change the course of various scientific domains. Future research can explore:
- Therapeutic Targets: Understanding how certain molecules behave in disease environments can reveal new avenues for drug development.
- Material Characteristics: In materials science, examining the fluorescence properties of new compounds can enhance material development.
In summary, the transformative impact of fluorescence microscopy cannot be understated. By illuminating the microscopic world, it not only enhances our understanding of complex phenomena but also paves the way for innovative applications across diverse scientific fields.
Prologue to Fluorescence Microscopy
Fluorescence microscopy stands at the forefront of modern scientific inquiry, playing a pivotal role in various fields such as biology, chemistry, and environmental science. Its significance lies not just in its ability to visualize structures at the cellular or molecular level, but also in the insight it provides into dynamic processes occurring in living organisms and complex materials. By using fluorescent labeling techniques, researchers can tag specific molecules or structures, enabling them to track interactions and behaviors that would be impossible to see otherwise.
The allure of fluorescence microscopy is its versatility. It is a powerful tool employed across diverse scientific disciplines, making it beneficial for academic researchers and industrial applications alike. Understanding its core principles and historical development is essential to appreciate how it has transformed research methodologies.
Fluorescence microscopy's ability to provide real-time, high-resolution images has revolutionized the analysis of biological and chemical processes, offering a glimpse into a world that previously remained unseen.
Historical Development
The journey of fluorescence microscopy is a fascinating one that began in the 19th century. Initial explorations into fluorescence came from the basic discovery of fluorescent materials. One notable moment was the identification of fluorescein in 1871 by Adolf von Baeyer, which opened doors to understanding fluorescence as a concept. However, it wasn't until the early 20th century when fluorescence microscopy as we know it started to take shape.
By the 1930s, researchers like C.W. Garder and Hugh M. T. D. K. Hill introduced fluorescence filters and improved optics, paving the way for enhanced imaging capabilities. The 1960s brought significant advancements with the introduction of laser technology, which refined excitation illumination—a game-changer in obtaining sharper images. The development of various fluorescent dyes further fueled its evolution, making it an indispensable asset in biological research.
Basic Principles of Fluorescence
To grasp how fluorescence microscopy works, one must understand the fundamental principles behind fluorescence itself. In essence, fluorescence occurs when a substance absorbs light at one wavelength and subsequently re-emits it at a longer wavelength. This happens because the absorbed energy excites electrons, which then return to their original state, releasing energy in the form of light during the process.
- Excitation: The process begins when a fluorophore, a molecule that can emit light, absorbs photons from a light source, typically a mercury vapor lamp or a laser.
- Emission: After absorption, the fluorophore enters an excited state and eventually returns to a ground state, emitting light in the form of fluorescence. This emitted light can be captured for imaging.
- Detection: Specialized detectors convert the emitted light into a signal that forms the image displayed on a monitor.
Fluorescence microscopy harnesses these principles, allowing scientists to study biological specimens with incredible specificity and contrast, thus forming the backbone of many research endeavors.
Fundamentals of Imaging Techniques
The role of fundamentals in imaging techniques cannot be overstated. These foundational elements lay the groundwork for the myriad applications of fluorescence microscopy in various domains. Grasping the fundamentals enables scientists and researchers to tweak parameters for optimal imaging, thus enhancing resolution and contrast. In fluorescence microscopy, knowing the specific imaging techniques and their suitable applications can be crucial for obtaining accurate and meaningful data.
Types of Fluorescence Microscopy
Conventional Fluorescence Microscopy
Conventional fluorescence microscopy is like the bread and butter of this field. It’s often the first stop for researchers wanting to visualize samples at the microscopic level. This technique primarily uses widefield illumination, making it relatively straightforward and cost-effective. One of the key characteristics is its ability to capture a wider field of view at once, which is a fantastic boon when you want to survey larger areas. However, this approach sometimes falls short in terms of resolution compared to more advanced methods.
The unique feature of conventional fluorescence microscopy is its accessibility. Any lab with a basic fluorescence setup can start using it right away. However, it’s not without its downsides; the image quality can suffer from out-of-focus light, leading to less sharp images.
Confocal Laser Scanning Microscopy
When precision is the name of the game, confocal laser scanning microscopy (CLSM) steps into the spotlight. This technique employs point illumination and a pinhole to improve resolution and focus on specific planes within a sample. It's particularly popular in cellular studies, as it allows for 3D reconstruction of images with immense clarity. The scoring point? It minimizes the background noise, making the vital details pop out.
CLSM’s unique feature lies in its ability to image thicker samples or layers of cells, imperative for understanding biostructures. However, this robustness comes with a cost: it is more expensive and can have limits in imaging speed.
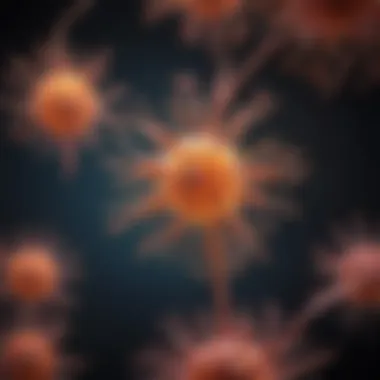
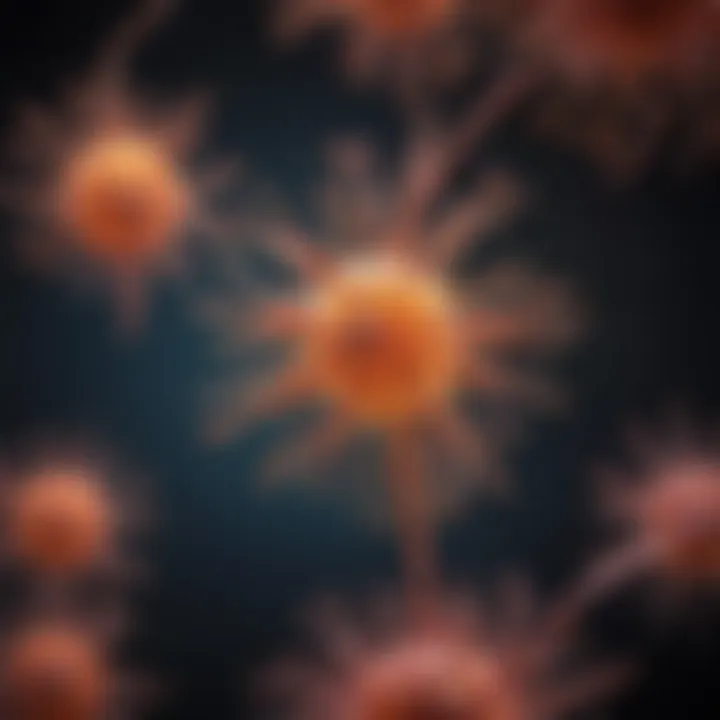
Super-Resolution Microscopy
You might think that there’s a hard ceiling on how small things can be resolved. But super-resolution microscopy challenges that notion. Techniques like STED or PALM push the limits beyond the diffraction limit, providing unprecedented details of cellular structures. The standout feature of super-resolution is its ability to visualize structures once thought impossible to discern, down to a few tens of nanometers. For researchers obsessed with detail, this is like unlocking a new realm of possibilities.
Nevertheless, super-resolution microscopy has its quirks. It often requires complex procedures and significant sample preparation, making it less straightforward than conventional methods. The setup can also be sensitive to environmental conditions.
Multiphoton Microscopy
Keeping to the theme of technological advancements, multiphoton microscopy offers another innovative approach. This technique shines a laser at a sample through a non-linear process, allowing greater depth penetration into tissues. It’s fantastic for living specimens, as it causes less damage due to lower energy requirements per photon. The key characteristic is the ability to image deeper within thicker samples, crucial for understanding complex biological interactions in situ.
However, multiphoton microscopy isn’t without its drawbacks. The systems can be costly and complex, requiring skilled operators to get the most out of the setup.
Key Components of Fluorescence Microscopy Systems
Light Sources
Light sources are the lifeblood of fluorescence microscopy. Ranging from simple light bulbs to sophisticated lasers, each type has its relevance based on the application's demands. Lasers, for example, provide specific wavelengths for targeted fluorescence, making them invaluable in most high-end setups. An advantage is the precision they offer, ensuring that only the desired fluorophores are excited. Some challenges arise with heat generation, which can hinder sample integrity if not carefully managed.
Filters and Dichroic Mirrors
No imaging system is complete without its filters and dichroic mirrors. These components ensure that only desired wavelengths of light reach the detector, eliminating background fluorescence. It’s the elegance of the design that allows researchers to filter out noise. The dichroic mirrors specifically bend light at certain wavelengths, letting others pass. This selective filtering is crucial for improving image clarity, but it can complicate the setup if multiple dyes are used, as each will require specific configurations.
Detectors and Imaging Systems
Finally, there are the detectors and imaging systems that complete the microscopy puzzle. From CCD cameras to PMTs, the choice of detector significantly impacts data quality. The key characteristic of these systems is their sensitivity—more sensitive detectors can pick up lower levels of fluorescence, which is vital when studying weak signals. Yet, this increased sensitivity comes at a cost; they may require longer exposure times, leading to potential photobleaching of samples.
The synergy between all components of fluorescence microscopy creates a powerful tool for scientific inquiry, enabling diverse applications across multiple fields.
Biological Applications
Biological applications of fluorescence microscopy are crucial as they bridge the gap between complex biological processes and their observable phenomena. This method has proven invaluable in dissecting the minutiae of cellular dynamics, mapping molecular interactions, and illuminating disease pathways. As scientists strive to unlock the secrets held within cells, fluorescence microscopy offers a window into the living world, enabling them to visualize processes in real-time.
Cellular Imaging
Studying Cell Dynamics
In the domain of cell dynamics, fluorescence microscopy shines like a beacon. It allows researchers to observe movements and changes in cells as they occur. The ability to track the behavior of live cells offers unparalleled insights into how cells respond to different stimuli, their migration patterns, and their interactions with neighboring cells. A key characteristic of this method is its capacity for real-time imaging, which helps understand dynamic processes that traditional imaging methods might miss.
However, there are a few things to keep in mind. While very effective, cellular imaging can reflect only a snapshot of events, and certain photobleaching can limit the duration of observation. Despite these challenges, the ability to monitor processes such as cell division or apoptosis in living organisms remains a boon for this field.
Visualizing Cellular Structures and Organelles
When it comes to visualizing cellular structures and organelles, fluorescence microscopy offers a unique lens. This technique can differentiate various organelles using specific fluorescent labels that emit unique colors. For instance, researchers can concurrently observe mitochondria, lysosomes, and the endoplasmic reticulum through various labeled dyes. This multifaceted approach enables a comprehensive understanding of cellular architecture.
But it's not without disadvantages. While it allows for detailed images, excessive manipulation can damage the delicate structures within cells. Furthermore, artifacts sometimes creep in from sample preparation, which can potentially lead to misinterpretations. Nonetheless, the ability to gain insights into how organelles function and interact is invaluable.
Fluorescence in Molecular Biology
Genetic Probes and Reporters
Genetic probes and reporters are at the forefront of the fluorescence phenomenon in molecular biology. These tools allow researchers to visualize specific genes and monitor their expression in real-time, offering insight into genetic functioning like never before. This characteristic is particularly beneficial for understanding gene behavior in various conditions and developmental stages.
One significant unique feature of these probes is their selectivity; they can be designed to respond to particular targets, thus minimizing background noise in imaging. However, while they provide precise information, the design process can be intricate and time-consuming, and off-target effects can sometimes muddy the results.
Protein-Protein Interactions
In studying protein-protein interactions, fluorescence microscopy provides an effective means of uncovering interactions that are crucial to numerous biological processes. Knowing how proteins collaborate helps us understand signaling pathways, enzymatic functions, and broader cellular functions. The key benefit of this method is its ability to visualize interactions in situ, revealing how proteins behave within their native environment.
Yet, this approach comes with challenges. While this imaging technique brings to light important interactions, the sensitivity to changes in the local environment may not always provide a complete picture. Appropriate controls must be employed to account for variability, ensuring that interpretations are solid.
Biomedical Research Applications
Imaging Disease Mechanisms
In medical research, imaging disease mechanisms holds tremendous significance. Fluorescence microscopy allows for studying the progression of diseases at a cellular level, shedding light on the morphological and functional changes that occur during disease states. For instance, observing how cancer cells migrate can lead to important insights into metastasis.
The unique advantage here is the ability to discern subtle changes that may indicate the onset of disease before physical symptoms manifest. However, quantifying these changes can be complex, as it requires sophisticated image analysis techniques, and biological variability might lead to varying interpretations under different conditions.
Drug Delivery Studies
When it comes to drug delivery studies, fluorescence microscopy serves as a game changer. It enables researchers to monitor how therapeutic agents are released, distributed, and metabolized within biological systems. The key characteristic that sets this method apart is its real-time tracking capability, which helps in understanding pharmacokinetics and pharmacodynamics in living systems.
Chemical and Material Science Applications
Fluorescence microscopy is increasingly pivotal in chemical and material science. This domain explores the behaviors and characteristics of substances at the molecular level, where the ability to visualize reactions and interactions opens up new avenues for research and development. The techniques offer distinct benefits, such as enhanced resolution and the capacity to track dynamic processes in real time. By harnessing these capabilities, scientists can unravel complex phenomena that traditional imaging methods might miss.
Characterization of Nanomaterials
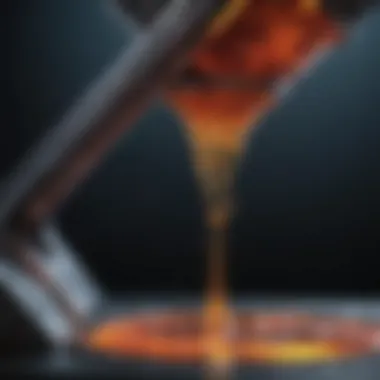
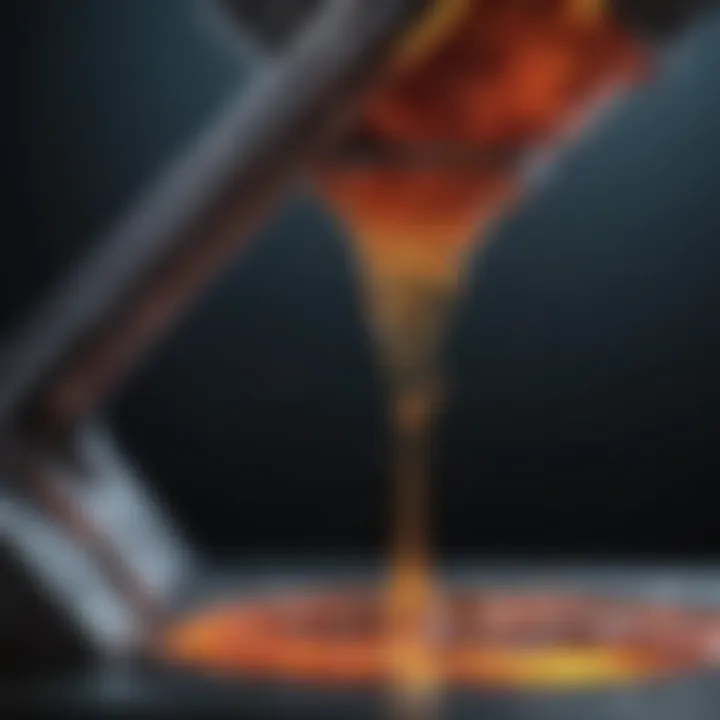
Nanomaterials have unique properties that differ significantly from their bulk counterparts, making their characterization essential for a variety of applications—from electronics to pharmaceuticals. Fluorescence microscopy plays a vital role here, allowing researchers to observe these materials under different conditions. For instance, it helps in identifying the size, shape, and distribution of nanoparticles within a sample. Thus, obtaining a detailed understanding of nanomaterials can often involve exploring their surface interactions and how they behave in various environments.
One key aspect of fluorescence microscopy in this area is its ability to detect very low concentrations of fluorescently labeled nanomaterials. This feature is fundamental in electronics and material science because it enables researchers to monitor the performance of nanomaterials when they are integrated into larger systems. Unique fluorescence tags can be attached to the nanomaterials, providing clear visual indications of their presence and behavior.
Optimization of Chemical Processes
Tracking Reaction Dynamics
The study of reaction dynamics is crucial for optimizing chemical processes. In this context, fluorescence microscopy allows researchers to monitor chemical reactions in real time, providing insights into how catalysts increase efficiency and where potential bottlenecks might occur. This specific aspect of tracking is essential because it enables scientists to elucidate reaction pathways, showing how reactants convert into products under various conditions.
A key characteristic of tracking reaction dynamics using fluorescence techniques is the high temporal resolution it offers. This timeliness is a beneficial factor for chemists who aim to refine their experimental methods by observing the immediate effects of changes in temperature, pressure, or concentration on reaction rates. Additionally, the unique feature of this approach lies in its potential for multicolor imaging, which allows the simultaneous observation of multiple species in a reaction. However, a potential disadvantage is that the photobleaching effect can limit the duration of observation, necessitating careful experimental design to acquire comprehensive data.
Analyzing Catalyst Performance
In the field of chemical reactions, understanding catalyst performance is paramount. Fluorescence microscopy shines in this regard, offering a means to visualize how catalysts operate under reaction conditions. By using fluorescent probes in conjunction with catalysts, researchers can gain insights into the active sites and pathways that are instrumental in driving reactions forward.
The ability to observe how catalysts interact with reactants visually is a notable advantage. It not only illuminates the spatial arrangement within the system but also provides qualitative and quantitative data on catalytic efficiency. Moreover, analyzing catalyst performance through this method can inform the development of more effective catalysts, bridging the gap between theoretical models and real-world applications.
Moreover, one unique aspect of this analysis is the capability to perform localized investigations. By focusing on specific regions where catalysis occurs, researchers can better understand heterogeneous catalysts and their dynamic changes during a reaction. However, as with tracking dynamics, there are drawbacks, such as the potential for interference from other imaging signals, which can complicate data interpretation.
In the realm of chemical and material science, fluorescence microscopy serves as a critical tool in expanding our understanding and improving processes and materials in ways that were once unimaginable.
The integration of fluorescence microscopy in chemical and material sciences showcases its transformative promise and establishes its crucial role in both current research and future advancements.
Environmental Applications
Fluorescence microscopy plays a pivotal role in the environmental sciences, providing a lens to examine, analyze, and understand the multifaceted interactions between pollutants and biological systems. The ability to visualize contaminants at micro and nano levels helps researchers assess ecological health and devise strategies to mitigate environmental issues effectively. Using this technology, we can track changes over time and space, which is crucial for studying dynamic environmental processes.
Monitoring Pollutants
One of the cornerstones of fluorescence microscopy in environmental science is its application in monitoring pollutants. With the growing concerns about the quality of ecosystems, the capacity to identify and quantify specific contaminants is invaluable.
Fluorescent Probes for Tracking Contaminants
Fluorescent probes have gained traction in the realm of environmental monitoring due to their sensitivity and specificity for various pollutants. These probes, which are engineered to emit light when exposed to certain wavelengths, enable researchers to pinpoint contaminants like heavy metals or organic pollutants with high precision.
A key characteristic of these probes is their ability to provide real-time data on contaminant levels. This immediacy is often a game-changer in both academic research and regulatory frameworks.
- Unique feature: The probes can be designed to respond to specific chemical environments, adapting dynamically to changes in their surroundings.
- Advantages: Their high sensitivity often allows for detection at low concentrations, which is critical for early intervention strategies in pollution control.
- Disadvantages: Though effective, the development and use of these probes can sometimes come with a higher cost and requires careful calibration to ensure accuracy.
Assessing Water Quality
Understanding water quality is another dimension where fluorescence microscopy shines. The intricacies of aquatic environments demand robust methodologies for assessing the presence of harmful contaminants and understanding their interactions with biological communities.
A significant aspect of assessing water quality through fluorescence microscopy is its ability to detect harmful algal blooms, which can release toxins detrimental to both aquatic life and human health. This method allows for the differentiation between safe and unsafe levels of toxic compounds, essential for public health and safety.
- Key characteristic: The rapid visualization of microbial activity and toxin production enables timely responses to contamination threats.
- Unique feature: Automated fluorescence microscopy systems can handle high throughput, enabling regular monitoring of water bodies.
- Advantages: Data generated can inform decision-makers about the state of water sources, contributing to public health measures.
- Disadvantages: However, interpreting fluorescence data can sometimes be complex, requiring specialized training and experience to draw accurate conclusions.
Studying Microbial Communities
Studying how microbial communities interact with pollutants is another critical application of fluorescence microscopy, shedding light on the resilience and adaptability of these communities in polluted environments. By examining these interactions, we can better understand ecosystem dynamics and the implications of contamination.
Environmental Microbiology
Environmental microbiology, through the lens of fluorescence microscopy, provides insights into how microorganisms respond to environmental stressors like pollutants. This field is crucial for understanding bioremediation processes, where certain microbes can break down harmful substances in their surroundings.
A defining feature of environmental microbiology is its focus on the functional roles of various microbial species in natural systems. By employing fluorescence techniques, researchers can visualize interactions between microbes and their environments, offering a clearer picture of ecological health.
- Key characteristic: The ability to visualize live microbial interactions allows for the study of microbial behavior in real-time.
- Unique feature: It can reveal community structures and dynamics in response to specific contaminants, enhancing our understanding of biogeochemical cycles.
- Advantages: This knowledge can inform strategies for pollution management and restoration efforts.
- Disadvantages: The complexity of microbial ecosystems can sometimes obscure straightforward interpretations of fluorescence data.
Impact of Environmental Changes
Fluorescence microscopy also serves as a critical tool for studying the impact of various environmental changes, such as climate change, on microbial systems. Understanding these impacts is essential for predicting future ecological shifts and developing adaptive management strategies.
One key aspect of this research involves assessing how altered conditions—like increased temperatures or salinity levels—affect microbial competitiveness and community resilience in polluted settings.
- Key characteristic: The capability of observing changes in microbial diversity in response to stressors allows researchers to understand the resilience of ecosystems.
- Unique feature: Time-lapse fluorescence imaging can illustrate shifts in community composition over time.
- Advantages: Data gathered can be vital for informing conservation efforts and predictive models for ecosystem health under various scenarios.
- Disadvantages: However, external factors can complicate these studies, requiring comprehensive experimental designs to isolate specific impacts effectively.
In summary, the importance of fluorescence microscopy in environmental applications cannot be overstated. From monitoring pollutants to understanding microbial communities, its insights drive efforts to protect and preserve ecological health.
Limitations and Challenges
Fluorescence microscopy, while a powerful analytical tool with significant advancements in recent years, is not without its limitations and challenges that researchers must navigate. Understanding these issues is critical, as it can affect experimental design and data interpretation. Both photobleaching and depth of tissue penetration are key factors that can greatly influence the effectiveness of fluorescent imaging in various applications. Recognizing and mitigating these challenges enables scientists to optimize their use of fluorescence microscopy in diverse fields.
Photobleaching
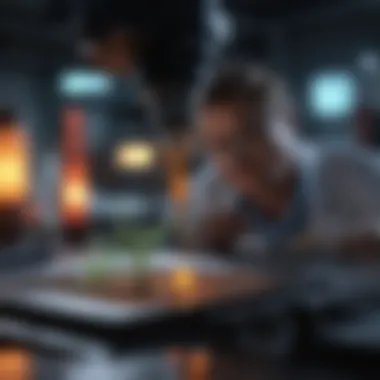
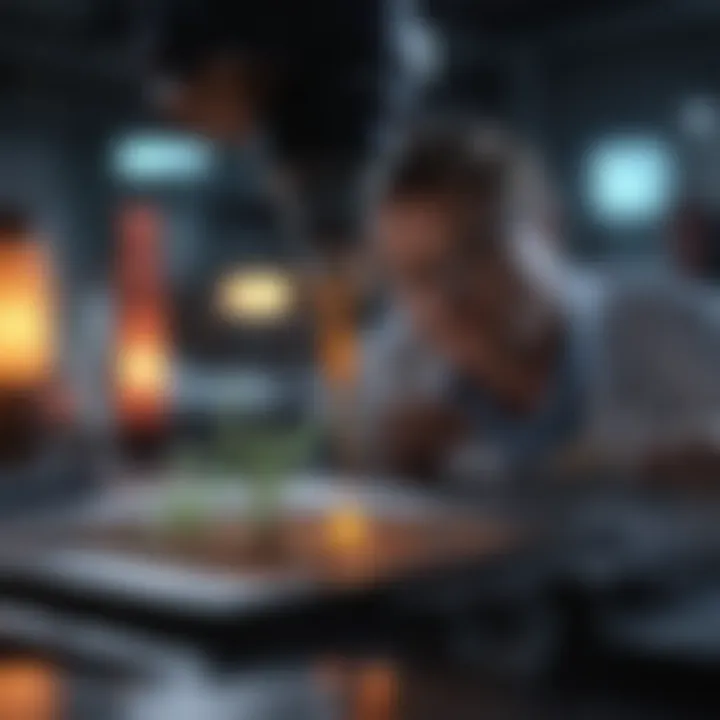
Photobleaching occurs when fluorescent dyes or proteins lose their ability to emit light after prolonged exposure to exciting light. This phenomenon can undermine an experiment’s reliability, leading to the misinterpretation of dynamic processes such as cellular activity. The sensitivity of fluorescence-based measurements to this limitation can be detrimental. To illustrate, in live-cell imaging studies, continuous observation of a fluorescently tagged protein over time may result in diminished signal intensity, distorting results. Researchers might misjudge cellular dynamics because of fading signals, which could lead them to conclude that the process under study is slower or less active than it truly is.
Preventive Measures Against Photobleaching:
To combat photobleaching, several strategies can be adopted:
- Use of Photostable Dyes: Employing newer, more photostable markers can significantly decrease the likelihood of signal degradation.
- Avoiding Over-Illumination: Minimizing the intensity and duration of the excitation light can help preserve the signal.
- Antioxidants: Chemicals like Trolox can protect the fluorophores from oxidative damage, reducing the chances of bleaches occurring.
Researchers need to be vigilant about photobleaching effects when analyzing their data—any observations made must take this potential limitation into account.
Depth of Penetration in Tissues
Another critical challenge relates to the depth of penetration of fluorescent light in tissues. Typically, the scattering and absorption of light by biological tissues hinder the effective imaging of deeper structures. In practice, this means that when working with samples like tissues or organs, only superficial layers may be visualized clearly, while deeper layers remain concealed. This limitation poses a significant hurdle for fields like developmental biology or histopathology where understanding deeper tissue interactions is crucial for drawing conclusions about physiological or pathological states.
Factors Influencing Depth Penetration:
- Excitation Wavelength: Longer wavelengths (like near-infrared) can penetrate deeper into tissues compared to shorter wavelengths.
- Sample Preparation: Different embedding and sectioning practices can improve clarity at various depths, but these often involve trade-offs in terms of sample integrity.
To conclude, both photobleaching and depth of penetration issues represent significant limitations in the application of fluorescence microscopy. Addressing these challenges through thoughtful experimental design and methodological rigor can help ensure that the vast potential of this imaging technique is effectively realized. As scientists grapple with these hurdles, continuous technological innovations may emerge to mitigate some of these issues, paving the way for more precise and detailed imaging in various scientific endeavors.
Future Directions in Fluorescence Microscopy
Fluorescence microscopy continues to evolve, shaping the landscape of multiple scientific fields in profound ways. As technology advances, the future directions in this area promise to expand the applicability and effectiveness of fluorescent techniques. Understanding these emerging trends is crucial for not only researchers but also practitioners in fields such as biology, chemistry, and environmental science. This section considers the pivotal advancements and applications likely to dominate the field in the years to come.
Technological Advancements
Integration with Other Imaging Techniques
The integration of fluorescence microscopy with other imaging modalities, such as electron microscopy and mass spectrometry, marks a significant leap forward. This hybrid approach allows for complementary insights that can hone in on cellular processes with greater precision. By combining techniques, researchers can visualize structures and analyze their chemical compositions simultaneously.
One key characteristic of this integration is the complex data sets it produces. Modern software can process this information, unveiling relationships and interactions that would otherwise remain hidden. This multifaceted view proves especially beneficial in developmental biology and oncology, where cellular dynamics are complex and need thorough investigation.
However, the integration can bring challenges. The need for sophisticated training and a steep learning curve may deter some potential users. Furthermore, maintaining optimal settings for both imaging types can complicate experimentation. Nonetheless, the advantages often outweigh the disadvantages, allowing for richer data collection and interpretation.
Nanoscale Imaging Innovations
Nanoscale imaging innovations represent another frontier in fluorescence microscopy, enhancing our ability to explore biological systems at a molecular level. Techniques like fluorescence resonance energy transfer (FRET) and super-resolution microscopy can now resolve structures at the nanometer scale, which was previously thought impossible.
The standout characteristic here is the resolution that approaches the physical limits of light, enabling scientists to observe previously invisible cellular components. This precision opens new doors in drug development and targeted therapies, where understanding a single molecule's behavior can have significant implications for treatment efficacy.
Despite these advancements, there are hurdles to overcome. Nanoscale imaging often requires expensive equipment and can involve complex preparation protocols, which may limit its routine use in some laboratories. The potential, however, cannot be overstated, as the ability to visualize interactions at this scale could radically transform our approach to both basic and applied biological research.
Emerging Applications
Single-Molecule Imaging
Single-molecule imaging is quickly gaining traction as a revolutionary approach in fluorescence microscopy. This technique focuses on visualizing individual molecules, providing a detailed look at their dynamics and interactions within a live cell context. This method allows researchers to capture fleeting events, such as protein folding or binding, that are crucial for understanding cellular mechanisms at a fundamental level.
What makes single-molecule imaging compelling is its precision. The ability to track the behavior of single biomolecules under physiological conditions provides insights that bulk measurements cannot. This technique is particularly beneficial in understanding intricate processes like signal transduction pathways and gene expression regulation.
Nevertheless, the technique can come with its own set of challenges. The requirement for high-quality fluorescent tags and specialized instrumentation means it isn’t always accessible to every research scenario. Moreover, data analysis can be intensive, requiring careful calibration and interpretation. Still, its potential for groundbreaking discoveries keeps this method in high regard.
Real-Time Monitoring in Living Systems
Real-time monitoring of biological processes in living systems stands as a fundamental advance in fluorescence microscopy. This capability enables scientists to observe how cells respond to various stimuli or changes in their environment without disrupting their natural behavior. The implications are immense, paving the way for advancements in drug discovery and regenerative medicine.
One key characteristic that sets this application apart is the immediacy of insights. As events unfold, researchers can capture and analyze processes as they happen, leading to a more nuanced understanding of pathophysiology. This immediacy enhances the relevance of findings, translating discoveries into real-world applications more swiftly.
On the flip side, implementing real-time monitoring requires a delicate balance; maintaining sample viability while ensuring clear imaging can challenge. Environmental conditions like temperature and pH must be closely monitored to avoid skewing results. Despite these hurdles, the advantages of capturing life in motion are compelling, solidifying the importance of this technique in contemporary biological research.
"Fluorescence microscopy is not just a tool; it’s a lens into the hidden world of life at the molecular level. The future holds extraordinary possibilities as integration, refinements, and innovative applications unfold."
In summary, the field of fluorescence microscopy is on the cusp of significant transformation. As researchers harness technological advancements, the potential applications of fluorescence imaging will broaden, revealing more about the complexities of life, processes, and interactions, thus enriching our understanding and capabilities across scientific disciplines.
Finale
The discussion surrounding fluorescence microscopy in this article underscores its paramount significance in enhancing scientific exploration across a variety of disciplines. As we navigate through our rapidly evolving world, the reliance on this imaging technique becomes increasingly vital. It not only enables meticulous examinations of biological, chemical, and environmental phenomena but also enriches our understanding of life at a fundamental level.
Summary of Key Insights
Fluorescence microscopy has contributed notable insights into multiple scientific fields. For instance:
- In cellular imaging, it has unveiled the intricacies of cellular dynamics and structures that were previously hidden.
- In chemical processes, it allows for the real-time tracking of reaction dynamics, pushing the boundaries of material science.
- With respect to environmental studies, fluorescent probes for tracking contaminants have become essential tools in assessing ecosystem health.
Emerging applications such as single-molecule imaging and real-time monitoring in living systems continue to define the trajectory of this tool.
The future of fluorescence microscopy is not just about technological sophistication but its adaptability and relevance to pressing scientific challenges.
The Impact of Fluorescence Microscopy on Science
The impact of fluorescence microscopy stretches far beyond mere imagery. It enables researchers to visualize processes in a non-invasive manner, fundamentally altering how scientific experiments are planned and executed. Some specific contributions include:
- Deciphering complex biological interactions: This technique allows scientists to dissect protein interactions and cellular pathways, providing insights that advance medical research and therapeutic development.
- Driving innovation in nanotechnology: In material science, it fosters innovation by characterizing nanomaterials and optimizing their functionalities, promoting advancement in fields like electronics and renewable energy.
- Addressing environmental concerns: It acts as a beacon for monitoring pollutants, hence playing a role in policy-making and conservation efforts.
Through these lenses, one can appreciate how fluorescence microscopy is not only a tool but a necessary ingredient in the culinary dish of scientific discovery, blending all fields together toward a common goal: enhancing our understanding of the universe.