High Molecular Weight DNA: Properties and Applications
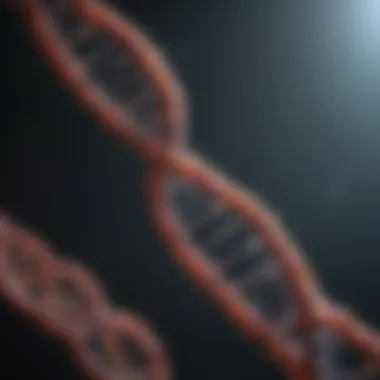
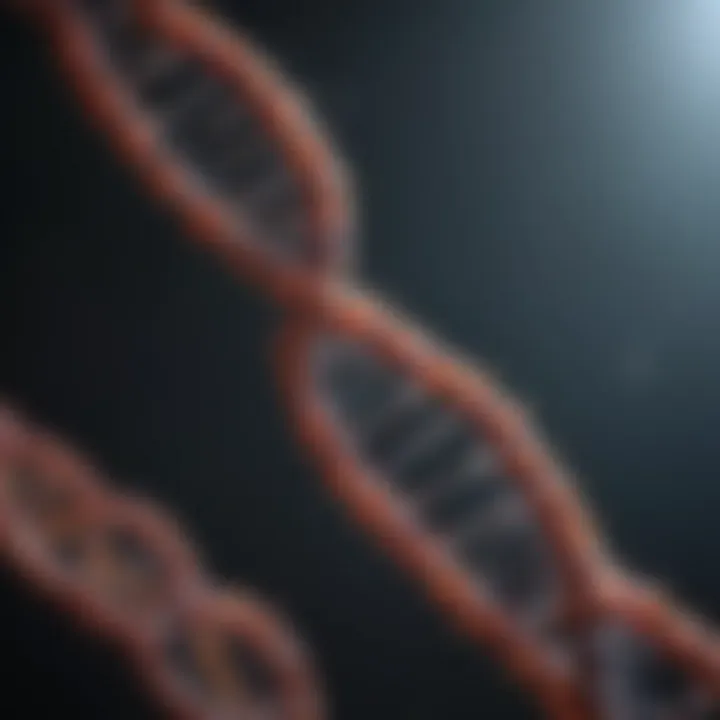
Intro
High molecular weight DNA is not just a fancy term thrown around in research labs; it's a cornerstone in a plethora of scientific endeavors. From genetic sequencing to crafting biotechnological innovations, understanding high molecular weight DNA can be the key that unlocks many doors in the field of molecular biology.
One might wonder, why the emphasis on high molecular weight? Simply put, it often has superior integrity and stability, making it especially valuable for applications that demand nuanced detail, such as studying large genomic regions or complex genetic pathways. Yet, navigating the intricacies of high molecular weight DNA comes with its challenges.
In this piece, we'll take a magnifying glass to various aspects surrounding high molecular weight DNA. We'll kick things off by dissecting the research methodologies commonly employed to study this vital biomolecule, followed by a dive into the implications of its applications in modern genetics and disease research. Consider this your playbook for understanding the role of high molecular weight DNA in pushing the frontiers of science forward.
Research Methodology
Description of research design and approach
The methodology surrounding high molecular weight DNA is multifaceted, drawn from diverse fields like genetics and molecular biology. Researchers typically use methods such as agarose gel electrophoresis and ultrasonication, which help in isolating and analyzing the unique characteristics of high molecular weight DNA. The goal is to harness its integrity for applications in predictive diagnostics or gene therapy.
To illustrate, the design often involves controlled conditions to minimize degradation during extraction. Teams employ rigorous protocols that can include:
- Mechanical shearing techniques to reduce fragmentation.
- Utilization of specialized buffers that maintain optimal pH.
- Focused centrifugation steps that separate high molecular weight DNA from contaminants.
Materials and methods used in the study
In order to study high molecular weight DNA, a range of materials and methods is put to use. Commonly, specialized extraction kits are employed. These kits are engineered to recover long DNA strands, promoting the preservation of their structural integrity. For example,
- Qiagen DNeasy Blood & Tissue Kit: This is widely used for isolating high molecular weight DNA from tissues and blood.
- Promega Wizard Genomic DNA Purification Kit: Another reliable option known for recovering longer strands effectively.
The methods chosen can significantly affect the research outcomes. Alongside these kits, researchers often utilize sonicators or symphonic extractors to manage the mechanical forces applied to the DNA sample. This precision helps prevent shearing that can occur if methods are mishandled.
"High molecular weight DNA is not merely an endpoint in research. It serves as a bridge leading to groundbreaking discoveries and innovative applications."
Discussion and Interpretation
Interpretation of results in the context of existing literature
When examining the landscape of high molecular weight DNA studies, it’s essential to relate findings back to existing literature. For example, some recent studies have revealed how high molecular weight DNA improves the performance of whole-genome sequencing, providing clearer insights into genetic mutations tied to diseases like cancer. Comparing these results to older studies shows a pronounced evolution in how high molecular weight DNA is perceived and applied.
Implications for future research or practical applications
Both the insights gained and the challenges identified in the study of high molecular weight DNA have broad implications. For the present and the future, technological advancements in DNA extraction and analysis can lead to innovations in personalized medicine, where treatments can be specifically tailored based on a patient’s genetic makeup. Furthermore, as researchers continue to unravel the complexities of high molecular weight DNA, new therapies for genetic disorders could arise.
The understanding of high molecular weight DNA doesn’t stand alone; it is interwoven with the entirety of genomic study and application. High molecular weight DNA can pave the way for breakthroughs in gene editing technologies like CRISPR, enhancing the precision and efficacy with which scientists can address genetic anomalies.
Preface to DNA
DNA, or deoxyribonucleic acid, is the molecule that contains the genetic instructions essential for the growth, development, functioning, and reproduction of all known living organisms and many viruses. Understanding DNA is crucial in a vast array of scientific disciplines, from genetics and molecular biology to biotechnology. This article will delve into high molecular weight DNA, focusing on its properties, extraction methods, and implications in various fields of research.
Fundamental Structure of DNA
At its core, the structure of DNA is often described as a double helix, resembling a twisted ladder. This formation is made up of two long strands of nucleotides, held together by hydrogen bonds between complementary base pairs. The four bases – adenine, thymine, cytosine, and guanine – pair specifically (A with T and C with G). Each nucleotide consists of a phosphate group, a sugar molecule, and a nitrogenous base. The sequence of these bases encodes genetic information, determining the unique traits of an organism. The arrangement, or sequence, of these bases can be likened to letters in a word, forming a comprehensive instruction manual for life, guiding everything from cellular processes to hereditary traits.
Role of DNA in Genetics
DNA plays a foundational role in genetics, serving as the blueprint for hereditary information. Each organism's genome – the complete set of DNA – facilitates the inheritance of traits from one generation to the next. Through the processes of replication and transcription, DNA ensures that genetic information is accurately copied and expressed, thereby fueling cellular functions and reproductive processes.
In essence, DNA defines an organism's characteristics and determines how it interacts with its environment. Mutations in DNA can lead to variations, some of which may confer advantages or result in diseases. For instance, understanding how alterations in DNA contribute to genetic disorders or cancer can be critical for developing therapeutic strategies.
"DNA is the blueprint for life; it encapsulates the essence of who we are as living beings."
In a nutshell, the significance of DNA stretches far beyond the basic understanding of its structure; it encompasses the entire spectrum of biological heritage. Grasping these fundamental aspects will set the stage for the nuanced discussion surrounding high molecular weight DNA and its implications across multiple domains.
Understanding Molecular Weight in DNA
Understanding molecular weight in DNA is more than just a scientific detail; it plays a pivotal role in the functioning of nucleic acids and their applications in various fields, especially in genetics and molecular biology. The molecular weight of DNA can influence how it behaves in biological systems, how it interacts with other molecules, and how it is manipulated in laboratory settings. It also has practical implications when it comes to techniques used for sequencing, cloning, and other genomic analyses.
Definition of Molecular Weight
Molecular weight, often referred to as molecular mass, is essentially the sum of the atomic weights of all the atoms in a molecule. In the context of DNA, this refers to the weight of nucleotides that make up the DNA strand. One must consider that DNA is composed of long chains of these nucleotides, with each nucleotide housing a sugar, a phosphate group, and a nitrogenous base. The molecular weight of DNA affects its stoichiometry, viscosity, and how well it can traverse through various media, such as gels during electrophoresis.
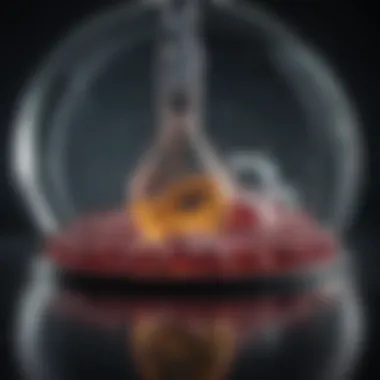
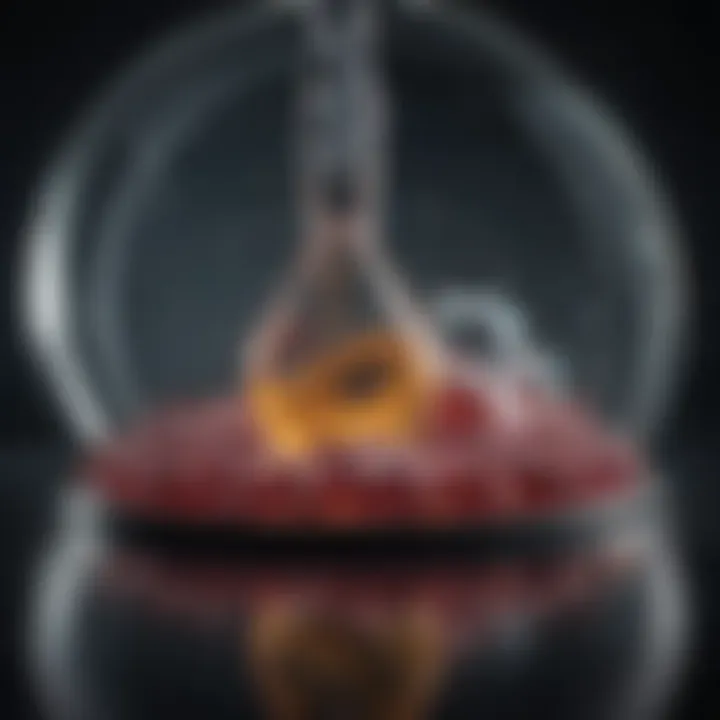
A simple way to quantify this is by using the formula:
Molecular Weight = (number of adenine × 331) + (number of thymine × 322) + (number of cytosine × 307) + (number of guanine × 347)
Here, the numbers represent the average molecular weights of the respective nucleotides in Dalton (Da).
Significance of High Molecular Weight DNA
The significance of high molecular weight DNA cannot be understated. In many aspects, it serves as the very backbone of genetic research and biotechnological innovations. High molecular weight DNA, generally defined as DNA with molecular weights exceeding 50 kilobases (kb), has some unique properties that set it apart from its lower molecular weight counterparts.
- Enhanced Stability: Compared to smaller DNA fragments, high molecular weight DNA is less prone to degradation due to its structural integrity. This allows for longer stable sequences, which is advantageous for applications such as genomic sequencing, where maintaining the original order of nucleotides is crucial.
- Holistic Genomic Insights: High molecular weight DNA allows researchers to look at broader genomic contexts, contributing to our understanding of complex traits and genetic disorders. By analyzing longer stretches of DNA, it is possible to identify regulatory elements and genomic architecture that would be missed in fragments.
- Applications in Cloning and Assembly: In cloning experiments, using high molecular weight DNA can result in more successful and precise constructions of recombinant DNA. Often, it is the case that larger constructs can carry more information, thus offering better options for gene therapy applications.
- Impact on Experimental Setup: When comparing high and low molecular weight DNA, researchers have to consider how molecular weight affects experimental outcomes. High molecular weight DNA typically results in fewer artifacts and noise in data analyses when conducting genomic studies.
High molecular weight DNA is not just data points; it tells the story of life’s complexity, aiding in deciphering genetic blueprints and unraveling the mysteries of organismal biology.
Properties of High Molecular Weight DNA
High molecular weight DNA possesses unique and essential properties that set it apart from its lower molecular weight counterparts. Understanding these properties is vital for researchers and practitioners in the field, as they directly influence how DNA is manipulated, studied, and applied in various scientific disciplines. High molecular weight DNA is not merely longer strands; its complexity adds layers of significance in areas such as genomics, genetics, and biotechnology.
Physical Characteristics
The physical characteristics of high molecular weight DNA dramatically impact its functionality. One of the most notable features is its length. High molecular weight DNA can consist of thousands to millions of base pairs, forming lengthy double helices that are crucial in encoding large amounts of genetic information. This extensive length enables the DNA to play significant roles in processes such as recombination and replication, which are foundational in maintaining genetic diversity.
Moreover, the conformation of these DNA strands tends to be more linear when compared to lower molecular weight variants. Such organization plays a pivotal role in gene expression. High molecular weight DNA is also more susceptible to mechanical shearing, a factor that needs to be carefully considered during extraction and handling. Researchers seeking high-quality samples need to ensure that the physical integrity of the DNA remains intact to avoid complications in downstream analyses.
"The manipulation of DNA requires understanding its physical attributes to maintain its integrity for successful applications in research and industry."
Several characteristics define the handling and analysis of high molecular weight DNA:
- Elasticity: High molecular weight DNA exhibits significant elasticity, which aids in processes like electrophoresis.
- Hydrophilicity: The hydrophilic nature of DNA influences its interactions with solvents, making it important for solubility and extraction methods.
- Viscosity: Longer DNA molecules tend to increase the viscosity of solutions, complicating certain laboratory procedures unless mitigated by specific techniques.
Chemical Stability
Chemical stability of high molecular weight DNA is another cornerstone in understanding its practical applications. The stability of these long strands is influenced by several forces, including hydrogen bonding, base stacking interactions, and ionic strength. Together, these forces contribute to the overall resilience of DNA in various environmental conditions.
One significant aspect of chemical stability is the susceptibility of high molecular weight DNA to degradation. Factors such as temperature, pH levels, and the presence of nucleases can lead to rapid breakdown of DNA, particularly during extraction and storage. It's not uncommon for researchers to face challenges related to the preservation of these fragile molecules. Therefore, employing protective environments, such as appropriate buffers and freezing techniques, becomes paramount for long-term research viability.
In contrast, high molecular weight DNA offers certain advantages when it comes to stability. For instance, the large size often allows for better reconstruction capabilities during processes like polymerase chain reaction (PCR), making it a valuable tool in various applications ranging from forensic science to genomics.
Factors to consider regarding the chemical stability of high molecular weight DNA include:
- Buffer Composition: Proper buffer systems can enhance stability and prevent degradation during isolation processes.
- Temperature Control: Low temperatures are generally favorable for the maintenance of DNA integrity.
- Avoidance of Chemical Contaminants: The presence of contaminants can compromise DNA quality and yield during extraction procedures.
Ultimately, recognizing the nuances of physical characteristics and chemical stability can empower scientists to advance their methodologies while minimizing complications in handling high molecular weight DNA. Such understanding embodies the foundational principles that underline the current and future landscape of genetic research and biotechnological innovation.
Methods of Extraction
The methods of extraction play a pivotal role in working with high molecular weight DNA. Understanding the extraction process is essential not only for obtaining quality DNA samples but also for ensuring that the integrity and functionality of the DNA is maintained throughout. This section explores both conventional techniques and more modern approaches to extraction, analyzing their respective merits and potential pitfalls.
Conventional Extraction Techniques
Conventional extraction methods have been the backbone of DNA extraction for decades. Techniques like phenol-chloroform extraction and alcohol precipitation have been widely used to separate DNA from proteins and other cellular debris.
- Phenol-Chloroform Extraction: In this method, phenol and chloroform are used to denature proteins, making it easier to isolate DNA into the aqueous phase. While effective, one must handle these reagents with utmost caution due to their hazardous nature.
- Alcohol Precipitation: This is a straightforward approach where an alcohol, typically ethanol or isopropanol, is added to a DNA solution, causing DNA to precipitate out. However, this method can yield varying results depending on the DNA concentration and purity of the initial sample.
Despite their effectiveness, conventional techniques have some drawbacks, including:
- Relatively lengthy processing times
- Potential for DNA degradation
- Use of hazardous chemicals that require careful disposal
These concerns often lead researchers to seek alternatives that can provide cleaner results or minimize handling risks.
Modern Approaches to Isolation
The advent of new technologies has revolutionized the extraction of high molecular weight DNA. Modern methods seek to simplify the extraction process, enhance DNA yield, and maintain integrity.
- Column-based Extraction: Utilizing silica membrane technology, this method streamlines the extraction process. DNA binds to the column while contaminants are washed away. Many find this method efficient, reducing handling time significantly.
- Magnetic Particle-Based Methods: These employ magnetic beads coated with DNA-binding agents. After mixing the sample, the beads can be easily separated using a magnet, allowing for a more rapid and cleaner extraction.
- Robotic Systems: Automation has come a long way; robotic systems can perform high-throughput extractions with minimal human intervention. This not only increases throughput but also minimizes variability between samples.
The benefits of modern methods are apparent:
- Higher DNA yields and purity
- Reduced risk of contamination
- Quicker turnaround times
Overall, as technology continues to advance, applying new extraction methods for high molecular weight DNA becomes more efficient. The careful selection of the technique can heavily influence the outcomes of subsequent experiments, making it a crucial aspect of molecular biology.
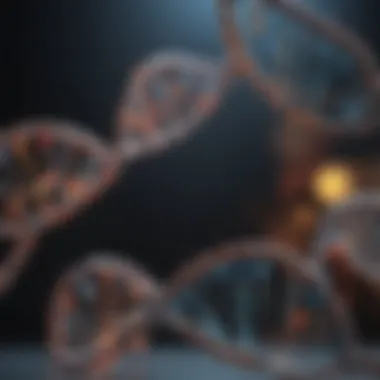
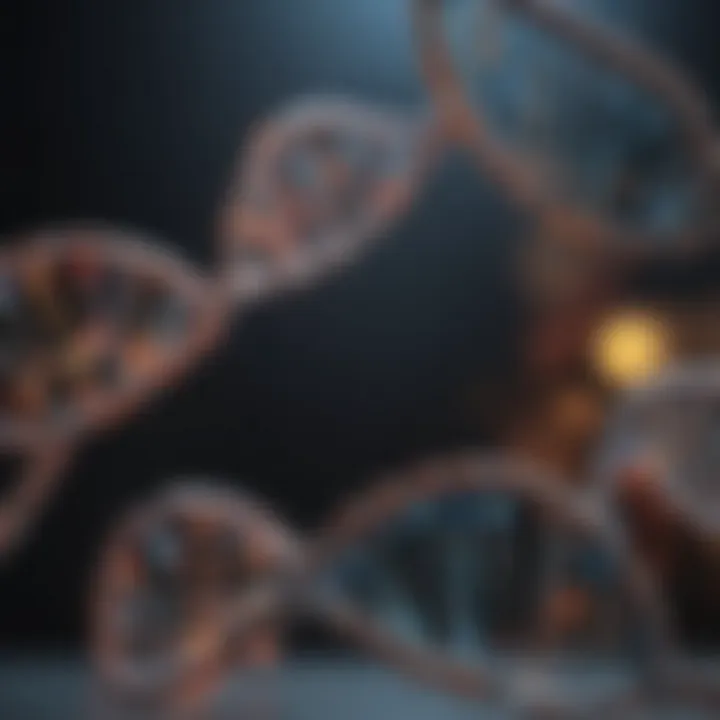
Distinguishing High and Low Molecular Weight DNA
Distinguishing between high and low molecular weight DNA is not just academic; it's fundamental in understanding how DNA operates in various biological contexts. Molecular weight, determined by the length and structure of DNA molecules, significantly influences their functionality. When we consider genomic sequences, for instance, longer sequences often play crucial roles in complex processes, such as regulation and expression.
This section explores critical aspects that highlight the distinctions between high and low molecular weight DNA, providing clarity on their implications for research and practical applications.
Comparison of Functional Roles
High molecular weight DNA generally participates in vital functions that low molecular weight forms might not. These differences result from the sheer length and complexity of the molecules they represent. For example, consider the architectural role that high molecular weight DNA plays in chromatin structure. Unlike its shorter counterparts, high molecular weight DNA can form intricate structures that facilitate gene regulation. This property is essential for the proper functioning of cellular processes.
Moreover, high molecular weight DNA is often involved in the development of strategies for gene therapy. Researchers use these larger molecules in constructs designed to introduce corrective genes into the genome to treat disorders. In contrast, low molecular weight DNA often pertains to quick regulatory processes or transient measurements, like plasmids used in cloning.
In this way, the functional roles of high versus low molecular weight DNA not only showcase their biological significance but also inform choices in experimental design. If one is looking at stable gene expression or genomic stability, working with high molecular weight DNA becomes essential.
Impact on Experimental Outcomes
The molecular weight of DNA can deeply influence experimental outcomes in a number of ways. High molecular weight DNA usually demonstrates enhanced stability in reactions like polymerase chain reaction (PCR), where the longer strand might lead to better amplification of target sequences. This enhanced amplification is critical for obtaining sufficient yields for downstream applications like whole genome sequencing.
Also, the length of DNA can affect its interactions with proteins. Large DNA molecules often bind more efficiently to certain proteins, facilitating crucial interactions necessary for processes such as transcription and replication. The presence of high molecular weight DNA can also influence the accuracy and efficiency of gene editing technologies, including CRISPR, where precise cuts are necessary for effective outcomes.
In contrast, low molecular weight DNA can introduce variability in experimental setups. For instance, its shorter nature might lead to unpredictable behavior during synthesis and replication, resulting in incomplete or erroneous products. Thus, distinguishing between these two types of DNA is not merely a technical endeavor; the ramifications on experimental design and interpretation are significant.
"Understanding the distinct roles of high and low molecular weight DNA is crucial to deriving meaningful insights in genomic research."
The analysis here clarifies that the investigation into high and low molecular weight DNA serves as a foundation for choosing appropriate methodologies and enhancing the quality of scientific outcomes.
Applications in Research and Technology
High molecular weight DNA has become a critical player in various fields of research and technology, effectively shaping advancements in molecular biology, genetics, and biotechnology. The diminished focus on its importance often overshadows the unique applications that high molecular weight DNA can provide. Research ventures utilizing these longer strands are pivotal in pushing boundaries of what we understand about genetic materials and their applications in health sciences and beyond. Here’s a closer look at some specific areas.
Genomic Studies
In genomic studies, high molecular weight DNA serves as an invaluable resource. The integrity and length of high molecular weight DNA promote more accurate representations of genomes. Without the fragmentation often found in cases of low molecular weight DNA, researchers can achieve a detailed mapping of genetic sequences. This can enhance the understanding of structural variations and chromosomal arrangements, ultimately aiding in the identification of genetic disorders.
Some key benefits to utilizing high molecular weight DNA in genomic studies include:
- Complete Genome Assembly: Longer DNA sequences facilitate a more complete assembly of genomes, revealing previously hidden genetic information.
- Enhanced Accuracy: With fewer gaps and less ambiguity in sequences, the chances of successful gene identification rise significantly.
- Chromatin Interaction Studies: Longer strands of DNA allow scientists to study chromatin structure, leading to insights about gene regulation and expression.
Researchers harness these capabilities to draw practical insights—such as longitudinal studies that track genetic mutations over generations. This often lends greater clarity in understanding health complexities that are tied to hereditary conditions. As genomic technologies advance, the focus on high molecular weight DNA becomes increasingly significant in achieving a fuller picture of an organism's genome.
"Utilizing high molecular weight DNA is not just a step forward in methodology; it is a leap into new realms of genetic discovery."
Biotechnological Innovations
In the realm of biotechnology, applications of high molecular weight DNA are plentiful and transformative. From genetic engineering to synthetic biology, longer strands of DNA unlock a wider set of possibilities. High molecular weight DNA provides a framework through which complex biological systems can be modified or engineered for desired functions.
Here are some notable applications:
- Gene Therapy: The longer structures allow for the incorporation of large therapeutic genes in gene therapy applications, potentially treating genetic disorders at a more foundational level.
- Synthetic Genomics: High molecular weight DNA is key in constructing synthetic organisms, enabling scientists to design and assemble entire genomes from scratch, which can lead to the development of biofuels, pharmaceuticals, and more.
- CRISPR Innovations: In CRISPR technology, high molecular weight DNA allows precise editing and alteration of genes, paving a path toward sophisticated agricultural and medicinal improvements.
These biotechnological applications highlight not only the diversity of uses for high molecular weight DNA but also how it represents an essential aspect of advancements aimed at solving complex biological challenges in today's world. As we progress, the potential for high molecular weight DNA to catalyze innovations remains bright and vast.
Challenges in Working with High Molecular Weight DNA
Working with high molecular weight DNA comes with its own set of hurdles that can often be as daunting as they are complex. Understanding these challenges is essential, as they can impact not just research outcomes, but also the interpretations and applications of the findings derived from such DNA. The stakes are high, especially in fields like genetics and biotechnology where precision is paramount. This section will focus on two major issues: degradation risks and contamination problems.
Degradation Risks
High molecular weight DNA is often sought after for its role in comprehensive genomic studies, but it is also more prone to degradation compared to shorter fragments. Factors such as the source of the DNA and the conditions under which it is stored can greatly influence its stability. For example, DNA extracted from older samples or poorly preserved tissues is likely to show signs of fragmentation. This degradation can throw a wrench in the works when researchers aim for complete genomic analyses.
Aside from environmental factors, biological reactions contribute significantly to DNA degradation. Nucleases, enzymes that break down nucleic acids, can rapidly degrade high molecular weight DNA if not effectively inhibited. Therefore, developing robust protocols that include protective measures such as the addition of buffer solutions, controlled storage temperatures, and minimizing freeze-thaw cycles can prove beneficial in preserving the integrity of this valuable genetic material.
"The effective preservation of high molecular weight DNA is not just a technical challenge, but a fundamental necessity for enabling future research discoveries."
Contamination Issues
Contaminants may seem like a nuisance, but they can be a significant barrier in working with high molecular weight DNA. Impurities can compromise the downstream application, affecting results from sequencing to polymerase chain reaction (PCR) tests. For instance, even minute traces of foreign DNA or proteins can allure unwanted enzymatic activities, leading to erroneous conclusions in genetic studies.
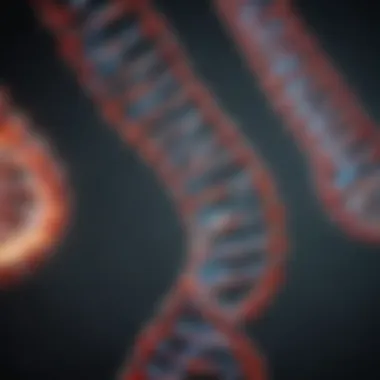
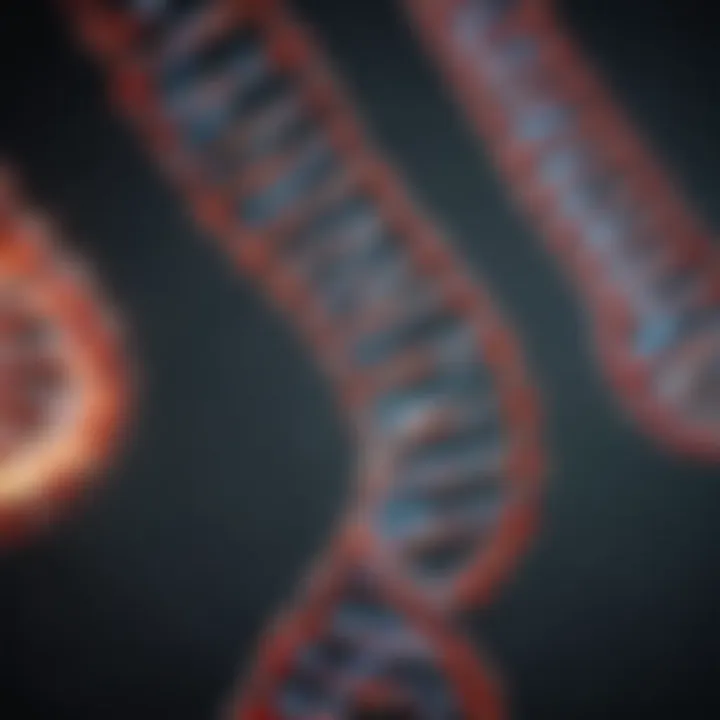
Clean extraction techniques become crucial when isolating high molecular weight DNA. Any lackadaisical approach in laboratory practices can result in contamination. Using quality reagents and ensuring sterility in the workflow are non-negotiable aspects to mitigate these risks. Researchers must be vigilant, employing methods like build-in controls to regularly check for unwanted contaminants in their samples. Also, using specialized kits designed for high molecular weight DNA extraction can vastly reduce contamination issues, leading to purer samples and more reliable outputs.
High Molecular Weight DNA in Disease Research
High molecular weight DNA plays a pivotal role in understanding various diseases. By examining how this type of DNA functions within cells, researchers can uncover vital information regarding genetic disorders and cancer biology. The significance of high molecular weight DNA in this realm cannot be overstated, as it provides the backbone for much of the genetic analysis conducted today.
Genetic Disorders
When it comes to genetic disorders, high molecular weight DNA serves as a critical resource. Many genetic conditions arise from mutations, duplications, or deletions in the DNA sequence. The ability to extract and analyze high molecular weight DNA allows scientists to pinpoint these mutations more accurately. For example, when studying cystic fibrosis, researchers utilize large segments of DNA to identify specific mutations in the CFTR gene.
The detailed analysis of high molecular weight DNA in genetic disorders enables:
- Identification of Genetic Variants: By comparing sequences, scientists can find variants that are associated with particular diseases.
- Development of Gene Therapies: Understanding the mutations provides a pathway for developing potential gene therapies, which may one day correct the underlying genetic issues.
- Risk Assessment: Many disorders have a hereditary component. High molecular weight DNA analysis can assist in determining the likelihood of passing on genetic disorders to offspring.
Meanwhile, certain disorders may exhibit complex inheritance patterns, making high molecular weight DNA vital for understanding multifactorial conditions, such as diabetes or heart disease.
"The role of high molecular weight DNA in uncovering the genetic architecture of diseases is essential for future therapeutic approaches."
Oncology Applications
In oncology, high molecular weight DNA sheds light on cancer mechanisms, progression, and treatment responses. Tumor samples, often enriched in high molecular weight DNA, allow oncologists to study somatic mutations that drive cancer. This analysis is indispensable for developing targeted therapies and understanding tumor heterogeneity.
The applications in oncology include:
- Characterization of Tumor Genomes: High molecular weight DNA from tumors can reveal specific mutations that offer insights into cancer types and their aggressiveness.
- Monitoring Treatment Efficacy: By analyzing circulating tumor DNA (ctDNA), which is often high molecular weight, clinicians can monitor how a tumor responds to treatment and adjust therapeutic strategies as needed.
- Personalized Medicine: Understanding the genetic makeup of a patient’s tumor enables personalized approaches, tailoring treatments to individual genetic profiles for better outcomes.
This ability to utilize high molecular weight DNA in oncology not only improves our grasp of cancer biology but also enhances the precision with which we can approach cancer treatment in patients. As ongoing research unfolds, the importance of high molecular weight DNA in disease research will only continue to grow.
Future Directions in Research
Research into high molecular weight DNA continues to open new frontiers in genetics and molecular biology. As scientists delve deeper into this fascinating subject, the potential unfolds with greater clarity, revealing numerous avenues worth exploring. Understanding these future directions is essential, as they hold the power to enhance our comprehension and treatment of various genetic disorders, improve biotechnological innovations, and refine techniques in molecular research.
This section will focus on two critical areas: the emerging technologies that are radically transforming the landscape of high molecular weight DNA manipulation and the potential discoveries that arise from these advancements.
Emerging Technologies
The pace of innovation in the field of biotechnology reveals how quickly emerging technologies can influence research surrounding high molecular weight DNA. Consider the rise of long-read sequencing technologies such as those provided by Pacific Biosciences and Oxford Nanopore Technologies. These enhancements allow for more accurate readouts of extensive DNA sequences compared to traditional methods like Sanger sequencing.
The integration of CRISPR-Cas9 gene editing with high molecular weight DNA also stands out. Researchers can target specific sequences within larger genomes, offering precise modifications that could lead to breakthroughs in therapeutic developments. Other promising technologies include:
- Nanopore sequencing: This method provides real-time analysis of stretching DNA strands, offering a unique perspective on molecular dynamics.
- Synthetic biology: By applying engineered DNA assembly methods, scientists can construct and modify entire genomes for better functional insights.
- Machine learning algorithms: Sophisticated data analysis techniques are beginning to emerge, easing the interpretation of large genomic datasets. The potential to predict the outcomes of gene alterations can accelerate research and application in clinical environments.
Adopting these tools will be essential in harnessing the full power of high molecular weight DNA and pushing the envelope of what’s possible.
Potential Discoveries and Implications
The intersection of high molecular weight DNA and emerging technologies could lead to numerous discoveries that reshape our understanding of genetics and medicine. For instance, advancements in these fields may result in:
- Accelerated drug discovery: By harnessing high molecular weight DNA to identify and validate new therapeutic targets, the pathway from research to clinical application can become substantially faster.
- Tailored gene therapies: Enhanced precision in altering DNA could lead to individualized treatments for genetic disorders, making cures more attainable and effective.
- Broader insights into genetic evolution: Utilizing long-read sequencing can reveal structural variations in genomes that were previously hidden, allowing researchers to map evolutionary trajectories with greater accuracy.
"The future of high molecular weight DNA research is not just an academic exercise; it’s the key to advancing medical science and personalized medicine."
As these technologies evolve, so too will their application in research settings. This treatment of high molecular weight DNA stands to redefine our understanding and capability in areas from genetic disease to cancer treatment, ensuring a continual push towards innovative solutions. By embracing these advancements, the scientific community is positioned to make profound contributions to human health and understanding of biology.
Finale
The significance of high molecular weight DNA is multifaceted, impacting various domains within genetics and biotechnology. This comprehensive look at the topic has brought to light the intricate relationships between molecular weight, DNA functionality, and the methodologies employed for its extraction. In this concluding section, we will highlight key findings and discuss their broader implications for future research.
Summary of Key Findings
In examining high molecular weight DNA, several pivotal elements emerge:
- Definition and Characteristics: High molecular weight DNA, generally exceeding 50 kilobases in size, exhibits unique physical and chemical properties that contribute to its stability and reliability in experimental setups.
- Extraction Techniques: A range of methods, both conventional and modern, have been detailed, emphasizing the ingenuity required to preserve the integrity of high molecular weight DNA during isolation.
- Functional Roles: Differences between high and low molecular weight DNA have implications in gene expression and regulation, thus influencing experimental outcomes significantly.
- Applications in Disease Research: High molecular weight DNA plays a crucial role in the detection and understanding of genetic disorders, as well as cancer research, establishing a basis for future developments in gene therapy.
Furthermore, the challenges identified, like degradation risks and contamination issues, underscore the need for meticulous handling and processing protocols.
Implications for Future Research
The trajectory of high molecular weight DNA research is promising and continues to evolve. Here are some implications drawn from the findings:
- Technological Innovations: The ongoing development of superior extraction methods could lead to more fruitful research outcomes, enabling researchers to access previously untapped genomic data. This includes harnessing high molecular weight DNA for CRISPR gene editing and other next-generation sequencing techniques.
- Understanding Genetic Mechanisms: Future studies can unravel the roles of high molecular weight DNA in complex traits and diseases. This understanding may foster the development of tailored therapeutic strategies.
- Biotechnological Applications: With continued research, high molecular weight DNA could pave the way for breakthroughs in synthetic biology and bioengineering, steering advancements in agricultural biotechnology and precision medicine.
In sum, as we grapple with the complexities of DNA and its myriad roles in health and disease, the insights gathered from this article prompt a reevaluation of research frameworks. High molecular weight DNA is not merely an entity to be studied; it is a vital key in unlocking the mysteries of biological function and therapeutic innovation.