Essential Protocols for Genomic DNA Isolation from Mammalian Cells
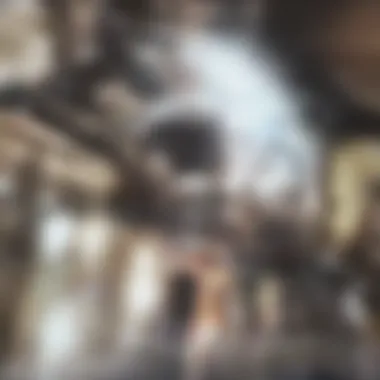
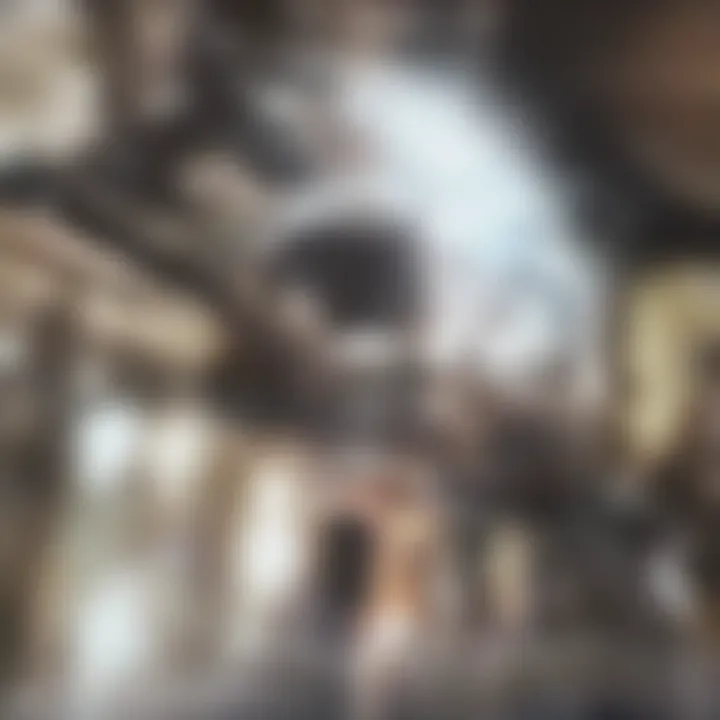
Intro
Genomic DNA isolation from mammalian cells is critical in various fields, such as genetics, molecular biology, and biotechnology. This process enables researchers to analyze and manipulate genetic material for studies related to diseases, development, and evolutionary biology. The integrity and yield of the isolated DNA can significantly affect downstream applications including PCR, sequencing, and cloning. Therefore, understanding detailed protocols and methodologies is essential for obtaining high-quality DNA.
This article will discuss the various techniques available for isolating genomic DNA from mammalian cells. Considerations regarding different cell types, lysis buffers, and purification methods will be explored. Moreover, best practices for maintaining the quality of the DNA during the isolation process will be outlined.
Research Methodology
Description of research design and approach
The research design centers on a systematic review of the methods for genomic DNA extraction. The approach taken includes a comparative analysis of different protocols, evaluating their efficacy based on yield, purity, and ease of use. Relevant literature has been examined to highlight advancements and optimizing strategies in DNA isolation. It is important to identify methodologies suited to specific research goals and biological samples.
Materials and methods used in the study
A variety of materials are utilized during the DNA isolation process. The following items are generally required:
- Cell Culture Media for growing mammalian cells.
- Lysis Buffer that disrupts cell membranes to release DNA.
- Proteinase K to digest proteins.
- Phenol-Chloroform for phase separation during purification.
- Ethanol or Isopropanol for precipitating the DNA.
- Savety Items such as gloves and goggles to ensure researcher safety.
Protocols may differ depending on the cellular type and desired yield. For instance, a commonly used protocol involves cell lysis followed by centrifugation to sediment cellular debris. The supernatant containing DNA is then subjected to purification methods such as phenol-chloroform extraction or column-based purification.
Discussion and Interpretation
Interpretation of results in the context of existing literature
When analyzing the results from genomic DNA isolation studies, it is crucial to consider factors such as yield and purity. Studies have shown that the use of certain lysis buffers can influence the quality of the isolated DNA. For example, SDS-based lysis buffers may produce higher yields compared to other buffers. Moreover, recent advancements in magnetic bead technology have provided alternatives that are quick and efficient, enhancing the overall DNA isolation process.
Implications for future research or practical applications
Reliable genomic DNA isolation techniques have practical implications in various research areas. The ability to obtain high-quality DNA allows researchers to conduct comprehensive genetic studies, contributing to knowledge in areas such as cancer research and gene therapy. Future research may focus on optimizing these protocols further, tailoring them for specific mammalian cell types or different experimental conditions. Improving the efficiency and reliability of DNA isolation will undoubtedly enhance molecular biology applications.
"High-quality genomic DNA is essential for accurate research outcomes."
Intro to Genomic DNA Isolation
The process of genomic DNA isolation is fundamental to many areas of biological research and clinical applications. Understanding how to effectively isolate DNA from mammalian cells is vital for obtaining high-quality samples. A comprehensive approach to genomic DNA isolation ensures that researchers retrieve DNA that is intact and of suitable quantity for downstream applications. This section explores the essential elements surrounding genomic DNA isolation, emphasizing its relevance in the modern scientific landscape.
Understanding Genomic DNA
Genomic DNA is the complete set of genetic material contained within an organism. In somatic cells of mammals, this DNA is organized into chromosomes, which carry genes essential for growth, development, and functioning. Extracting genomic DNA involves breaking open the cell membrane, denaturing proteins, and releasing the nucleic acids present. The purity and integrity of the isolated DNA are crucial; any contamination or degradation may hinder analysis and lead to erroneous results.
Importance of DNA Isolation
The importance of DNA isolation can not be understated. First, it allows scientists to study genetic material to uncover information about gene expression, variation, and mutations. Second, isolated DNA serves as a starting point for molecular techniques such as PCR (Polymerase Chain Reaction), sequencing, and cloning. Finally, successful DNA isolation can have implications in clinical diagnostics, genetic profiling, and biobanking. The effectiveness of these applications largely hinges on the extraction method used and the quality of the isolated material.
Applications in Molecular Biology
Genomic DNA isolation is pivotal in various applications within molecular biology. Some prominent uses include:
- Genomic Sequencing: The basis for understanding genetic blueprints and genetic disorders.
- Gene Expression Studies: To assess how different genes are expressed under varying conditions.
- Forensic Analysis: Utilizing isolated DNA samples for identification and legal investigations.
- Genetic Engineering: Enabling the manipulation and study of genes for desired outcomes.
In summary, the ability to isolate high-quality genomic DNA from mammalian cells is integral to advancing research across multiple domains in biology. This article will delve deeper into the methodologies and best practices surrounding this essential procedure.
Cell Collection and Preparation
Cell collection and preparation are critical steps in the process of isolating genomic DNA from mammalian cells. The quality of the DNA extracted largely depends on how well the cells are handled prior to the isolation process. This section discusses several key aspects that ensure optimal outcomes for genomic DNA isolation, including the selection of appropriate cell types, the cultivation conditions of these cells, and the best practices during harvesting. Each of these elements contributes towards obtaining high yields of intact DNA, suitable for subsequent analysis.
Selecting Appropriate Cell Type
The choice of cell type is significant in genomic DNA isolation. Various mammalian cells exhibit distinct characteristics that influence their growth, response to treatments, and DNA yield. In general, adherent cell lines such as HeLa cells or fibroblasts are commonly used. These cells can be subcultured efficiently and typically provide good yields of genomic DNA.
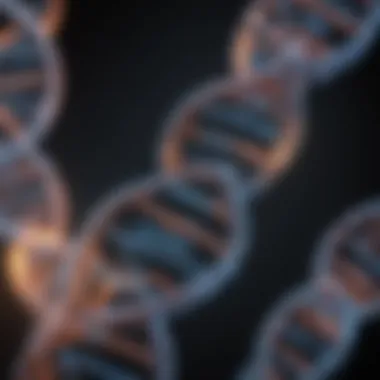
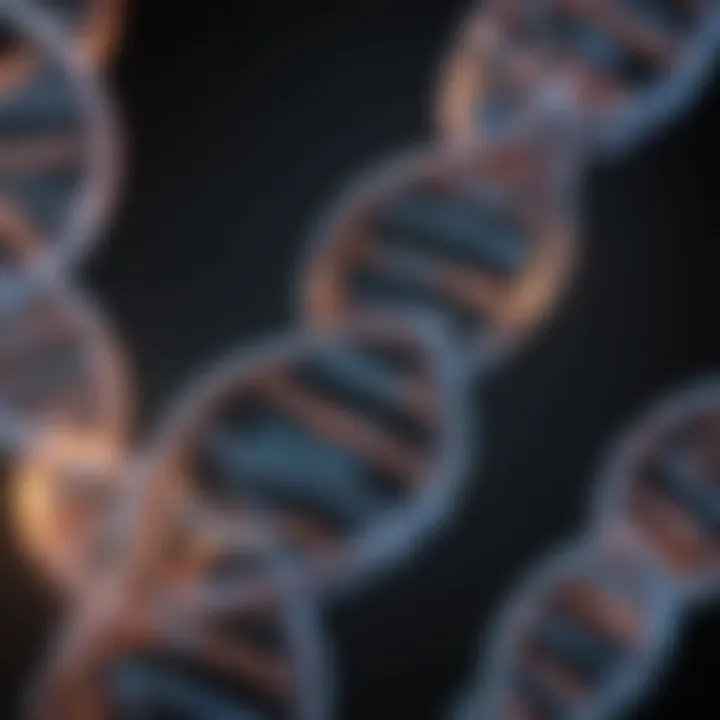
In contrast, suspension cells like lymphocytes require different handling techniques. They can be isolated from blood easily, but their collection may involve more complex procedures to ensure viability. Therefore, the first step in this process should always involve assessing the source and type of cells needed for your specific application. This will help in planning appropriate culture and harvesting methods.
Cell Culture Conditions
Proper cell culture conditions are vital for the health and growth of the cells prior to DNA isolation. Cells should be maintained in a controlled environment to ensure optimal conditions. This usually includes maintaining a specific temperature, humidity, and CO2 concentration. In addition, the type of culture medium used can affect cell growth directly.
For mammalian cells, commonly used media include DMEM (Dulbecco's Modified Eagle Medium) or RPMI 1640. Supplementation with fetal bovine serum (FBS) provides essential nutrients, ensuring cells are healthy and robust. Regular observation for contamination is essential as well. Any bacterial or fungal contamination can impact cell health and the quality of DNA extracted.
Harvesting Cells for DNA Isolation
The harvesting of cells is a critical step that should be done with care to maximize recovery and minimize damage. Cells can be harvested using methods such as trypsinization for adherent cells, which involves detaching the cells using trypsin enzyme. For suspension cells, centrifugation is the primary method for collection.
Once collected, it is crucial to wash the cells thoroughly using phosphate-buffered saline (PBS) to remove any residual culture medium and serum components. These contaminants can interfere with the DNA isolation process. After washing, cells should be counted and resuspended in an appropriate lysis buffer that facilitates the subsequent extraction of genomic DNA.
Maintaining sterility at every stage is essential to prevent contamination, which could lead to compromised DNA integrity. Overall, thoughtful consideration in cell collection and preparation significantly enhances the quality and yield of isolated genomic DNA.
Methods of Genomic DNA Isolation
The process of isolating genomic DNA is crucial in many fields of biological research. The methods of genomic DNA isolation determine not only the quality of the DNA but also its yield. Different methods have their own advantages and disadvantages. Therefore, understanding the methods available is essential for selecting the best approach for specific experiments and applications.
Phenol-Chloroform Extraction
Overview of the Procedure
Phenol-chloroform extraction is a well-established method for extracting DNA from various biological samples. In this procedure, cells are lysed, and proteins are removed using a mixture of phenol and chloroform. This process separates nucleic acids from proteins and lipids, allowing for the recovery of pure DNA. One key characteristic of this method is its efficiency in purifying high-quality DNA, which is critical for downstream applications such as PCR and sequencing. Given its long-standing use in laboratories, it remains a popular choice for many researchers.
This method significantly enhances DNA purity, making it suitable for experiments requiring high fidelity.
Advantages and Disadvantages
This technique offers several advantages. It can yield a high purity of DNA, resulting from its effective separation of nucleic acids from contaminants. However, the disadvantages include its complexity and the potential hazard of handling phenol and chloroform, which are toxic solvents. Moreover, it may require several steps, which can increase the time needed for the extraction process, making it less convenient in some situations compared to other simpler methods.
Silica Membrane-Based Isolation
Principles of the Method
Silica membrane-based isolation leverages the properties of silica to bind DNA selectively in the presence of chaotropic salts. As the sample is processed, the DNA attaches to the silica membrane while contaminants are washed away. This method has gained popularity because of its simplicity and ability to process multiple samples simultaneously. The need for specialized equipment is minimal, making it a favorable choice for many laboratories.
Step-by-Step Guide
The silica membrane-based method typically involves several straightforward steps:
- Lysis of the cells to release DNA.
- Addition of buffer containing chaotropic salts to facilitate DNA binding to the membrane.
- Washing to remove impurities.
- Elution of the DNA from the membrane for downstream applications.
This process is efficient and provides a reliable yield of DNA. Its unique feature is that it simplifies the DNA isolation procedure, making it accessible even for those with limited experience in molecular techniques.
Magnetic Bead-Based Isolation
Working Mechanism
Magnetic bead-based isolation utilizes magnetizable beads coated with specific molecules that bind DNA. When the beads are introduced after cell lysis, the DNA binds to the bead's surface. A magnet is then used to pull the beads from the mixture, allowing for the separation of DNA from contaminants. This method stands out due to its versatility and automation potential. It provides quicker isolation times and can be scaled up easily, making it attractive for high-throughput applications.
Applications in Research
Magnetic bead-based isolation is widely used in research applications such as genotyping, sequencing, and clone selection. Its flexibility allows for use with various types of samples, including cells from blood, tissue, and more. Researchers appreciate its efficiency and the high quality of DNA extracted. However, the initial cost of magnetic beads may be higher compared to traditional methods, which can deter some labs from using this technology extensively.
Lysis Buffer Composition
Components of the Lysis Buffer
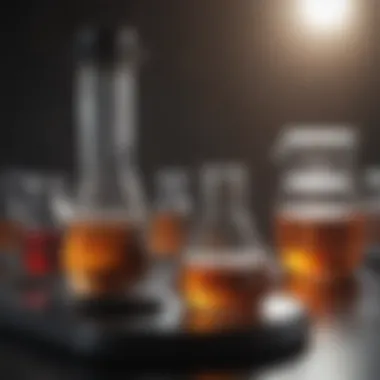
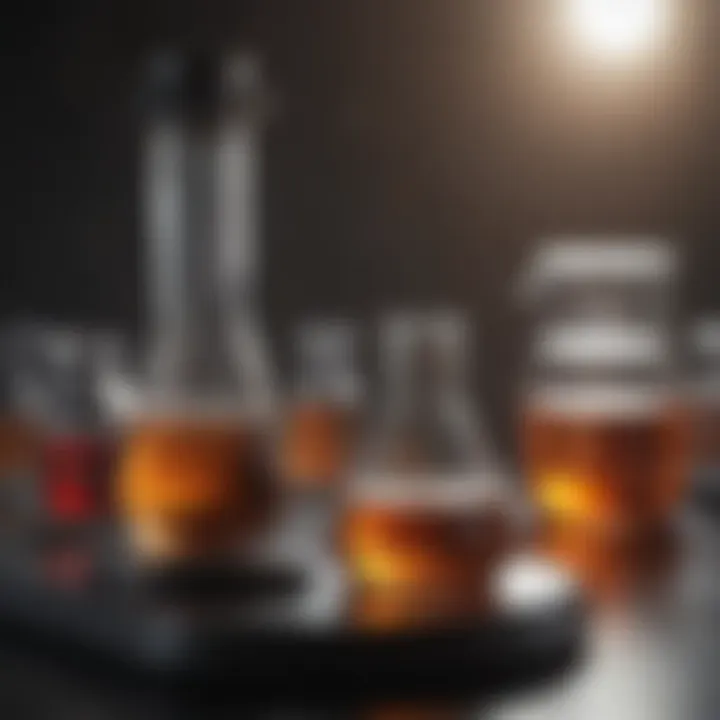
The lysis buffer is a critical component in any DNA isolation protocol. It typically contains detergents to disrupt cell membranes and enzymes to digest proteins. Common ingredients include SDS (sodium dodecyl sulfate) and proteinase K. The composition must be tailored depending on the cell type being processed. This is essential, as different cell types may require different lysis conditions to maximize DNA yield.
Optimal Conditions for Cell Lysis
To achieve effective cell lysis, specific conditions must be met. These include maintaining a suitable temperature, typically around 55-65 degrees Celsius, and ensuring the buffer's pH is optimal, usually around 7.5-8. This careful balance is essential for breaking down cellular structures without damaging the DNA itself. When optimized, the lysis buffer can significantly enhance the quality and quantity of the isolated genomic DNA.
Quality Control of Isolated DNA
Quality control of isolated DNA is a crucial part of the DNA extraction process. Ensuring the quality and purity of the genomic DNA can significantly impact subsequent experiments and analyses. Poor-quality DNA can lead to unreliable results, affecting the conclusions drawn from genetic studies, diagnostics, and therapeutic applications. Hence, a robust quality control framework is essential to validate the extraction process, providing confidence in the obtained results.
It covers two main aspects: assessing DNA concentration and evaluating DNA integrity. Each of these aspects utilizes specific techniques that allow researchers to confirm their DNA samples meet the required standards for research applications.
Assessing DNA Concentration
Spectrophotometric Analysis
Spectrophotometric analysis is a widely used method for assessing DNA concentration. This technique utilizes the absorbance of light at specific wavelengths to quantify nucleic acid concentrations. The unique capability to measure DNA concentration non-destructively makes it popular among researchers.
A key characteristic of this method lies in its simplicity and speed. Spectrophotometry can quickly provide results, helping researchers determine concentrations before proceeding to further analyses. The analysis usually occurs at 260 nm, where the absorbance is indicative of the amount of nucleic acid present in the sample.
However, this method has its limitations. While it is effective for quantifying DNA, it may also detect contaminants, such as proteins or phenol. This can lead to overestimation of the DNA concentration if the sample is not pure. Overall, spectrophotometric analysis is a beneficial and commonly employed technique, but researchers need to interpret the results cautiously.
Fluorometric Methods
Fluorometric methods are another reliable approach to assess DNA concentration. These techniques use fluorescent dyes that bind specifically to DNA, enabling quantification through fluorescence. The ability to achieve high sensitivity and specificity makes fluorometric methods a valuable choice for many research applications.
A significant advantage of this technique is its capacity to discern DNA from other substances in the sample. Fluorometric analysis yields accurate concentration measurements even in samples with low nucleic acid content. This trait is particularly useful when working with degraded samples or low-yield extractions.
Despite these benefits, the requirement for specific reagents and equipment can be a drawback. Additionally, it can be more time-consuming compared to spectrophotometric methods. Nonetheless, fluorometric techniques stand out for their sensitivity and specificity in evaluating DNA concentration effectively.
Evaluating DNA Integrity
Agarose Gel Electrophoresis
Agarose gel electrophoresis serves as a standard method for evaluating DNA integrity. This technique separates DNA fragments based on size when an electric current is applied to a gel matrix. The ability to visualize DNA allows researchers to determine whether the extracted DNA is intact or fragmented, providing insights into the sample's quality.
A key characteristic of agarose gel electrophoresis is its capability to separate DNA of varying sizes, facilitating a clear assessment of integrity. Researchers can easily distinguish between high-quality, intact DNA and degraded samples. This visualization aids in ensuring that only suitable DNA is utilized for downstream applications, enhancing the overall workflow.
The simplicity of the method adds to its appeal; however, it requires careful preparation and handling to avoid sample degradation. Additionally, interpreting results may require some experience to avoid misjudgment. Yet, agarose gel electrophoresis remains a fundamental technique for researchers to confirm DNA integrity.
Fragment Analysis Techniques
Fragment analysis techniques are sophisticated methods for evaluating DNA integrity. These approaches can assess the length and sequence of DNA fragments, providing additional information beyond simple size separation. The ability to analyze the characteristics of the DNA adds depth to quality control assessments.
These techniques are particularly beneficial for applications requiring high-resolution data, such as genotyping or forensic analysis. The sensitive nature of fragment analysis allows researchers to detect even small amounts of DNA damage or degradation.
However, the complexity involved can be disadvantageous. These methods often demand specialized equipment and technical expertise. Additionally, the cost may be higher than other quality control approaches. Despite these challenges, fragment analysis holds significant value in ensuring the integrity of isolated DNA, particularly when precise DNA characteristics are essential.
Quality control is an integral aspect of DNA isolation. Accurate assessment of concentration and integrity not only validates the extracted DNA but also safeguards the success of future experiments.
Troubleshooting Common Issues
Troubleshooting common issues during genomic DNA isolation is an essential aspect of ensuring reliable results. Effective troubleshooting can address several concerns that arise during the extraction process. It helps to enhance yield, purity, and integrity of the isolated DNA. Focusing on these challenges aids researchers in optimizing their workflows and minimizes the risk of conducting flawed experiments.
Low DNA Yield
Low DNA yield can result from various factors, which include cell type, lysis efficiency, and extraction method. Researchers might find that the expected quantity of DNA is not attained, which can hinder further experiments. To troubleshoot low yield, several steps can be taken:
- Increase cell number: Utilize more cells prior to the isolation process to maximize the amount of usable DNA.
- Optimize lysis conditions: Adjusting the lysis buffer's composition can enhance the release of DNA from the cells. Ensuring the buffer works effectively for the specific cell type will improve results.
- Refine extraction method: Using more efficient techniques, such as silica-based methods or magnetic bead isolation, can yield better results compared to traditional methods.
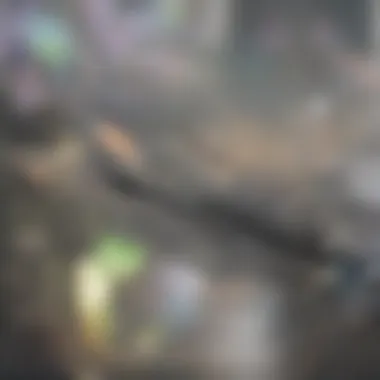
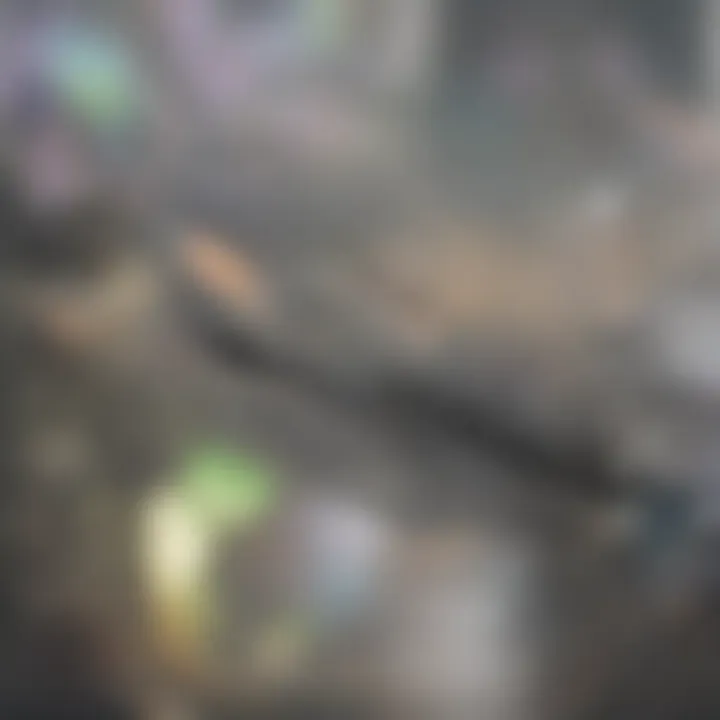
Overall, understanding why low yields occur plays a major part in improving protocols.
Contaminants in Isolated DNA
Contamination can severely impact the purity of isolated DNA. Contaminants may include proteins, salts, or phenolic compounds that interfere with downstream applications. Several strategies can help address this issue:
- Precipitation with alcohol: Using ethanol or isopropanol to precipitate DNA can help remove impurities. This step should be done carefully to avoid losing desired DNA fragments.
- Use of purification columns: Employing columns designed specifically for DNA purification can help remove contaminants effectively. Silica columns are quite effective in this regard.
- Verifying the purity: Utilizing spectrophotometric analysis to assess the A260/A280 ratio is a quick way to check for protein contamination. A ratio between 1.7 and 2.0 is generally considered acceptable.
By addressing contaminant presence, researchers can ensure the DNA's suitability for analysis and experimentation.
Degraded DNA Samples
DNA degradation can significantly impact the integrity of isolated samples. Factors contributing to degradation could be excess exposure to heat, inappropriate storage, or suboptimal lysis conditions. Here are some recommendations to handle degraded samples:
- Use of RNase-free reagents: Ensuring all reagents are free from nucleases can prevent RNA contamination. RNases can degrade DNA during the extraction process.
- Quickly process samples: Reducing the time cells are exposed to unfavorable conditions will help maintain DNA integrity. Perform extraction as soon as possible after cell harvest.
- Storage conditions: Store isolated DNA samples at -20°C or -80°C to minimize degradation over time. Working with samples on ice during procedures can also help prevent temperature-related breakdown.
Taking these precautions can greatly reduce the risk of working with compromised DNA samples and facilitate more successful experiment outcomes.
Storage and Preservation of Isolated DNA
The storage and preservation of isolated genomic DNA is crucial for maintaining its integrity and usability in future experiments. Once DNA is isolated, it is vulnerable to degradation and contamination. Thus, understanding the right conditions for storage, as well as techniques for long-term preservation, is vital for researchers.
Proper storage ensures that DNA samples retain their quality over time. This has several implications for experiments, including reproducibility, accuracy, and reliability. Maintaining the purity of DNA is essential for downstream applications such as PCR, sequencing, and cloning. This section provides detailed information about critical storage conditions and preservation methods that will safeguard DNA integrity.
Optimal Storage Conditions
Isolated genomic DNA requires specific conditions to remain stable and functional. The primary factors that affect DNA stability include temperature, pH, and the presence of contaminants. Here are some optimal conditions to consider:
- Temperature: Store DNA samples at -20°C or -80°C. Long-term storage at these low temperatures minimizes enzymatic activity that can lead to degradation. For short-term use, 4°C is acceptable but should not exceed a few weeks.
- Buffer: DNA should be resuspended in a buffer, such as TE buffer (Tris-EDTA), which helps maintain pH and protect against degradation. The ideal pH range is typically around 7.5 to 8.0 to ensure stability.
- Aliquoting: To prevent repeated freeze-thaw cycles, which can damage DNA, aliquoting samples into smaller volumes is recommended. This practice helps ensure that only the necessary amount is thawed for use.
- Avoid Contaminants: Ensure that samples are free from contaminants such as proteins, phenol, or ethanol. These substances can inhibit subsequent reactions and reduce DNA quality.
"Proper storage conditions are essential to prevent the degradation and loss of genomic DNA integrity in biological research."
Long-term Preservation Techniques
To ensure the longevity of genomic DNA, various preservation techniques can be employed:
- Lyophilization (Freeze-Drying): This technique removes water from DNA samples, reducing the risk of hydrolytic damage. Lyophilized DNA can be stored at room temperature for extended periods. However, care must be taken when rehydrating the samples to ensure they are fully dissolved.
- Cryopreservation: For samples that require long-term storage, cryopreservation in liquid nitrogen (-196°C) is an effective method. This technique preserves cell viability and DNA integrity, but the infrastructure for such storage might not be available in all labs.
- Polymerase Inhibitors: The addition of substances that inhibit DNases (like proteinase K) during storage can decrease the likelihood of DNA degradation. This method is particularly valuable in preserving samples collected from diverse environments or biological contexts.
Finale and Future Directions
The conclusion of this article provides an essential overview of the importance of genomic DNA isolation and what is to come in the field. As researchers continue to explore the complexities of mammalian cells, advancements in DNA isolation methods will become even more crucial. The effectiveness of isolation protocols impacts the reliability of subsequent analyses, including sequencing and gene expression studies. Therefore, choosing the right method and understanding its nuances can greatly enhance experimental success.
Future directions in DNA isolation will likely focus on increasing efficiency and minimizing contamination. Automation in DNA extraction holds promise, as it can speed up the process and reduce human error. Additionally, the integration of novel materials in isolation techniques may help overcome existing limitations in yield and purity.
Moreover, as new technologies emerge in genomics, the demand for refined DNA isolation methods will persist. Innovative approaches, such as the use of nanomaterials or microfluidics, may revolutionize the standard practices in the near future. Keeping abreast of these trends will be vital for researchers aiming to maintain high standards in their work.
"The future of genomic DNA isolation is not just about strategies; it’s also about integrating technology that can enhance precision and yield."
Summary of Key Takeaways
The key points regarding genomic DNA isolation are essential for informed practice. First, the choice of method can significantly affect the quality of DNA obtained. Each method discussed, such as phenol-chloroform extraction or silica membrane-based approaches, has its advantages and limitations. Understanding these subtleties will help researchers select the most suitable protocol based on their specific needs.
Second, quality control is paramount. Assessing DNA concentration and integrity through various techniques ensures the suitability of isolated DNA for future applications. Implementing robust quality checks reduces the likelihood of experimental failure.
Finally, proper storage and preservation of isolated DNA cannot be overlooked. Implementing optimal storage conditions ensures longevity and stability, further supporting experimental groundwork.
Emerging Trends in DNA Isolation
Emerging trends in DNA isolation point to exciting advancements that hold potential for improved methodologies. One prominent trend is the rise of automated systems that provide higher throughput and replicate isolation processes efficiently. These systems also mitigate the risk of human error and contamination, which is vital for research integrity.
Another trend is the application of machine learning algorithms to optimize extraction processes. By analyzing past data on yields and purity, these systems could provide tailored protocols corresponding to specific cell types or experimental conditions.
Additionally, the use of biodegradable and environmentally friendly materials in lysis buffers and isolation kits is gaining traction. As ecological concerns become more pressing, developing sustainable methods for DNA extraction aligns with broader research goals.
Overall, this convergence of technology and sustainability positions genomic DNA isolation on a path of significant evolution. Staying informed about these trends will allow researchers to adapt to and leverage new opportunities in the realm of molecular biology.