A Comprehensive Overview of HPLC Methodology
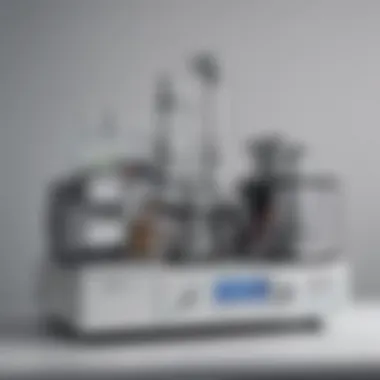
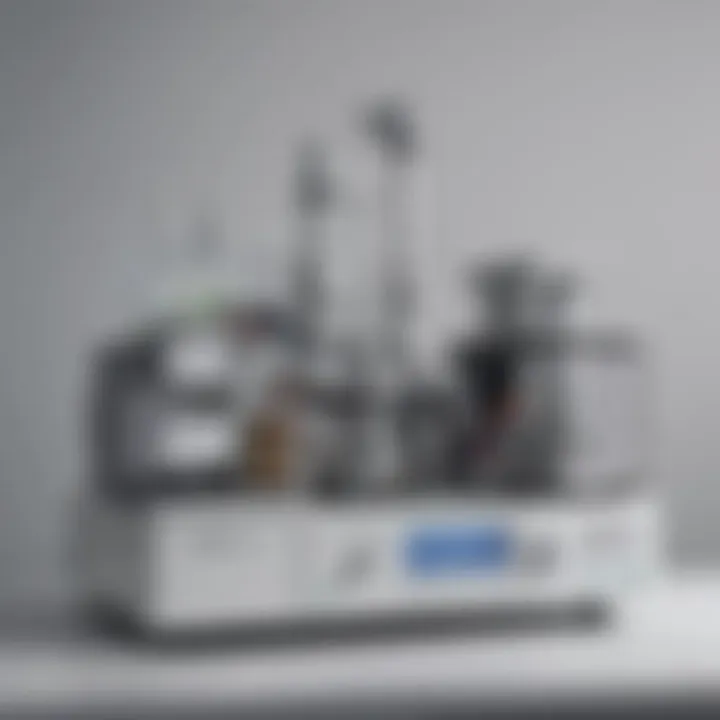
Intro
High-Performance Liquid Chromatography (HPLC) stands as a cornerstone in the realm of analytical chemistry. Its intricate processes enable researchers to separate, identify, and quantify components in complex mixtures with exceptional precision. This methodology finds relevance across various scientific domains, from pharmaceuticals to environmental science. In this article, a thorough examination of HPLC will be undertaken, addressing its fundamental goals, methodologies, and the implications of modern advancements.
Research Methodology
Description of Research Design and Approach
The research approach in HPLC involves systematic exploration of its operational principles. This starts with understanding the basic components that make up an HPLC system. Key parts include the solvent delivery system, autosampler, column, detector, and data analysis software. Researchers often aim to optimize separation efficiency and detection sensitivity. For many studies, a comprehensive analysis includes comparative methodologies, elucidating varying HPLC techniques such as reverse-phase, normal-phase, and size-exclusion chromatography.
Materials and Methods Used in the Study
In conducting HPLC experiments, several materials are commonly utilized. Solvents such as methanol, acetonitrile, and water are regularly used as mobile phases. They are selected based on their polarity, viscosity, and compatibility with the analytes. Columns packed with specific stationary phases play a crucial role in achieving efficient separation.
Furthermore, selecting appropriate detectors is essential for data gathering. UV-Vis, fluorescence, and mass spectrometry detectors are popular choices and must align with the analyte characteristics.
"The versatility of HPLC lies in its ability to adapt to numerous chemical and physical properties of substances, enhancing its value in research."
Discussion and Interpretation
Interpretation of Results in the Context of Existing Literature
When results are obtained, they must often be analyzed against existing literature. This not only validates the findings but also explores discrepancies or confirmations of previously published data. Many researchers emphasize the importance of replicability and precision in their HPLC studies, revealing data that can prompt further exploration of chemical interactions or stability.
Implications for Future Research or Practical Applications
The implications of HPLC research extend well into practical applications. By refining methodologies, researchers can improve drug formulations, enhance purity testing, and delve deeper into metabolic pathways. Advancements, such as the integration of automation in HPLC procedures, promise greater throughput and efficiency, catering to the demands of high-throughput laboratories.
Prolusion to HPLC
High-Performance Liquid Chromatography, or HPLC, is a critical analytical technique employed across various scientific disciplines. Its significance lies in its ability to separate, identify, and quantify components in a mixture with high precision and speed. In this section, we will delve into the definition and historical context of HPLC, shaping the groundwork for understanding its methodology and applications.
Definition of HPLC
High-Performance Liquid Chromatography refers to a form of liquid chromatography that uses high pressure to push the mobile phase through the column. The term "high-performance" highlights the efficiency and effectiveness of the method compared to traditional liquid chromatography. HPLC operates under specific conditions that optimize the separation process.
The system consists of several components including a pump, injector, column, and detector. The main advantage of HPLC is its versatility; it can analyze a wide range of substances, from small molecules to large biomolecules. Notably, it is essential in sectors such as pharmaceuticals, environmental monitoring, and food safety.
Historical Context of HPLC Development
The evolution of HPLC can be traced back to the mid-20th century. Early chromatoraphic techniques were simplistic and lacked the precision seen today. However, the introduction of high-pressure systems in the 1960s marked a turning point in chromatography. The adoption of packed columns and advancements in detectors significantly improved separation efficiency and analysis speed.
The landmark innovation was the development of the first commercial HPLC system in the 1970s, which propelled this technology into various research domains. Since then, continuous improvements have led to the establishment of standardized methods, enhancing reliability and reproducibility in research and industrial settings.
Understanding this context is crucial, as it highlights the changing landscape of analytical chemistry and the pivotal role HPLC has played in its advancement. The ongoing refinement of HPLC technology suggests a promising future where precision analytics meet the growing demands of scientific inquiry.
Principles of High-Performance Liquid Chromatography
High-Performance Liquid Chromatography (HPLC) serves as a cornerstone of modern analytical chemistry. Understanding the principles that govern HPLC is essential to grasp its widespread use in various scientific fields. The efficiency of HPLC lies in its ability to separate complex mixtures into individual components based on their distinct physical and chemical properties. This differentiation enables researchers to perform detailed analysis and quantification of substances in samples, a necessity in areas such as pharmaceuticals and environmental science.
Basic Components of an HPLC System
To appreciate the effectiveness of HPLC, one must comprehend its basic components. These elements work in tandem to facilitate the separation and analysis of samples.
Solvent Reservoir
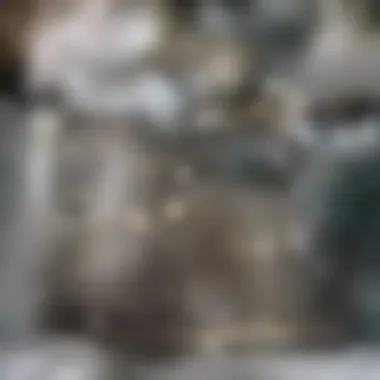
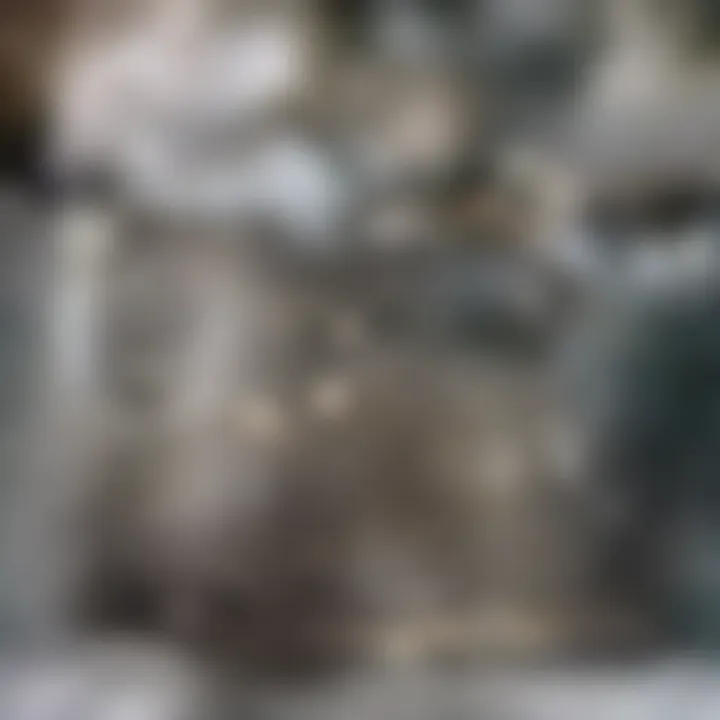
The solvent reservoir stores the mobile phase, which is crucial in HPLC. It allows for the consistent delivery of solvents, ensuring that the flow rate remains stable during the separation process. This component is vital because any fluctuation in solvent composition can lead to variations in results. The key characteristic of a solvent reservoir is its ability to maintain a constant supply of solvent, which is essential for reliable data collection. Polypropylene is a popular choice for these reservoirs due to its durability and resistance to various solvents. However, one disadvantage is that cleaning can be time-consuming if residues form.
Pump
The pump is another critical part of an HPLC system. It generates the necessary pressure to propel the mobile phase through the system. High-pressure pumps are crucial for achieving the flow rates needed for efficient separation. A common choice among professionals is the binary pump, which allows for mixing two solvents in different ratios. This feature provides flexibility in method development. A downside, however, may be that these pumps require regular maintenance to ensure optimal performance.
Injector
The injector is responsible for delivering sample into the HPLC system. It needs to act quickly and accurately to ensure precision in injections, which is paramount in analytical procedures. Auto-samplers are popular because they can inject multiple samples without manual input, increasing efficiency and reproducibility. The unique feature of autosamplers is their ability to handle a large number of samples with minimal human intervention. However, they can be costly and may complicate initial setup.
Column
The column is where the actual separation of components occurs. It contains the stationary phase, which interacts with the analytes as they flow through. Different types of columns can be used depending on the method and sample type. For example, reverse-phase columns are widely used for non-polar compounds. The key characteristic here is the length and diameter of the column, which can influence resolution and analysis time. The main downside, though, is that columns can be sensitive to the mobile phase, requiring careful selection of solvents.
Detector
The detector measures the separated components as they elute from the column. Common detectors include UV and fluorescence detectors, each suitable for different types of analytes. The key characteristic of a detector is its sensitivity and selectivity for specific analytes. A UV detector, for example, is favored for its ability to detect a wide range of compounds. Nonetheless, it may not detect some species that do not have UV-absorbing properties, which can limit its applicability in certain situations.
Mechanisms of Separation
The effectiveness of HPLC relies on various mechanisms of separation, each providing unique benefits and considerations.
Adsorption
Adsorption is a mechanism based on the interaction between the stationary phase and sample components. In this method, different compounds adhere to the stationary phase, separating based on their affinity. This mechanism is beneficial due to its ability to offer high resolution in separating compounds with similar properties. However, it can be less effective for very polar compounds that may elute too quickly.
Partitioning
Partitioning involves the distribution of a compound between two immiscible phases. It is particularly useful when separating compounds based on their polarity differences. One key characteristic is the capability to achieve high selectivity, making it a popular choice. However, it may require optimization of the mobile phase composition to achieve the desired results.
Ion-Exchange
Ion-exchange separation is based on the charge of the analytes. Anionic or cationic substances are retained on oppositely charged stationary phases. This mechanism allows for precise manipulation of separation parameters based on pH and ionic strength. It is notably useful for biomolecules like proteins. The main drawback is that it needs careful adjustment of conditions to avoid unsatisfactory separation.
Size Exclusion
Size exclusion chromatography separates compounds based on their size. Larger molecules elute faster while smaller molecules are retained longer. This feature makes it ideal for purifying proteins or polymers. Despite its efficiency, the resolution can be lower when comparing smaller fragments, thus requiring the use of high performance equipment for optimal results.
Elution Techniques
Finally, different elution techniques influence the outcome of the HPLC analysis. Each technique has its place in method development.
Isocratic Elution
Isocratic elution maintains a constant composition of the mobile phase throughout the separation. It is advantageous for straightforward separations with well-defined components. The simplicity of this approach is a significant benefit as it generally results in shorter analysis times and lower costs. However, it may not effectively separate components that have significantly different retention times.
Gradient Elution
Gradient elution involves changing the composition of the mobile phase over time. This technique is particularly useful for complex mixtures where different components require varying conditions for effective separation. A primary feature of gradient elution is its ability to analyze multidimensional samples effectively. Yet, its complexity can lead to increased method development time and potential inconsistencies between runs.
Applications of HPLC
High-Performance Liquid Chromatography is a cornerstone in analytical chemistry, serving various industries and scientific fields. Its applications are expansive, influencing pharmaceutical research, environmental monitoring, food safety, and biotechnology. Each sector benefits from HPLC's precision in separating and quantifying compounds, making it an invaluable analytical technique.
Pharmaceutical Industry
In the pharmaceutical sector, HPLC plays a critical role in drug development and quality control. It ensures that active pharmaceutical ingredients (APIs) are pure and meet regulatory standards. By performing assays to measure drug concentration and purity, HPLC assists in stability testing and evaluating the validity of new formulations. The ability to separate complex mixtures into individual components allows researchers to analyze the pharmacokinetics and metabolism of drugs, making HPLC essential for developing safe and effective medications.
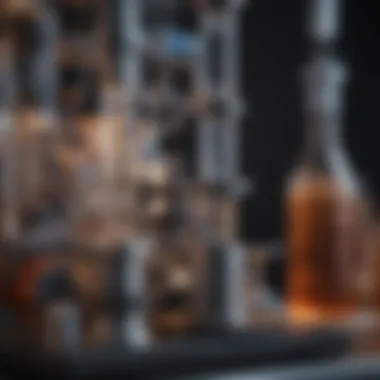
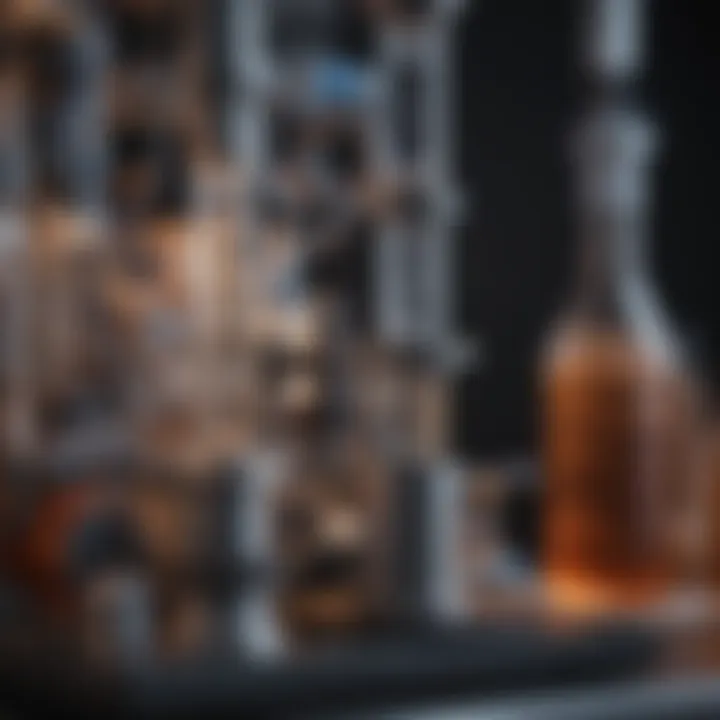
Environmental Analysis
Environmental monitoring increasingly relies on HPLC for assessing pollutants in air, water, and soil. Through its sensitive detection capabilities, HPLC quantifies contaminants at low levels, providing data essential for public health and environmental protection. For example, analyzing pesticides in water samples or determining heavy metal concentrations in soil requires precise techniques. HPLC's role ensures compliance with environmental regulations and provides researchers the tools needed to address ecological issues.
Food and Beverage Testing
The food industry utilizes HPLC for quality assurance and regulatory compliance. Testing for additives, preservatives, and contaminants is crucial to ensure consumer safety. HPLC analyzes the nutritional content of food products, quantifying vitamins and other key nutrients. Manufacturers rely on HPLC to maintain product consistency and to validate label claims. Without HPLC, guaranteeing the integrity and safety of food supply would be significantly more challenging.
Biotechnology Applications
In biotechnology, HPLC is utilized for protein purification and characterization. Researchers depend on HPLC to isolate enzymes, antibodies, and other biomolecules essential for diagnostics and therapeutics. Its ability to analyze complex biomolecular mixtures enables scientists to understand interactions at the molecular level. This knowledge facilitates the development of new therapies, vaccines, and diagnostic tools, underscoring HPLC’s importance within the field.
"Technical expertise and precision in measurement define the advantages of HPLC across diverse applications."
HPLC's multifaceted applications highlight its relevance in today's scientific landscape. Each application demonstrates how this technique supports essential research, thereby driving innovations across various fields.
HPLC Method Development
HPLC Method Development is critical in the realm of analytical chemistry. This phase typically involves devising and fine-tuning the conditions under which liquid chromatography is performed. The primary goal is to achieve optimal separation, identification, and quantification of the analytes of interest. Without rigorous method development, the results obtained from HPLC would lack reliability and reproducibility, which are paramount in scientific research.
The complexity of samples analyzed demands a structured approach to method development. Common considerations include the nature of the analytes, the type of matrix, and the desired outcome of the analysis. A systematic approach allows scientists to identify the best working conditions that accommodate the specifics of their study, ultimately enhancing the overall quality of the data produced.
Parameter Optimization Strategies
Mobile Phase Selection
Mobile phase selection is a fundamental aspect of the HPLC methodology. Selecting the right mobile phase ensures that the analytes are sufficiently solubilized and can interact ideally with both the stationary phase and the mobile phase to achieve effective separation. The key characteristic of this selection is its ability to influence the resolution, retention time, and overall efficiency of the chromatography process.
A beneficial choice often includes a mixture of water and organic solvents such as acetonitrile or methanol. These solvents provide a good balance between polarity and viscosity, which is essential for achieving optimal performance. However, careful consideration must be made towards the sample's chemical properties, as some compounds may require unique or specialized mobile phases to achieve suitable separation.
Column Temperature Adjustment
Column temperature adjustment is another significant factor in HPLC method development. This aspect relates to how temperature variations can affect retention times, peak shapes, and overall analysis time. The key characteristic of temperature control is that it can improve the reproducibility of results. A stable temperature eliminates variability caused by fluctuating conditions, making it a popular choice in method development.
Unique features include the potential to reduce viscosity of the mobile phase. A lower viscosity increases flow rates, which can enhance productivity. Nonetheless, higher temperatures might cause decomposition of sensitive analytes, presenting a disadvantage in some scenarios.
Flow Rate Modulation
Flow rate modulation involves adjusting the speed at which the mobile phase travels through the column. This parameter is crucial, as it affects the time analytes spend in contact with the stationary phase, which in turn influences separation efficiency. The key characteristic of optimal flow rates is their capacity to reduce analysis time while maintaining resolution.
Higher flow rates may lead to shorter analysis times but can also decrease the resolution if not managed correctly. Therefore, a balance must be found. An advantage of flow rate modulation is its ability to improve throughput in laboratories that require analysis of numerous samples in a limited timeframe.
Validation Protocols
Validation protocols ensure that the developed HPLC methods yield reliable and accurate results. The significance of this stage cannot be overstated. It provides the framework for assessing the quality and integrity of analytical results.
Accuracy
Accuracy reflects the closeness of measurements to the true value. A high level of accuracy is essential in analytical methods as it builds trust in the results produced. The validation of accuracy involves comparing results against known standards or reference materials, making it a beneficial practice in method development. Its role in enhancing confidence cannot be ignored, although it requires rigorous testing and calibration to achieve.
Precision
Precision refers to the degree of agreement among repeated measurements. High precision indicates that the method will consistently yield the same results under unchanged conditions. It is a beneficial attribute as it assures the reliability of the data. The challenge lies in how to construct experiments that are reproducible and avoid analytical errors, which may affect precision.
Linearity
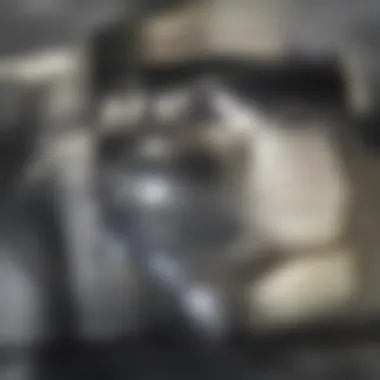
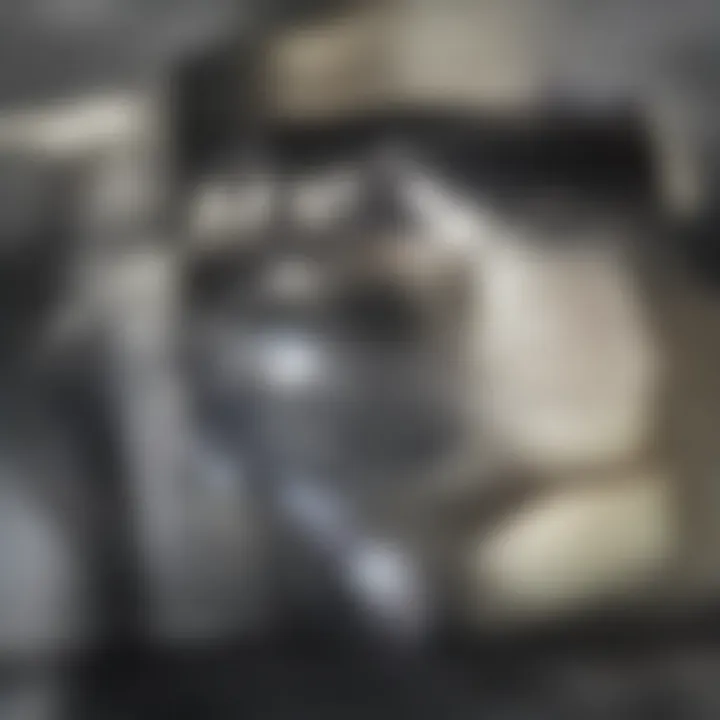
Linearity demonstrates the method's ability to produce results that are directly proportional to the concentration of analytes across a specified range. Establishing linearity is crucial as it informs the appropriate concentration range for quantifying the target analytes. The main advantage of a linear method is its straightforward interpretability. However, linearity must be verified through exhaustive testing for each new method to ensure it achieves valid results.
Specificity
Specificity ensures a method effectively separates the analyte from other components in the sample matrix. This aspect is particularly important in complex analytical environments where interferences may occur. A highly specific method will enhance trust in the results by ensuring that quantification is accurate and not affected by the presence of other substances.
However, achieving a balance in specificity might lead to prolonged method development times. The unique feature of specificity lies in its ability to discern closely related analytes and interference compounds in complex mixtures.
Method development in HPLC should always be comprehensive. Individual merits found within parameters play a crucial role in ensuring successful analysis.
Challenges and Limitations of HPLC
High-Performance Liquid Chromatography (HPLC) is a powerful analytical technique, yet it encounters several challenges that can limit its effectiveness and applicability. Understanding these challenges is crucial, as they can affect the choice of method, the reliability of results, and overall research outcomes. This section discusses sample complexity and instrument limitations, both significant factors in HPLC use.
Sample Complexity
Sample complexity refers to the diverse and intricate nature of samples being analyzed. In many scenarios, samples may contain a wide range of components that differ in polarity, molecular weight, and size. This diversity poses challenges in achieving effective separation. For example:
- Matrix Effects: Compounds from the sample matrix can interfere with the detection and quantification of target analytes. This is especially common in biological samples.
- Overlapping Peaks: In a complex mixture, important analytes may have similar retention times. This overlap can make it difficult to quantify individual components accurately.
- Concentration Variability: The concentration of target substances may vary greatly, requiring careful calibration and method optimization. Different sample preparation techniques might be necessary to mitigate these issues.
Researchers must account for these factors before developing an HPLC method. Supplementary techniques, like sample pre-treatment or using extraction methods, might help simplify the analysis.
Instrument Limitations
While HPLC instruments are highly developed, they are not free from limitations. Understanding these constraints is essential for effective application in various research areas:
- Sensitivity: Not all detectors provide the same level of sensitivity. Some analytes may be present in trace amounts, which could lead to undetectable levels if a suitable detector is not used.
- Maintenance: HPLC systems require regular maintenance and calibration to ensure optimal performance. Neglecting this aspect can lead to drifts in baseline and poor reproducibility.
- Cost: High-quality HPLC systems, columns, and consumables are often expensive. This can be a barrier for many laboratories, particularly those in developing regions or smaller institutions.
Acknowledging these challenges is a step toward enhancing the reliability and effectiveness of HPLC methodologies, enabling researchers to leverage its full potential in various scientific domains.
Future Trends in HPLC Technology
High-Performance Liquid Chromatography (HPLC) continues evolving, driven by innovations and the need for more efficient and precise analytical methods. As research demands escalate, understanding future trends in HPLC technology becomes essential. This section explores significant developments that promise to shape the future of HPLC, highlighting miniaturization and integration with mass spectrometry.
Miniaturization of HPLC Systems
The miniaturization of HPLC systems represents a significant trend in the field. Smaller systems offer portability and ease of use, becoming suitable for a broader range of applications. This trend is important for both academic and industrial laboratories.
- Reduced Sample Size: Smaller HPLC systems require less sample volume, making them ideal for precious or limited substances. This contrasts with traditional setups that often require larger quantities.
- Cost Efficiency: Less solvent and fewer resources are necessary for operation. This not only reduces costs but also minimizes waste, aligning with growing sustainability efforts in research practices.
- Increased Throughput: Miniaturized systems often allow for faster analysis times. Quick analyses expand the capability of labs to conduct numerous tests within a short period. This is valuable in fields where timely results are critical, such as clinical research or environmental monitoring.
- Enhanced Resolution: With advancements in technology, miniaturized columns can offer improved separation performance. This clarity in results pushes forward the analytical capabilities, catering especially to complex matrices.
"Miniaturization is not just about reducing size. It is about enhancing performance while minimizing resource usage."
In summary, miniaturization can change the landscape of HPLC applications. The ability to conduct quality analyses without extensive laboratory infrastructure is transformative, especially for field applications.
Integration with Mass Spectrometry
Another crucial trend is the integration of HPLC with mass spectrometry (MS). This combination is increasingly popular due to the high sensitivity and precise quantification that both techniques offer collectively.
- Enhanced Detection Limits: When combined with MS, HPLC provides the ability to detect compounds at very low concentrations. This is particularly useful in pharmacokinetic studies and biomarker discovery.
- Comprehensive Data Acquisition: This integration results in richer datasets. HPLC separates compounds efficiently, while MS identifies and quantifies them accurately, leading to a more complete understanding of sample composition.
- Versatile Applications: The coupling of HPLC and MS is versatile. It is employed in diverse areas, such as proteomics, metabolomics, and environmental analysis. The flexibility allows researchers to adapt methods for specific analytical challenges.
- Streamlined Workflow: Automated systems with integrated HPLC and MS streamline workflows. This improvement in efficiency saves time and labor, permitting focus on data interpretation rather than manual operations.
These trends reflect a commitment to enhancing the efficacy of analytical methods in HPLC, ensuring it remains at the forefront of scientific research.
Finale
In the realm of analytical chemistry, High-Performance Liquid Chromatography (HPLC) stands as a cornerstone technique, offering unmatched precision and versatility. The conclusion of this article serves to underscore its importance and relevance across various scientific domains. HPLC facilitates effective separation and analysis of complex mixtures, making it indispensable in industries such as pharmaceuticals, environmental science, food testing, and biotechnological applications.
Summarizing Key Takeaways
- HPLC Overview: High-Performance Liquid Chromatography is based on the principle of separation, utilizing a liquid phase to transport analytes through a chromatographic system.
- Applications: Its applications span numerous fields, making it relevant for professionals in various sectors. Each application leverages its capacity for detail and accuracy.
- Method Development: Developing HPLC methods is crucial for ensuring precise results. Considerations such as parameter optimization and validation protocols must be carefully addressed.
- Challenges: Various challenges exist, like sample complexity and the need for appropriate instrument selection, which researchers must navigate to achieve reliable outcomes in their analyses.
The Significance of HPLC in Modern Research
High-Performance Liquid Chromatography plays a vital role in advancing scientific inquiry and innovation. Its precise methods provide insights that drive research forward. Whether separating pharmaceutical compounds for drug formulation or analyzing environmental pollutants, HPLC demonstrates its essential nature in modern research. The ongoing developments in HPLC technology, such as integration with mass spectrometry and miniaturization, signify a trend towards enhanced efficiency and accuracy. This ensures HPLC remains at the forefront of analytical methods used in both academic and industrial settings. Ultimately, the value of HPLC cannot be overstated; it is a critical tool that supports the reliability and efficacy of scientific exploration.