Exploring High Fidelity Polymerases in Molecular Biology
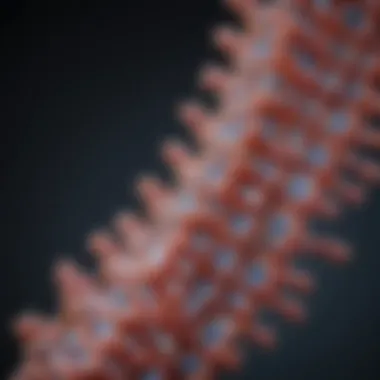
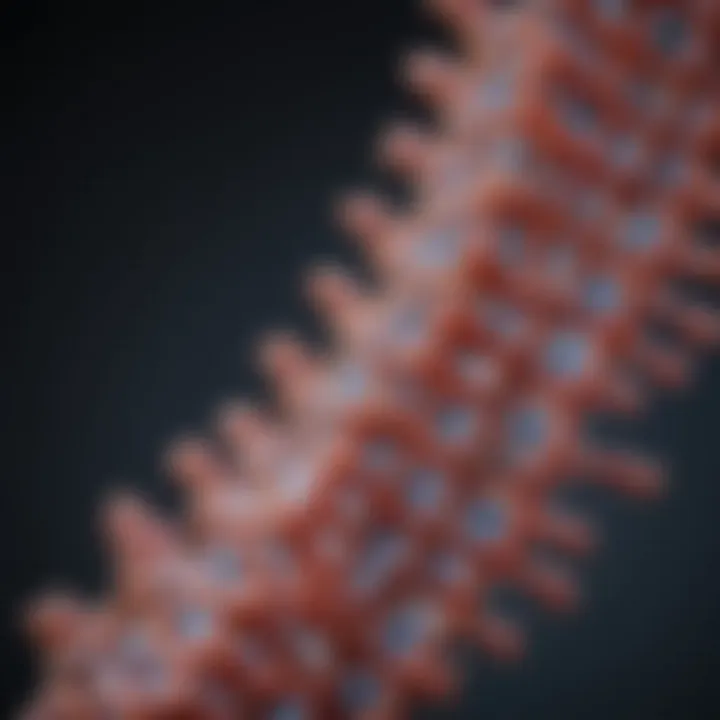
Intro
High fidelity polymerases have become crucial tools in molecular biology. Their ability to synthesize DNA accurately allows researchers to explore genetic material with enhanced reliability. This section introduces the fundamental aspects of high fidelity polymerases, including their mechanisms, significance, and applications in various fields.
High fidelity polymerases perform DNA synthesis with remarkably high accuracy, minimizing errors that can lead to mutations or other undesirable outcomes. This precision is critical in applications such as gene cloning, sequencing, and diagnostic testing. The enzyme's structure and catalytic properties contribute to its exceptional performance, making it a subject of interest both in academic research and in the biotechnology industry.
With the ongoing advancements in enzyme engineering, high fidelity polymerases have seen enhancements that increase their efficiency and specificity. Understanding these developments benefits not only theoretical knowledge but also practical applications. This exploration will provide insights into how these enzymes work and their implications in the evolving landscape of molecular biology.
Research Methodology
Description of Research Design and Approach
This article employs a qualitative research approach, synthesizing current literature on high fidelity polymerases. The aim is to extract and analyze key findings related to enzyme mechanisms, performance, and applications.
Primary sources include peer-reviewed journal articles focusing on polymerase characterization, performance data, and recent innovations in enzyme engineering. This comprehensive review facilitates a structured examination of the existing knowledge in the field.
Materials and Methods Used in the Study
Research on high fidelity polymerases involves various methods to assess their performance, such as:
- Enzyme assays: These assess the activity and accuracy of polymerases during DNA replication. Data is collected to illustrate their effectiveness under different conditions.
- Gel electrophoresis: This technique is used to analyze the products generated by polymerase reactions, providing insights into error rates and fidelity.
- Mutagenesis studies: These explore the impact of specific conditions and modifications on enzyme performance, revealing pathways for enhanced fidelity.
Discussion and Interpretation
Interpretation of Results in the Context of Existing Literature
Current literature highlights that high fidelity polymerases, like Taq polymerase, exhibit superior accuracy compared to traditional polymerases. Studies have shown that these enzymes can achieve error rates of less than one mistake per 10,000 base pairs. This performance is pivotal in applications requiring precise genetic replication.
The implications of this enhanced fidelity extend to fields such as diagnostics, where even minor errors can lead to significant consequences. High fidelity polymerase applications in next-generation sequencing also underscore this point, as sequencing accuracy is paramount for reliable genomic analysis.
Implications for Future Research or Practical Applications
The ongoing advancements in polymerase engineering suggest a promising future. As research progresses, we expect to see:
- Development of new polymerases tailored for specific applications, improving efficiency and specificity.
- Enhanced understanding of polymerase mechanisms, paving the way for innovative applications in synthetic biology and gene therapy.
- Greater accessibility to high fidelity enzymes, enabling widespread use in laboratories globally.
The future of high fidelity polymerases is integral to advancing molecular biology, impacting both research methodologies and practical applications.
Through this examination, the article aims to enrich the reader's understanding of high fidelity polymerases, their functions, and their significance in ongoing and future scientific endeavors.
Prelims to High Fidelity Polymerases
High fidelity polymerases are essential tools in molecular biology, offering significant advantages in accuracy and efficiency during DNA synthesis. This introductory section outlines the critical role these enzymes play in various applications, ranging from basic research to clinical diagnostics. Understanding the significance of high fidelity polymerases can greatly enhance one’s ability to conduct experimental work and achieve reliable results.
Research and biotechnology benefit immensely from these enzymes. They minimize errors in DNA replication, thus supporting studies in genetics, genomics, and synthetic biology. Additionally, their proficiency in producing high-quality DNA enables advancements in areas such as gene cloning, sequencing, and mutagenesis. This foundational knowledge highlights the necessity for scientists to grasp how high fidelity polymerases work and their functional characteristics, leading to improved methodologies and outcomes in their work.
Definition and Importance
High fidelity polymerases can be defined as DNA polymerases that possess enhanced accuracy in incorporating nucleotides during DNA replication. Unlike standard polymerases, which may introduce a range of errors, these specialized enzymes exhibit significantly lower error rates. Their importance cannot be overstated; in many applications, such as cloning and next-generation sequencing, the integrity of results hinges on the precision of polymerases used.
In a nutshell, the use of high fidelity polymerases ensures that the genetic material produced is highly reliable, paving the way for advancements in genetic engineering and synthetic biology. This reliability is particularly vital when analyzing the function of genes or when developing therapeutic interventions that require accurate DNA manipulation.
Historical Context
The evolution of polymerases reflects the advancements in biotechnology. Early DNA polymerases, derived from organisms such as E. coli, were commonly used for DNA manipulation. However, these enzymes were suboptimal for applications requiring high accuracy due to their relatively high error rates. As molecular techniques evolved, so did the need for improved enzymes.
In the late 20th century, researchers began to isolate and characterize high fidelity polymerases from other organisms, especially those thriving in extreme environments. Notable examples include Taq polymerase, derived from the thermophilic bacterium Thermus aquaticus, and Pfu polymerase, known for its high fidelity stemming from its archaeological origins.
The scientific community's focus on enhancing the fidelity of DNA synthesis led to the development and refinement of various high fidelity enzymes over the years. Innovations in enzyme engineering have enabled the design of polymerases with tailored properties and enhanced performance, reflecting the dynamic relationship between technological development and scientific exploration.
Understanding the historical progression of high fidelity polymerases is paramount. It informs current research practices and illustrates how the field has continuously adapted to meet growing demands for precision and accuracy in genetic work.
Mechanisms of Action
The mechanisms of action of high fidelity polymerases are crucial to understanding their role in molecular biology. These mechanisms reveal how these enzymes maintain their high accuracy during DNA synthesis. By exploring the structure and function of these enzymes, researchers can enhance their applications in various fields.
Structural Characteristics
The structural characteristics of high fidelity polymerases play a significant part in their functionality. These enzymes are typically complex proteins composed of several domains. Each of these domains contributes to their activity and specificity. For example, the palm, fingers, and thumb domains work collaboratively to facilitate the binding of DNA and nucleotides. The palm region is often where the catalytic activity occurs.
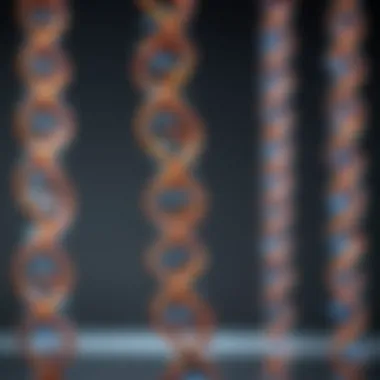
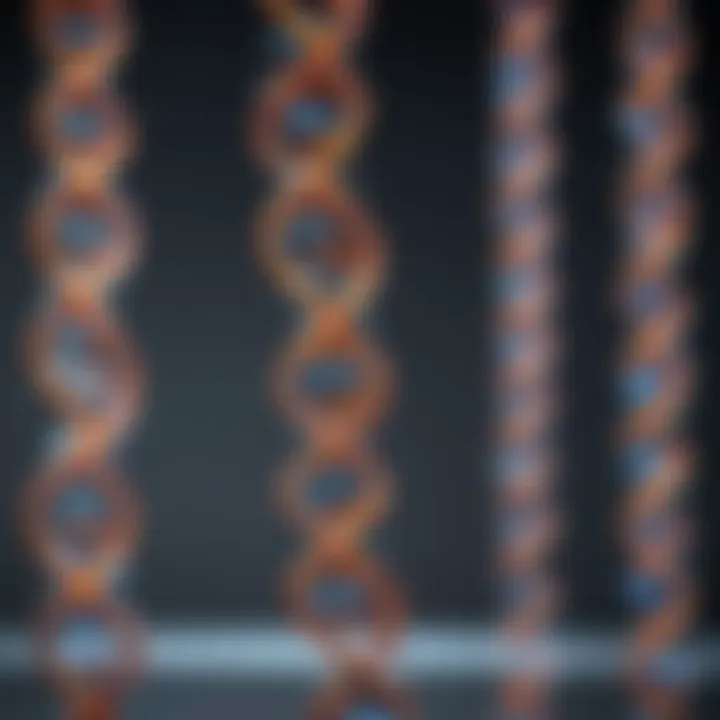
Key aspects include:
- Active site configuration: The shape and charge of the active site allow for precise nucleotide incorporation.
- Metal ion coordination: Metal ions like magnesium are essential for the polymerase activity, helping stabilize the negatively charged DNA backbone during synthesis.
- Structural flexibility: The ability of the enzyme to adapt its shape during the catalytic process can minimize errors in nucleotide pairing.
These characteristics collectively ensure that high fidelity polymerases can effectively read templates and synthesize complementary DNA strands with minimal error.
Enzymatic Pathways
Enzymatic pathways in high fidelity polymerases encompass the biochemical processes these enzymes employ to synthesize DNA. Understanding these pathways is essential for optimizing their use in laboratory settings.
The main enzymatic pathway involves several key steps:
- Binding: The polymerase binds to a single-stranded DNA template.
- Nucleotide selection: The enzyme selects and positions the correct nucleotide for incorporation.
- Catalysis: The polymerase catalyzes the formation of a phosphodiester bond between nucleotides.
- Proofreading: Many high fidelity polymerases have a proofreading activity that checks and removes incorrectly paired nucleotides. This step is pivotal in ensuring low error rates.
In summary, the mechanisms of action of high fidelity polymerases highlight their structural intricacies and enzymatic functions, both of which are fundamental to DNA synthesis accuracy. This understanding is vital for anyone working in molecular biology.
"The reliability of high fidelity polymerases in providing accurate DNA synthesis cannot be overstated, as their mechanisms are finely tuned to minimize errors."
For further exploration of the topic, consider visiting Wikipedia.
Accuracy in DNA Synthesis
The importance of accuracy in DNA synthesis cannot be overstated, particularly in the context of high fidelity polymerases. These enzymes are pivotal in ensuring the integrity of genetic information during replication and amplification processes. The precision with which planty enzymes replicate DNA markedly influences the results of experiments in molecular biology, including cloning, sequencing, and mutagenesis. High fidelity polymerases play a critical role in minimizing errors, which can lead to incorrect sequences and potentially flawed biological conclusions.
Error Rates Compared to Other Polymerases
High fidelity polymerases exhibit significantly lower error rates compared to traditional DNA polymerases. For instance, while Taq polymerase has an error rate that can be as high as 1 in 1,000 bases, high fidelity enzymes such as Phusion or Q5 polymerase can achieve error rates as low as 1 in 100,000 to 1 in 1,000,000 bases. This drastic difference in accuracy can make a substantial impact on downstream applications.
- Taq Polymerase:
- Phusion Polymerase:
- Q5 Polymerase:
- Error Rate: ~1 in 1,000
- Characteristics: Fast but low fidelity
- Error Rate: ~1 in 100,000
- Characteristics: High speed, high fidelity
- Error Rate: ~1 in 1,000,000
- Characteristics: Extremely high accuracy, versatile
This disparity means that researchers can rely on high fidelity polymerases for experiments demanding stringent accuracy, which is crucial when working with sensitive genetic materials.
Factors Affecting Fidelity
Several factors can influence the fidelity of DNA synthesis by polymerases. Key factors include:
- Temperature: Many high fidelity polymerases function optimally at elevated temperatures, enhancing their accuracy in DNA synthesis. However, excessive heat can lead to denaturation of the enzyme, resulting in decreased fidelity.
- pH Levels: The pH conditions during PCR reactions can also affect the polymerase activity. Maintaining a stable pH is essential for optimal enzyme performance
- Ionic Strength of Reactions: The concentration of salts, particularly magnesium ions, can influence the fidelity of the polymerase. High magnesium concentrations can enhance activity but may also increase error rates in some cases.
"Maintaining precise conditions can be the difference between a well-executed experiment and one riddled with errors."
Adjusting these variables can lead to improved outcomes in experiments. Thus, understanding these factors is essential for researchers to maximize the effectiveness of high fidelity polymerases in their work.
Applications in Molecular Biology
High fidelity polymerases are essential tools in molecular biology, facilitating numerous applications with a high degree of accuracy. Their ability to synthesize DNA with minimal errors makes them invaluable in research, diagnostics, and biotechnology. Understanding these applications allows researchers and practitioners to leverage the strengths of high fidelity polymerases effectively.
PCR Techniques
Polymerase Chain Reaction, or PCR, is a fundamental technique in molecular biology. High fidelity polymerases, such as Phusion DNA Polymerase or Q5 High-Fidelity DNA Polymerase, play a crucial role by ensuring that the generated DNA amplifications maintain fidelity across multiple cycles of denaturation, annealing, and extension. The precision of high fidelity polymerases minimizes introduction of mutations, which is vital for downstream applications like cloning and sequencing.
In PCR, enzyme selection directly affects the experimental outcome. High fidelity polymerases often have specialized properties, such as enhanced processivity and resistance to inhibitors.
Advantages of High Fidelity DNA Polymerases in PCR include:
- Scalability: Suitable for applications from small to large-scale DNA amplification.
- Specificity: Reduced primer-dimer formation due to streamlined reaction conditions.
- Yield: Higher and more consistent yields compared to traditional Taq polymerases.
"The choice of polymerase in PCR defines the accuracy and utility of the results obtained, making high fidelity enzymes an essential consideration."
Cloning and Sequencing
Cloning and sequencing of DNA are key processes in molecular biology, and they benefit significantly from the use of high fidelity polymerases. When constructing plasmids or other vectors, accurate DNA replication is paramount. Errors during the amplification process can lead to incorrect sequences, rendering clones unusable. High fidelity polymerases provide the reliability needed in constructing these vectors, ensuring that the inserted sequences are correct and can function as intended in application contexts.
In sequencing, precision is of highest priority. Using high fidelity polymerases reduces the background noise during sequencing runs, leading to clearer results. This is particularly important for next-generation sequencing technologies, which can easily detect inaccuracies.
Mutagenesis Studies
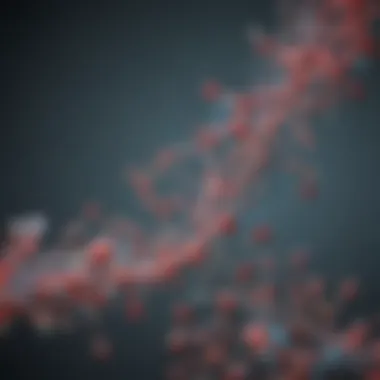
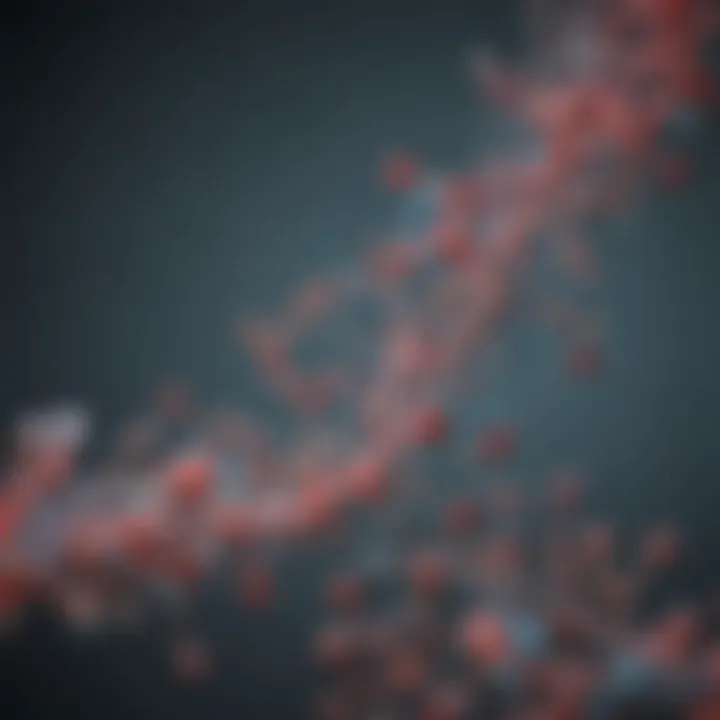
Mutagenesis studies aim to induce changes in specific DNA sequences and assess the resulting phenotypic effects in organisms. High fidelity polymerases facilitate site-directed mutagenesis approaches, such as the QuikChange method. These polymerases allow precise incorporation of mutations without introducing additional, unintended errors.
Key benefits of using high fidelity polymerases in mutagenesis include:
- Precision: Allows for targeted mutations while maintaining the integrity of surrounding sequences.
- Efficiency: Optimized reaction conditions lead to successful incorporation of mutations in fewer attempts.
- Versatility: Applicable in both plasmid-based and lentiviral systems for diverse research needs.
Overall, applications involving high fidelity polymerases significantly advance the field of molecular biology. These tools not only enhance experimental accuracy but also broaden the scope of research possibilities.
Polymerase Characterization
Characterizing polymerases is essential to fully understand their role and efficacy in molecular biology. Characterization allows for evaluation of the enzyme's properties, which directly impact its performance in various applications. This section focuses on two critical aspects: enzyme kinetics and stability under varying conditions such as temperature and pH.
Enzyme Kinetics
Enzyme kinetics refers to the study of the rates of enzyme-catalyzed reactions. For high fidelity polymerases, understanding kinetics is vital. It determines how quickly the enzyme synthesizes DNA and how effectively it can incorporate nucleotides without errors. The rate of reaction can be influenced by several factors:
- Substrate Concentration: At low substrate levels, a higher rate of increase in the product synthesis is observed. However, saturation eventually occurs, where increasing substrate concentration does not significantly enhance the reaction rate.
- Enzyme Concentration: A higher concentration of the polymerase generally leads to an increased reaction rate, provided substrates are available in excess.
- Temperature: The activity of the enzyme often increases with temperature, up to an optimal point, after which activity can decrease sharply.
These kinetic parameters can be quantified using the Michaelis-Menten equation, allowing researchers to determine maximum reaction rates and affinity constants. The precision of a polymerase's kinetic profile help in selecting the appropriate enzyme for specific applications in research and industry.
Temperature and pH Stability
Stability is another fundamental characteristic of polymerases. Each enzyme operates optimally within a specific range of temperature and pH, which can significantly influence its performance.
- Temperature Stability: Polymerases must maintain stability over a range of temperatures to perform effectively in techniques such as PCR. Many high fidelity polymerases can withstand higher temperatures, which are critical for denaturing DNA. Optimal temperatures enhance polymerase activity while minimizing degradation and denaturation.
- pH Stability: Polymerases also exhibit specific pH ranges where they function best. Deviations from this range can lead to reduced activity or complete loss of function. Understanding the optimal pH conditions is crucial when designing experiments, especially in buffer formulation.
Maintaining optimal temperature and pH conditions allows for greater efficiency and accuracy in DNA synthesis, reducing error rates significantly.
Optimization for Laboratory Use
In the realm of molecular biology, the optimization of high fidelity polymerases for laboratory use plays a crucial role in experimental success. This optimization ensures that the enzyme performs at its best, leading to reliable and reproducible results. Focusing on specific elements, such as buffer conditions and reagent concentrations, can drastically enhance the performance of polymerases in practical applications. By fine-tuning these factors, researchers can improve the fidelity and efficiency of DNA amplification, thereby expanding the range of possible experiments.
Buffer Conditions
Buffer conditions significantly affect polymerase activity. The pH, ionic strength, and composition of the buffer can impact enzyme functionality. High fidelity polymerases generally operate optimally at specific pH ranges, often between 7.0 and 9.0. Deviations from this range may result in decreased activity or even denaturation of the enzyme. Furthermore, the presence of optimal concentrations of specific ions, such as magnesium, is critical. Magnesium ion stabilizes the polymerase-DNA complex and is essential for catalysis. Researchers must conduct preliminary trials to determine the ideal buffer formulation for their specific assays.
When adjusting buffer conditions, consider the following points:
- pH stability: Ensure that the chosen pH is consistent with the polymerase’s optimal activity range.
- Ionic strength: Higher salt concentrations can enhance polymerase stability but may inhibit activity in some cases.
Additionally, here is a quick reference for buffer components:
- Tris-HCl: Commonly used due to its pH stability.
- Magnesium chloride: Essential for DNA synthesis.
- Sodium chloride: Helps maintain ionic strength.
Reagent Concentrations
Another important aspect of laboratory optimization is the concentration of reagents used in conjunction with high fidelity polymerases. The concentrations of nucleotides, polymerases, and primers must be balanced for optimal reaction conditions. If the concentration of any component is too low, it may lead to incomplete amplification, while excessive amounts might result in non-specific products.
To optimize reagent concentrations:
- Polymerase concentration: Use the enzyme at its recommended level. Too little may yield poor results, while too much can lead to increased background noise in assays.
- Nucleotide ratio: Ensure an equal ratio of dNTPs, as they are all required for the polymerization process.
- Primers concentration: Optimal primer concentration aids in specific binding and reduces non-specific amplification.
Rather than a one-size-fits-all approach, each experiment may require specific adjustments. Measuring these concentrations precisely is paramount, as they can influence the overall yield and accuracy of PCR products.
Optimization extends beyond just choosing high fidelity polymerases; it encompasses a holistic approach to experimental design, integrating buffer chemistry and reagent preparations for superior results.
In summary, careful consideration and adjustment of buffer conditions and reagent concentrations stand pivotal in the successful application of high fidelity polymerases in laboratory settings. This process necessitates experimentation and may differ across various protocols, thus highlighting the intricate nature of polymerase optimization.
Polymerase Engineering and Innovations
Polymerase engineering reflects a dynamic intersection of science and technology. In molecular biology, high fidelity polymerases have gained a prominent role. Their precision in DNA synthesis translates to broad applications in research and biotechnology. Essentially, engineering these enzymes can enhance their performance and expand their utility in various disciplines.
The beauty of polymerase engineering lies in its ability to tailor enzymes for specific functions. For example, modulations in enzyme structure can result in improved stability and activity under varied conditions. These enhancements often yield enzymes that work efficiently across a range of temperatures and pH levels. When you can alter the functionalities of these polymerases, it also affects their efficiency in PCR amplification and other molecular techniques.
In light of this, the benefits of polymerase innovations include but aren't limited to, increased yield, heightened specificity, and reduced error rates. However, careful consideration is necessary when undertaking polymerase engineering. The engineering process may pose challenges, such as unpredictable interactions resulting from alterations in the enzyme's structure.
Directed Evolution
Directed evolution serves as a powerful approach in polymerase engineering. This method allows for the iterative selection and amplification of enzyme variants with desired traits. The process begins with the introduction of genetic diversity into the polymerase gene. This can be achieved through a variety of techniques, such as random mutagenesis.
Subsequently, these variants are screened to identify those that exhibit superior characteristics. High-throughput screening methods often facilitate this selection process. The selected variants can then undergo additional rounds of mutation and selection, continuously refining the polymerase's properties. This method has successfully produced novel polymerases with enhanced fidelity and efficiency, marking significant advancements in the field.
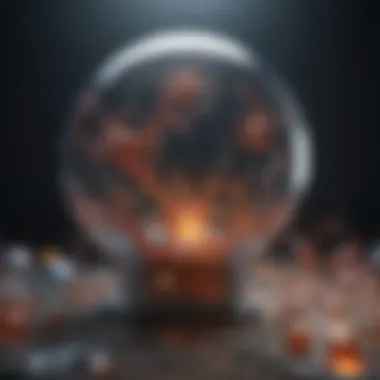
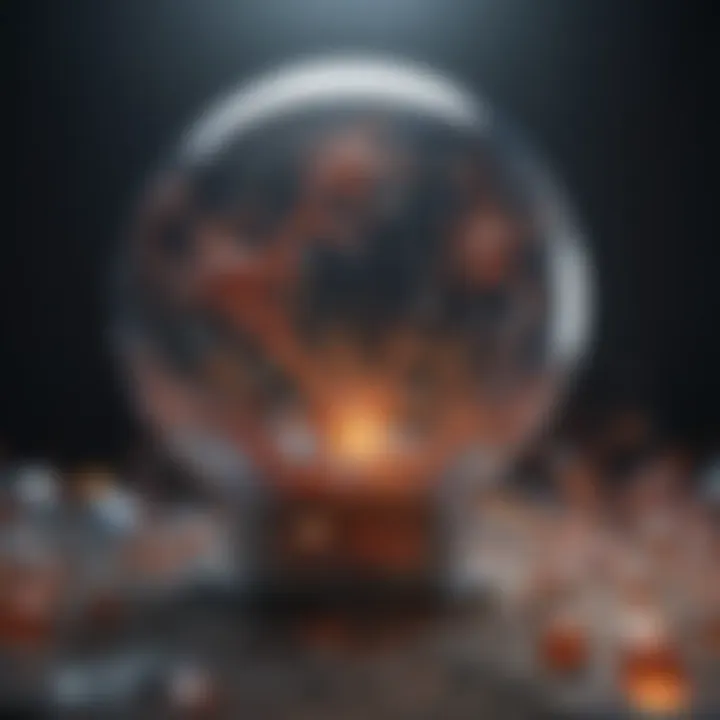
In practical terms, directed evolution can yield polymerases tailored for specific applications, such as those used in extreme conditions where traditional enzymes might fail.
Synthetic Biology Applications
The interplay between high fidelity polymerases and synthetic biology highlights significant progress in biotechnology. Polymerases engineered for synthetic biology applications contribute to the creation and manipulation of genetic material. They support the construction of complex genetic circuits, capable of executing specific functions within organisms.
These engineered polymerases enhance pathways in the assembly of DNA parts or modules, allowing for more precise construction of synthetic constructs. Such applications are pivotal in fields ranging from medicine to environmental science.
Consider synthetic requirements in gene therapy, where precise delivery and expression of therapeutic genes are necessary. Engineered high fidelity polymerases can facilitate accurate gene assembly, vital for successful therapeutic outcomes. Additionally, in the field of bioremediation, polymerases can aid the design of organisms capable of breaking down pollutants.
By harnessing the capabilities of engineered polymerases, researchers can explore new frontiers in genetic manipulation, pushing the boundaries of what is possible in molecular biology.
By continuing to investigate polymerase engineering and its various innovations, the scientific community stands to enhance numerous applications that may one day solve pressing problems in health, technology, and the environment.
Challenges and Limitations
High fidelity polymerases play a significant role in the field of molecular biology. However, with their advantages come certain challenges and limitations that researchers must consider. Understanding these issues is essential for optimizing their use in various applications. In this section, we will explore specific elements that encompass challenges related to cost, accessibility, and specificity. By dissecting these topics, we can appreciate both the benefits and limitations of utilizing high fidelity polymerases.
Cost and Accessibility
The cost associated with high fidelity polymerases can be a considerable barrier for many laboratories, especially those with limited funding. High fidelity polymerases are often more expensive than their standard counterparts. This premium pricing often stems from the complex processes involved in developing them, including protein engineering and purification techniques.
Access to these specialized enzymes can vary, depending on geographical location and available suppliers. Some researchers might find it difficult to acquire high fidelity polymerases due to limited sources. Additionally, budget constraints can restrict the ability to purchase enough enzyme for multiple experiments, leading to potential subpar results. Researchers may need to carefully assess not only the cost in terms of money but also the implications of possible compromises in research quality.
Here are some considerations regarding cost and accessibility:
- Supplier Variability: The presence of multiple suppliers can lead to fluctuations in pricing.
- Bulk Purchase Options: Buying in bulk may reduce costs, but the initial investment can still be high for many labs.
- Regional Disparities: Some regions have limited access to high-quality reagents, which can restrict research opportunities in those areas.
"The accessibility of high fidelity polymerases must be factored into research design, as it can significantly impact experimental outcomes."
Specificity Issues
Specificity is a critical aspect of high fidelity polymerases, as these enzymes are designed to amplify DNA with minimal errors. However, achieving high specificity is an ongoing challenge.
One issue arises from the potential for the enzyme to amplify non-target sequences. This can occur due to cross-reactivity, which reduces the overall fidelity of the process. In some cases, high fidelity polymerases can still bind to similar sequences, leading to non-specific amplification. The implications are far-reaching, affecting downstream applications like cloning, where precision is vital. To mitigate these specificity issues, researchers often have to optimize several parameters including:
- Annealing Temperature: Adjusting the temperature can help enhance specificity.
- Primer Design: Careful design of primers can avoid complementary regions in non-target sequences.
- Enzyme Selection: Different polymerases may vary in their specificity profiles, influencing the choice based on the application.
Research continues to address specificity issues by developing even more refined enzymes that can discriminate better between target and non-target sequences.
Future Directions in Research
The future of high fidelity polymerases is both promising and critical for advancements in molecular biology. Ongoing research increasingly focuses on diversifying their applications and improving their efficiency. As understanding of these enzymes deepens, potential novel uses are emerging in fields such as personalized medicine, environmental science, and synthetic biology. The exploration of high fidelity polymerases is not purely academic; it also holds practical implications that can significantly influence scientific progress.
Potential New Applications
Various new areas are ripe for exploration where high fidelity polymerases can be applied.
- Next-Generation Sequencing: The demand for faster and more accurate sequencing is rising. High fidelity polymerases can provide accurate base calling, crucial for reliable genomic analysis.
- Gene Therapy: With their precision, these polymerases can potentially drive the development of more effective gene therapy solutions, correcting genetic defects with higher accuracy.
- Metagenomics: In studying complex microbial communities, the accurate amplification of DNA allows for more reliable insights into biodiversity and ecosystem health. The use of high fidelity polymerases can help elucidate genetic diversity in environmental samples.
- Agricultural Biotechnology: The precision offered by these enzymes can lead to innovative crop modifications, enhancing yield and resilience against diseases.
These potential applications suggest that high fidelity polymerases could revolutionize many sectors beyond traditional research labs.
Advancements in Technique Development
Technique advancements also play a crucial role in furthering the capabilities of high fidelity polymerases. Some significant efforts include:
- Enhanced Processivity: Developing polymerases with improved processivity allows for longer DNA fragments to be replicated accurately in a single reaction, saving time and resources in molecular experiments.
- Increased Thermal Stability: By engineering polymerases that withstand higher temperatures, their usability in thermocycling procedures can be enhanced, thus improving reaction efficiency.
- Improved Error Correction: Continued research aims to augment the natural error-correcting abilities of these enzymes. This can minimize the occurrence of mismatched bases during DNA synthesis, particularly in critical applications like gene cloning and sequencing.
- Combining Various Features: Integrating multiple beneficial traits into a single polymerase has the potential to streamline reactions further and allow for more complex and nuanced biochemical experiments.
These advancements indicate that the field is moving towards more robust and versatile polymerases, helping researchers push the boundaries of what's currently achievable in molecular biology.
Ongoing innovation in polymerase technologies paves the way for future breakthroughs in research applications.
Culmination
The conclusion serves as a significant wrap-up for this article, summarizing the intricate aspects of high fidelity polymerases discussed throughout the sections. This final part reiterates the essential takeaways for readers, ensuring a comprehensive understanding of the subject matter. It highlights the pivotal role that high fidelity polymerases play in molecular biology and their applications in various fields such as research, biotechnology, and clinical diagnostics.
Summary of Key Points
Several key points emerge from this exploration of high fidelity polymerases:
- Definition and Importance: High fidelity polymerases are critical for accurate DNA synthesis, making them indispensable tools in many molecular biology applications.
- Mechanisms of Action: Understanding their structural characteristics and enzymatic pathways provides insights into their high precision during DNA replication.
- Applications: These polymerases are widely used in PCR, cloning, sequencing, and mutagenesis studies, demonstrating their versatility.
- Polymerase Characterization: The analysis of enzyme kinetics, temperature, and pH stability is vital for optimizing their performance in laboratory settings.
- Engineering Innovations: Advances in polymerase engineering, including directed evolution and synthetic biology applications, showcase the ongoing developments in this field.
- Challenges: Issues of cost, accessibility, and specificity remain challenges that researchers must address to fully utilize high fidelity polymerases.
Final Thoughts on High Fidelity Polymerases
In summary, high fidelity polymerases represent a cornerstone in molecular biology. Their accuracy and reliability fundamentally shape the outcomes of various applications, from fundamental research to clinical settings. As researchers continue to push the boundaries of technology and applications, high fidelity polymerases will likely evolve further, offering new possibilities for genetic analysis and manipulation. The exploration of new polymerase variants and innovative engineering techniques promises to enhance their utility, potentially resolving current limitations regarding cost and specificity.
This article has aimed to provide a detailed overview and an informed perspective on high fidelity polymerases. The knowledge shared here should serve as a foundation for students, researchers, and professionals interested in harnessing these powerful tools in their work.