Heart Tissue Engineering: Advances and Future Directions
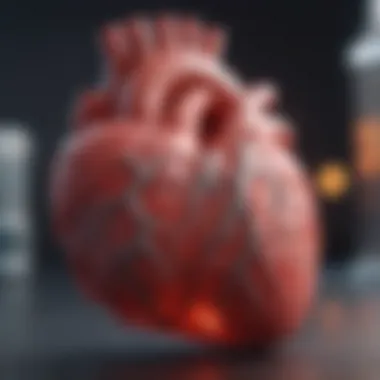
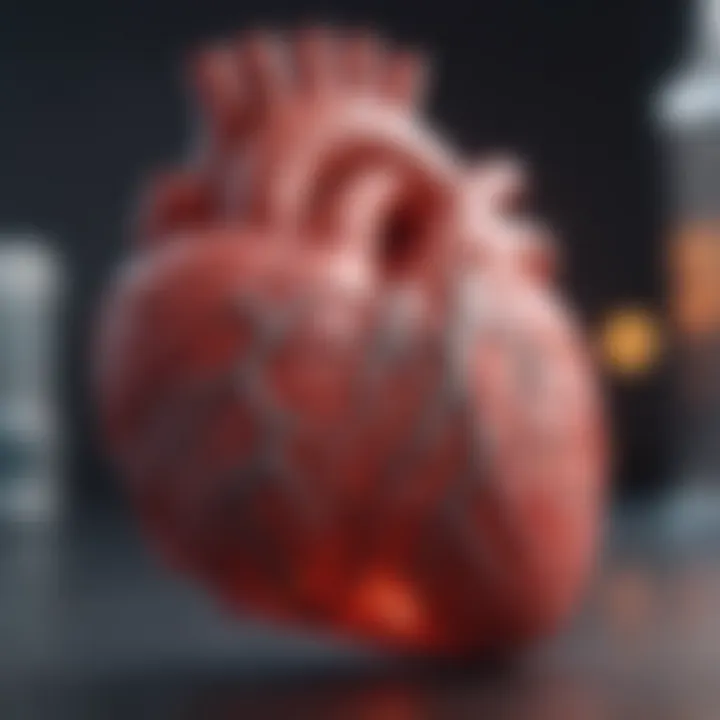
Intro
Heart tissue engineering has emerged as an essential discipline within regenerative medicine. It combines knowledge from various fields like biology, materials science, and engineering to address the complexities of cardiovascular diseases. This discussion seeks to explore the various advancements that have been made, the challenges that still exist, and the optimistic future ahead in this field. Understanding how these components interact will be crucial for both current applications and future innovations in patient care.
Research Methodology
Description of research design and approach
The design of research in heart tissue engineering often utilizes a multidisciplinary approach to develop viable cardiac tissues. This involves collaboration between engineers, biologists, and clinicians. Many studies emphasize in vitro models for testing biocompatibility and functionality of engineered tissues. The aim is to create structures that can mimic the natural heart's functions and response to mechanical stimuli.
Materials and methods used in the study
Key materials include stem cells, which are derived from various sources such as induced pluripotent stem cells or adult stem cells. The scaffolds are typically constructed from biodegradable polymers, hydrogels, or a combination of both. These materials provide a framework for cell attachment and growth. Technologies such as 3D bioprinting have revolutionized how these scaffolds are produced, offering precise control over structure and composition.
Discussion and Interpretation
Interpretation of results in the context of existing literature
Recent studies have reported promising results in using engineered cardiac tissues for treatment of heart diseases. For example, some methods have shown the ability to improve contractility when implanted in animal models. These findings suggest that there is potential for clinical application, yet significant work needs to be done. Many challenges, such as immune rejection and integration with host tissues, persist.
Implications for future research or practical applications
Future research should focus on overcoming existing obstacles in heart tissue engineering. Advancements in biomaterials, stem cell technology, and bioprinting will enhance the functional properties of engineered tissues. Clinical trials will play a crucial role in validating these techniques, allowing them to be translated into standard therapeutic approaches.
By continuing to innovate and adapt, heart tissue engineering holds significant promise in transforming cardiovascular care.
End
Preface to Heart Tissue Engineering
Heart tissue engineering stands as a pivotal component of regenerative medicine, with significant implications for the treatment of cardiovascular diseases. The intricate nature of heart tissue presents unique challenges and opportunities, making it imperative for researchers and practitioners to push the boundaries of biomedical science. Here, we shall delve into the profound importance of this field, not only in repair and regeneration of heart tissues but also in enhancing the quality of patient care.
As the burden of heart diseases escalates worldwide, innovative solutions are critically needed. Heart tissue engineering offers the promise of creating functional cardiac tissues that can be used to replace damaged areas in the heart. This approach integrates various scientific disciplines—from biology to materials science and engineering. The benefit lies in its potential to restore normal heart function with less risk of rejection compared to traditional transplant methods.
Nevertheless, the complexity and multifaceted nature of heart tissue engineering warrant thorough understanding. Factors such as cell types, materials, and biocompatibility play crucial roles. Researchers are continuously faced with questions regarding how to effectively mimic the heart's natural environment in laboratory settings. Addressing these considerations not only aids research but also fosters advancements that could lead to successful clinical applications. Thus, the exploration of heart tissue engineering is essential in the quest to find more effective therapies for heart disease, ultimately improving patient outcomes.
Definition and Scope
Heart tissue engineering involves the development of biological substitutes that can restore, maintain, or improve the function of damaged heart tissues. It encompasses a variety of techniques and materials, relying on biological, physical, and chemical principles. The scope of this field extends beyond simple tissue repair; it integrated smart biomaterials, stem cell therapy, and advanced bioprinting technologies to generate functional cardiovascular tissues.
The intersection of these areas creates a vast landscape of research and application. As heart tissue engineering evolves, its definition expands to include a diverse array of methodologies aimed at addressing cardiac conditions.
Historical Context and Milestones
The journey of heart tissue engineering can be traced back to several significant milestones that underscored its development as a field. In the early 20th century, researchers began investigating the use of biological materials to repair cardiac tissues, but substantial progress was slow.
The notable breakthrough came with the discovery of stem cells in the late 20th century. This finding expanded possibilities, inspiring further research into cell-based therapies. In the early 2000s, the development of biomaterials specifically designed for cardiac applications allowed for the creation of scaffolds that mimic the mechanical properties of heart tissues.
Recent advancements in 3D bioprinting technology mark another pivotal milestone. This innovative technique has enabled the precise deposition of cells and materials, allowing for the creation of more complex tissue structures. Overall, the historical evolution of heart tissue engineering showcases the remarkable strides made in understanding and manipulating biological tissues for therapeutic purposes.
Fundamental Concepts
Understanding the fundamental concepts of heart tissue engineering is essential for grasping the complex interplay of various elements that contribute to the field. This section discusses the structure of heart tissue, the pivotal cell types involved, and the role of the extracellular matrix. Each component holds significance, as they collectively contribute to the growth and repair of cardiac tissues, which are crucial for effective treatment strategies against heart diseases.
The Structure of Heart Tissue
The heart is a sophisticated organ, integral to the circulatory system. It consists of several layers - epicardium, myocardium, and endocardium - each serving distinct functions. The myocardium is the primary layer, composed of cardiomyocytes, which are responsible for cardiac contractions. Essentially, this structure facilitates the effective pumping of blood throughout the body.
Moreover, heart tissue's structural organization influences its mechanical properties and functionality. Understanding this arrangement is critical for replicating the heart's natural function in engineered solutions. If tissue engineering can accurately mimic this structure, it may lead to improved functional outcomes after heart repair procedures.
Cell Types Involved
Within heart tissue engineering, three main cell types play significant roles: cardiomyocytes, fibroblasts, and endothelial cells. Each cell type contributes uniquely to the development and functionality of engineered heart tissues.
Cardiomyocytes
Cardiomyocytes are the heart muscle cells responsible for contractions. They contain specific proteins, such as actin and myosin, which are crucial for contractility. This property makes cardiomyocytes a favored choice in heart tissue engineering. They can regenerate damaged tissue
However, obtaining an adequate supply of functional cardiomyocytes remains a challenge. Their unique feature of having a limited replicative capacity means that scaling their production for clinical applications is difficult.
Fibroblasts
Fibroblasts are connective tissue cells that contribute to structural integrity in the heart. They produce extracellular matrix components, which support cardiomyocytes. The critical characteristic of fibroblasts is their ability to promote wound healing, making them important in tissue regeneration.
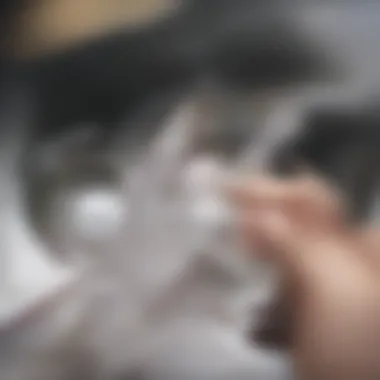
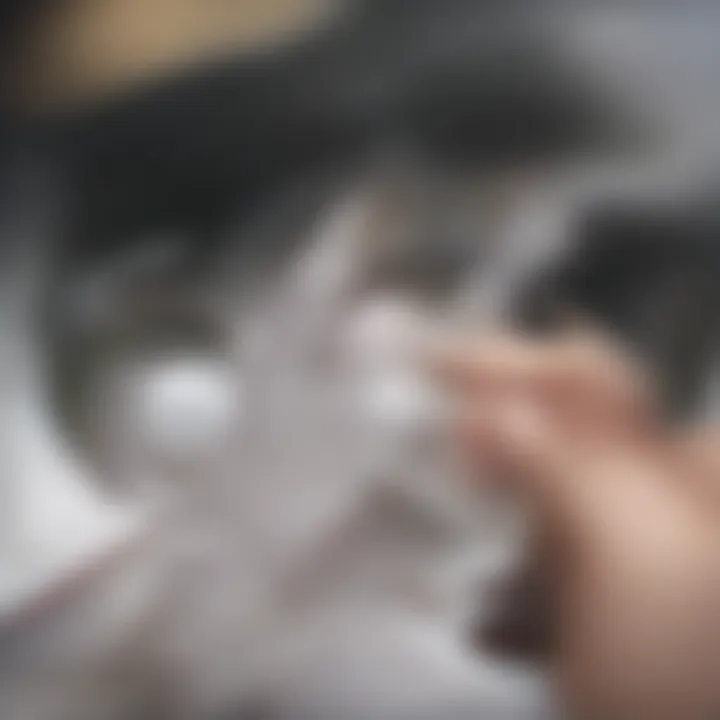
Nonetheless, the presence of too many fibroblasts can lead to fibrosis, which may impede heart function. Therefore, striking a balance in their application is essential for successful outcomes in tissue engineering.
Endothelial Cells
Endothelial cells line the heart's blood vessels and are responsible for maintaining vascular health. Their key role in sustaining the supply of nutrients and oxygen to cardiomyocytes illustrates their importance in heart tissue engineering.
Their unique advantage lies in promoting vascularization within engineered tissues, which is essential for long-term survival and integration. However, challenges persist in ensuring adequate alignment and function of vascular networks in engineered constructs.
Extracellular Matrix Composition
The extracellular matrix (ECM) is crucial in heart tissue engineering. It provides structural support and biochemical signals that guide cellular functions. The composition of the ECM varies, containing proteins like collagen, elastin, and glycoproteins. Understanding this composition is vital for replicating heart tissue function.
"Heart tissue engineering is not just about cells; it is equally about the environment they reside in."
The exploration of these concepts provides a solid foundation for addressing the advances, challenges, and future directions in the field.
For further reading, visit Wikipedia - Heart, Britannica - Heart, and Reddit - Tissue Engineering.
Cell Sources for Heart Tissue Engineering
Cell sources are fundamental to heart tissue engineering. The ability to generate functional cardiac tissue rests heavily on the selection of appropriate cell types. These cells must not only possess the capability to form heart tissue but also integrate effectively with existing cardiac structures. Understanding various cell sources allows researchers to innovate and potentially improve treatment outcomes for heart disease.
Stem Cells
Stem cells play a pivotal role in heart tissue engineering due to their unique characteristics and versatility. They have the ability to differentiate into various cell types, making them highly valuable for creating functional heart tissue.
Embryonic Stem Cells
Embryonic stem cells are derived from early-stage embryos. A notable aspect of these cells is their pluripotency, which means they can differentiate into any cell type, including cardiomyocytes. This characteristic makes them a powerful choice for heart tissue engineering.
Key Benefits: Embryonic stem cells can proliferate indefinitely in culture, providing a potentially limitless supply. Their capacity to develop into heart cells supports advancements in generating cardiac tissue for research and therapeutic purposes.
However, there are disadvantages. The use of embryonic stem cells raises ethical concerns related to embryo destruction. Moreover, their potential to form teratomas, or tumor-like structures, poses challenges in clinical applications.
Induced Pluripotent Stem Cells
Induced pluripotent stem cells are adult somatic cells reprogrammed to an embryonic-like state. This technique allows ordinary cells, such as skin or blood cells, to return to a pluripotent state.
Key Value: The foremost advantage of induced pluripotent stem cells is their ethical acceptance. Since they are generated from adult tissues, they do not involve embryonic destruction. Additionally, they can be created from the patient’s own cells, which lowers the risk of immune rejection.
They also maintain the potential for unlimited proliferation and differentiation into cardiomyocytes, mirroring embryonic stem cells in functionality.
Nonetheless, a limitation is their efficiency in reprogramming, which can be lower compared to embryonic stem cells. Furthermore, there are concerns regarding their genetic stability and potential tumorigenesis depending on the reprogramming methods used.
Cardiac Progenitor Cells
Cardiac progenitor cells are another source that has gained attention in heart tissue engineering. Unlike pluripotent stem cells, these cells are more specialized and are committed to forming heart tissues. They can be isolated from various sources, including the heart itself.
Benefits of Cardiac Progenitor Cells: They are less likely to exhibit tumor formation compared to pluripotent stem cells. The risk of immune rejection is also lower when using auto-logous progenitors, increasing their applicability in personalized medicine.
However, the availability of cardiac progenitor cells is limited. Harvesting them can sometimes require invasive procedures. There is also a need for further research to fully understand their differentiation potential and long-term functionality in engineered tissues.
Further exploration of these cell sources can lead to innovative solutions in cardiac repair and regeneration.
Scaffold Materials in Tissue Engineering
Scaffold materials play an essential role in heart tissue engineering. They provide structural support for cell attachment, growth, and differentiation. The right scaffolding can mimic the native extracellular matrix, promoting the necessary environment for cardiovascular cells to thrive. In this section, we will explore the two principal types of scaffolds—natural and synthetic—and discuss vital factors like biocompatibility and biodegradability that influence their selection.
Natural Scaffolds
Natural scaffolds are derived from biological sources. Common materials include collagen, gelatin, and alginate. These materials offer several advantages.
- Biological Compatibility: Natural scaffolds are generally well-accepted by the body. Their composition resembles native tissues, reducing the risk of rejection.
- Bioactive Properties: They possess intrinsic bioactivity. This facilitates cellular attachment and proliferation, fostering tissue regeneration.
- Degradability: Natural scaffolds often degrade at rates aligned with tissue formation, providing a timely support structure as new tissue is generated.
However, they also present challenges. The mechanical properties of some natural materials may not meet the demands for heart tissues, which often undergo significant strain. Furthermore, variability in source material can lead to inconsistencies in scaffold performance.
Synthetic Scaffolds
Synthetic scaffolds are engineered from materials like polylactic acid (PLA), polycaprolactone (PCL), and polyethylene glycol (PEG). The advantages of synthetic scaffolds are significant.
- Controlled Properties: Their mechanical and degradation properties can be finely tuned to match specific requirements of heart tissues.
- Scalability: Synthetic materials can be produced in large quantities with uniform characteristics. This consistency is valuable for clinical applications.
- Customization: Researchers can incorporate various biological signals to enhance cell behavior, tailoring the scaffold for specific applications in heart repair.
Nevertheless, synthetic materials can provoke a negative immune response and may not provide the same level of cell signaling seen in natural scaffolds.
Biocompatibility and Biodegradability
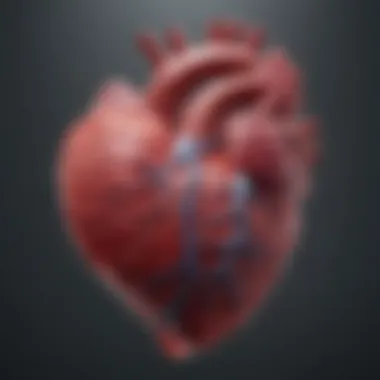
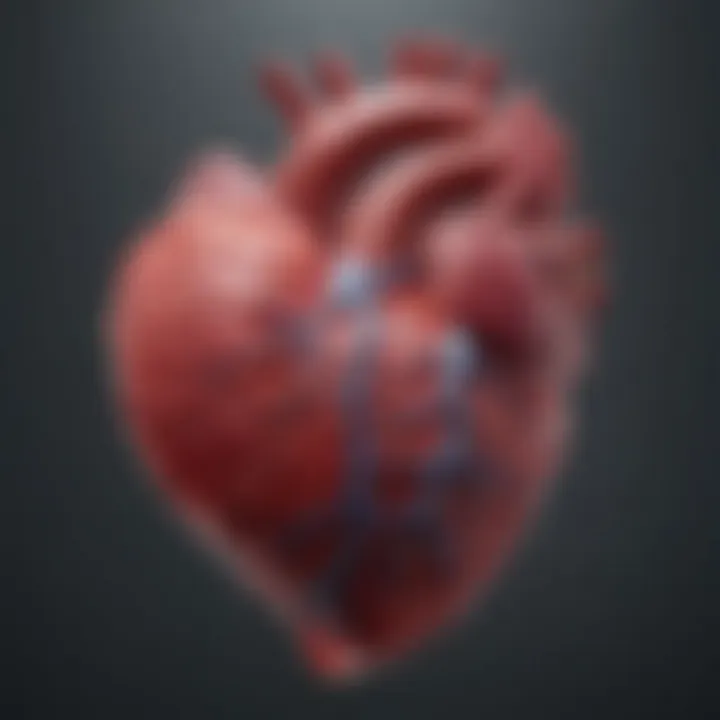
Biocompatibility is crucial when selecting scaffold materials. It ensures that the scaffold does not induce an adverse immune response when implanted. The following aspects are important to consider:
- Material Selection: Both natural and synthetic materials should undergo thorough testing for biocompatibility.
- Surface Modification: Techniques like coating or chemically altering the scaffold surface can help enhance interactions with cells, improving biocompatibility.
Biodegradability also plays a critical role in ensuring that scaffolds dissolve at a rate conducive to tissue growth. If a scaffold degrades too quickly, it could lead to structural failure. Conversely, a scaffold that remains for too long could cause inflammation or scarring. Successful heart tissue engineering relies on a balanced approach, where the selected scaffold promotes optimal cellular growth while providing transient support during the regeneration process.
Ultimately, scaffold materials are the backbone of heart tissue engineering. Their composition significantly affects the success of tissue regeneration, influencing both biological and mechanical outcomes.
3D Bioprinting Technologies
3D bioprinting technologies are an essential component of heart tissue engineering. This innovative method allows for the precise placement of cells and biomaterials to create engineered tissues that can mimic natural heart structures. With the rising incidence of cardiovascular diseases, these technologies are becoming increasingly relevant. They offer a pathway toward personalized medicine by enabling the creation of custom tissues tailored to individual patient needs, addressing critical issues in heart repair and regeneration.
Techniques and Approaches
3D bioprinting involves several techniques that facilitate the fabrication of complex tissue structures. Among these methods, extrusion-based bioprinting is one of the most popular. This approach uses a syringe-like nozzle to deposit bioinks, composed of living cells and biomaterials, layer by layer to form a 3D structure.
Another method is laser-assisted bioprinting, which employs focused laser beams to precisely position cells onto a substrate. This technique minimizes damage to the cells during the printing process, ensuring higher viability post-printing.
Furthermore, inkjet bioprinting uses thermal or piezoelectric mechanisms to eject droplets of cell-laden bioink onto a surface, allowing for rapid and high-resolution fabrication of 3D constructs. These techniques allow researchers to manipulate various parameters, such as cell types and growth factors, fostering an environment conducive to tissue formation.
Applications in Cardiac Models
The application of 3D bioprinting in cardiac models holds great promise for advancing heart tissue engineering. Researchers are now able to create realistic in vitro models of heart tissue that can be used to study disease mechanisms, drug responses, and toxicity.
These bioprinted cardiac models can contain a complex arrangement of cardiomyocytes, fibroblasts, and endothelial cells, resembling the architecture of natural heart tissue. Thus, they can be utilized for:
- Drug Testing: By providing a more relevant model for testing pharmaceuticals, these structures can help predict drug efficacy and safety more accurately than traditional two-dimensional cultures.
- Disease Modeling: Bioprinted tissues can be designed to replicate specific cardiovascular diseases, enabling researchers to explore the underlying pathophysiology and test potential treatments more effectively.
- Regenerative Therapies: In the long-term, successful integration of bioprinted tissues into host myocardial tissues could be a breakthrough in treating heart failures, offering hope for restoration of function and repair of damaged areas.
Ultimately, 3D bioprinting represents a convergence of engineering, biology, and medicine, promising transformative impacts in cardiac care and regenerative therapies.
Current Applications in Heart Repair
Heart repair through tissue engineering is at a pivotal point in modern medicine. With advancements in cell biology, materials science, and engineering, the applications of these technologies have the potential to significantly impact cardiovascular health. This section delves into applications focusing on acute myocardial infarction and chronic heart failure. Each subsection highlights current methodologies, benefits, and considerations in clinical settings.
Acute Myocardial Infarction
Acute myocardial infarction (AMI), commonly known as a heart attack, results from a sudden blockage of blood flow to the heart. The aftermath often leads to tissue damage and impaired heart function. Current applications in heart tissue engineering aim to repair this damage and restore functionality.
Engineered tissues for AMI can be designed to replace damaged myocardial tissues. Techniques typically involve using stem cells, such as induced pluripotent stem cells (iPSCs), which can differentiate into cardiomyocytes. By injecting these cells or applying engineered patches to the damaged area, researchers aim to enhance repair by promoting cell survival and integration with existing tissues.
"The use of stem cell therapies in AMI demonstrates promising results in improving cardiac function and reducing scar formation."
Challenges remain, such as ensuring adequate vascularization and oxygen supply to the newly formed tissues. Without sufficient blood flow, engineered tissues cannot survive or function properly. Additionally, considerations regarding the scaling up of these technologies for clinical use are critical. Regulatory pathways for testing and approval can be lengthy and complex, potentially delaying patient access to these innovative therapies.
Chronic Heart Failure
Chronic heart failure (CHF) is a debilitating condition, leading to progressive cardiac dysfunction over time. It poses a substantial challenge for current medical treatments. The integration of tissue engineering offers a dynamic solution to tackle the limitations faced with standard interventions.
Engineered cardiac patches are one of the pioneering approaches in managing CHF. These patches are developed using biocompatible materials and living cells, which can be implanted onto the heart's surface. The goal is to augment the heart's contractile ability and improve pumping efficiency. Research shows that these patches can also secrete growth factors that aid in the regeneration of native heart tissue.
Moreover, bioprinting is being explored as a method to create complex tissue architectures that mimic the natural structure of heart tissue. This approach holds promise for developing personalized treatments tailored to individual patient anatomies, enhancing the efficacy of heart repair strategies.
In summary, the applications of heart tissue engineering in both acute myocardial infarction and chronic heart failure offer a transformative potential for treating cardiovascular diseases. While the technologies are advancing, the focus must remain on overcoming existing challenges, ensuring patient safety, and facilitating regulatory approval to enable widespread clinical adoption.
Challenges in Heart Tissue Engineering
Heart tissue engineering holds significant promise for regenerating cardiac tissues damaged by disease or injury. However, it also faces many challenges that can hinder its progress and application. Understanding these challenges is essential to advance the field and improve patient outcomes. In this section, we delve into the intricate issues of vascularization, immune response, and integration with host tissue, which are critical factors influencing the success of engineered heart tissues.
Vascularization Issues
A major challenge in heart tissue engineering is the establishment of effective vascular networks within engineered tissues. The heart is a highly vascularized organ, requiring an adequate supply of oxygen and nutrients delivered through blood vessels. Without a functioning vascular system, even the most successfully engineered cardiac tissue may not survive beyond a few days after implantation.
Existing methods for achieving vascularization in engineered constructs often fall short. Strategies such as incorporating growth factors or using pre-vascularized tissue patches have shown some success, but they lack scalability and may not always translate well in vivo. Researchers are exploring techniques like the use of endothelial cells to create capillary networks within scaffolds, but a complete and functional vascular system remains elusive.
Effective vascularization is crucial for the survival and function of engineered heart tissues. Challenges must be addressed to enhance outcomes in regenerative therapies.
Immune Response Considerations
Another critical element is managing the immune response to implanted tissues. The body’s immune system may recognize engineered tissues as foreign and mount an immune response against them. This reaction can lead to inflammation, tissue necrosis, and eventual rejection of the implanted construct.
To mitigate these immune responses, researchers are investigating various approaches, including the use of immunosuppressive agents and designing biomaterials that mimic the native extracellular matrix. However, these strategies must be balanced with the need to maintain overall immune function. Finding a way to promote tolerance without severely compromising the immune system is an ongoing challenge.
Integration with Host Tissue
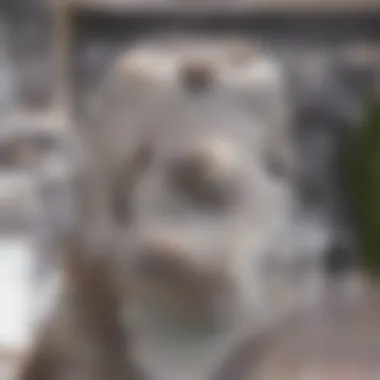
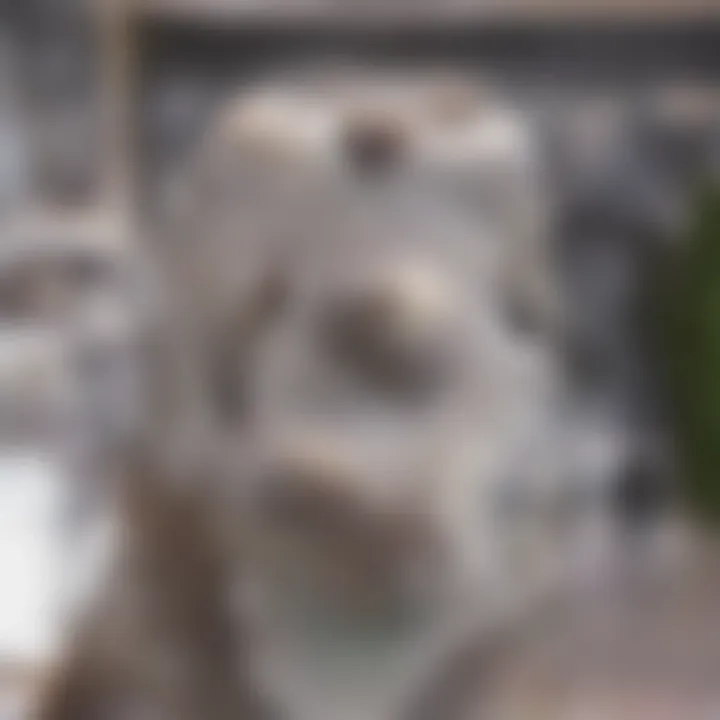
For heart tissue engineering to be successful in clinical applications, engineered tissues must integrate seamlessly with the surrounding host tissue. Poor integration can lead to functional discrepancies and complications post-implantation. The engineered tissue must not only maintain functional viability but also synchronize with the host's electrical and mechanical activity.
Factors influencing integration include the biochemical cues from host tissue, the physical properties of the scaffold, and the type of cells incorporated. Current research focuses on optimizing these factors to enhance integration. For instance, developing scaffolds that can degrade at matched rates to tissue growth may facilitate better integration. However, achieving the desired level of functionality and integration presents a complex array of scientific and clinical hurdles.
In summary, addressing these challenges in vascularization, immune response, and integration is key to the advancement of heart tissue engineering. Continuous research and innovation are vital to resolve these issues, thus paving the way for more effective regenerative therapies for cardiac conditions.
Regulatory and Ethical Considerations
Regulatory and ethical considerations play a vital role in the advancement of heart tissue engineering. These factors are essential for ensuring that innovative therapies not only meet scientific and clinical standards but also adhere to ethical norms that protect patients and research subjects. Understanding the regulatory landscape allows researchers and clinicians to navigate the complexities of bringing new treatments from bench to bedside.
The regulatory framework is designed to guarantee the safety and efficacy of new therapies. Additionally, ethical considerations underscore the importance of integrity in research practices and patient welfare. These aspects help mitigate risks associated with emerging technologies while fostering public trust in medical advancements.
Safety and Efficacy Requirements
The safety and efficacy requirements for heart tissue engineering products are stringent. Regulatory bodies, such as the U.S. Food and Drug Administration (FDA) and the European Medicines Agency (EMA), require robust evidence that demonstrates any new treatment’s performance before it can be approved for clinical use. This usually entails extensive preclinical studies followed by multiple phases of clinical trials.
An effective preclinical assessment typically involves the following elements:
- In vitro studies: Here, researchers use cellular models to evaluate how engineered tissues respond to varied conditions.
- In vivo studies: These studies involve animal models to assess how the therapy interacts with a living organism.
- Long-term follow-up: This is crucial to determine any delayed adverse effects that might arise after the treatment.
Essential factors that are evaluated during this process include:
- Biocompatibility: Materials used in constructs must not provoke an immune response.
- Mechanical properties: The engineered tissues should mimic the characteristics of natural heart tissue.
- Functional performance: This includes assessments of contractility and electrical conductivity traits.
Thorough evaluations help ensure that when new heart tissue engineering treatments make it to the clinic, they have undergone rigorous testing to minimize potential risks to patients.
Ethical Implications of Stem Cell Research
The use of stem cells in heart tissue engineering raises significant ethical implications. Stem cells, particularly those derived from embryos, are a subject of debate. Issues surrounding moral status, consent, and the potential for exploitation of human cells are critical areas of concern.
Some notable ethical considerations include:
- Informed consent: Researchers must guarantee that all participants understand the processes involved and potential risks. This applies particularly to adult stem cell donors and embryo sources.
- Moral status of embryos: The debate about whether embryos have rights complicates the discourse on embryonic stem cell research. Some individuals and groups oppose the destruction of embryos.
- Equity in access: As with many advanced medical technologies, there is a risk that only affluent individuals may benefit from cutting-edge stem cell therapies.
The future landscape of heart tissue engineering will depend not only on technological advancements but also on addressing these ethical concerns. Engaging stakeholders in dialogue and establishing comprehensive regulatory guidelines will be essential to promote responsible research practices and public confidence in future treatments.
"Integrating ethical considerations into biomedical research ensures respect for human dignity while advancing scientific knowledge."
As heart tissue engineering continues to evolve, it is crucial for stakeholders to remain vigilant about these regulatory and ethical dimensions. Only through thoughtful navigation and rigorous standards can the full potential of this field be realized while protecting patients and society.
Future Directions in Heart Tissue Engineering
The realm of heart tissue engineering is evolving rapidly, with profound implications for future health care strategies. As researchers and clinicians continue to confront limitations in current methodologies, the forecasted advancements hold the promise of revolutionizing treatment paradigms for cardiovascular diseases. This section examines emerging technologies and posits potential applications that can bridge the gap between current research and effective clinical practice.
Innovative Technologies on the Horizon
As technology progresses, several innovative approaches in heart tissue engineering are gaining traction. These include advancements in bioprinting, microfluidics, and gene editing.
- 3D Bioprinting: This method allows for the precise layering of cells and materials to create cardiac tissues that closely mimic the natural heart environment. Technologies like the bioink formulation are under development to improve cell viability and functionality after printing.
- Microfluidics: This technology can facilitate the study of cardiac cells in dynamic environments, enabling researchers to observe how tissues respond to mechanical stimuli and pharmaceuticals in real time.
- Gene Editing Tools: Techniques such as CRISPR-Cas9 are poised to enhance the ability to customize cardiac cells, potentially repairing genetic defects at their source. This capability could vastly improve patient outcomes by tailoring treatments to individual genetic profiles.
These technologies, combined with ongoing research in biomaterials, are likely to yield more effective and patient-responsive heart tissues in the near future.
Forecasting Clinical Applications
The anticipated benefits of innovations in heart tissue engineering extend into various clinical applications. Presently, challenges exist in translating research into real-world benefits, but the outlook is increasingly optimistic.
- Regeneration of Damaged Tissue: The ability to create functional, vascularized heart tissue could allow for new strategies in repairing areas damaged by myocardial infarction. This regenerative approach promises to restore heart function rather than merely managing symptoms.
- Personalized Medicine: With the integration of bioprinting and gene editing, it is possible to create treatments that are tailored to the genetic makeup of each patient. This could significantly reduce the prevalence of adverse reactions and improve recovery times.
- Combination Therapies: Future applications may involve synergistic approaches that combine tissue engineering strategies with pharmacological treatments. This dual approach could enhance the efficacy of existing drugs and improve patient care management.
The future of heart tissue engineering lies not only in technological advancements but also in the ability to seamlessly integrate these technologies into everyday medical practice.
Addressing the complexities of patient care is vital. As these innovative technologies make their way from laboratory studies to clinical trials, the need for collaborative efforts across disciplines will be paramount. This collaboration can facilitate a more comprehensive understanding of patient needs and ensure that the developments in heart tissue engineering translate into tangible health benefits.
The End
The conclusion of this article holds significant importance as it encapsulates the advancements and ongoing challenges in heart tissue engineering. This field, integral to regenerative medicine, promises revolutionary solutions for cardiovascular diseases. Understanding these developments allows us to appreciate the intricate balance between scientific innovation and practical application.
Summary of Key Points
- Heart Tissue Engineering Foundations: The integration of biological systems with engineering principles marks a turning point in modern medicine.
- Innovative Cell Sources: The availability of various cell types such as stem cells and cardiac progenitor cells is crucial for creating functional heart tissue.
- Material Selection: The choice of scaffold materials, whether natural or synthetic, impacts the success of tissue integration and functionality.
- 3D Bioprinting: This technology proves promising for creating complex cardiac structures that mimic natural tissue.
- Clinical Applications: While significant progress has been made, real-world applications for conditions like acute myocardial infarction and chronic heart failure remain a focal point.
- Regulatory and Ethical Issues: Navigating these elements is essential for future advancements and successful patient outcomes.
By summarizing these critical points, it is clear that heart tissue engineering is not just a scientific endeavor but also a platform derailing future healthcare solutions.
Call for Multidisciplinary Collaboration
To fully realize the potential of heart tissue engineering, a call for multidisciplinary collaboration is essential. These efforts can bridge gaps between various fields:
- Biomedical Engineering: Engineers contribute by designing effective scaffolds and biocompatible materials.
- Cell Biology: Understanding cellular mechanisms better informs tissue development strategies.
- Clinical Research: Clinicians provide insights from patient care that can guide research toward practical applications.
- Regulatory Experts: Their role is vital in navigating the legal landscape as innovations emerge.
Together, these diverse professionals can cultivate a collaborative environment enhancing research efforts and accelerating the translation of lab developments into clinical realities. Such partnerships can drive forward-thinking solutions, refining techniques and improving safety standards, ultimately leading to better tools for patient care.
The future thus hinges on the interaction of these fields, making collaborative efforts not just beneficial but necessary.