Exploring Induced Pluripotent Stem Cell Reprogramming
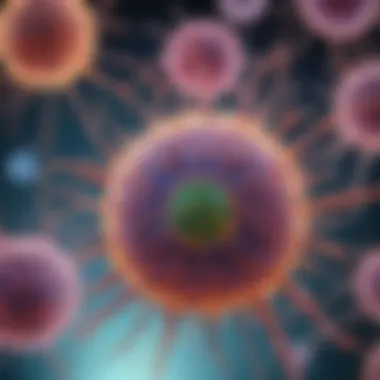
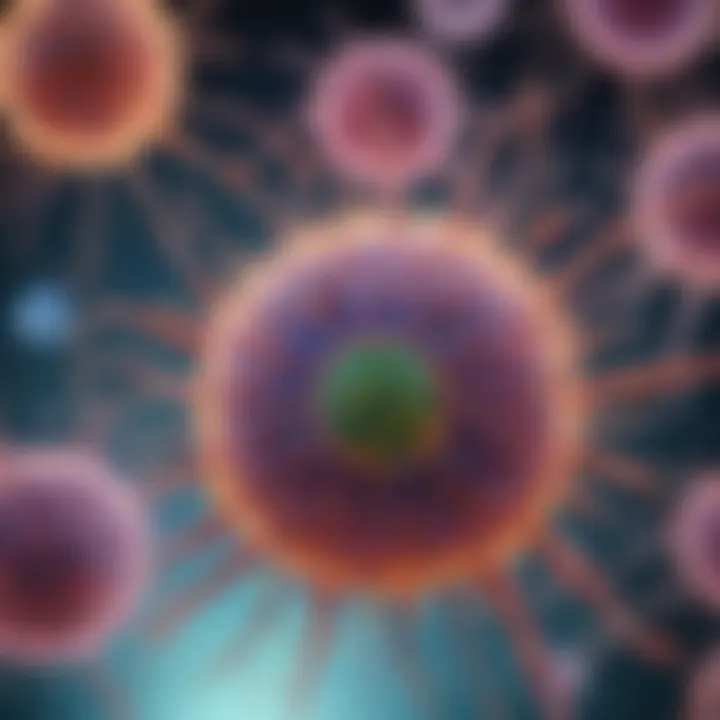
Intro
Induced pluripotent stem (iPS) cells have emerged as a groundbreaking tool in modern biology and medicine. The ability to convert somatic cells into pluripotent stem cells offers immense potential for research, therapy, and our understanding of complex diseases. This significant advancement sheds light on how cells can be reprogrammed and what it means for regenerative medicine. Recent developments in this area have opened new avenues in disease modeling, enabling researchers to study illnesses at a cellular level and discover more targeted treatments.
While the benefits of iPS cell technology are clear, it is also important to consider the potential ethical implications. As scientists push the boundaries of what is possible, they must navigate the moral landscape surrounding stem cell research. This article will explore the various dimensions of iPS cell reprogramming, focusing on the methodologies used, the challenges faced, and the future of this transformative field.
Research Methodology
Description of research design and approach
The research design surrounding the reprogramming of somatic cells to iPS cells typically involves a series of systematic steps. Researchers often use a combination of genomic reprogramming techniques that include viral transduction, plasmid-based methods, or small molecule compounds. Among the most common methodologies are the use of the Yamanaka factors: Oct4, Sox2, Klf4, and c-Myc. These transcription factors are crucial in the transition of differentiated cells back to a pluripotent state.
Materials and methods used in the study
The materials and methods utilized in the study of iPS cell reprogramming include:
- Cell Types: Fibroblasts, blood cells, or other somatic cells are often chosen for their availability and ease of manipulation.
- Reprogramming Vectors: These include lentiviruses, adenoviruses, or integration-free methods like Sendai viruses.
- Culture Conditions: Cells are typically maintained in a specific medium that supports growth and pluripotency. For example, mTeSR1 or a defined serum-free medium is commonly used to enhance the efficiency of reprogramming.
- Selection and Screening: After transduction, researchers often employ drug selection strategies to isolate successfully reprogrammed cells.
This comprehensive approach to research methodology ensures a thorough examination of how iPS cells can be generated and their potential applications in various biomedical fields.
Discussion and Interpretation
Interpretation of results in the context of existing literature
The results stemming from iPS cell research have consistently shown promising outcomes. Various studies have demonstrated that iPS cells can differentiate into any cell type, mirroring the capabilities of embryonic stem cells. However, unique challenges remain, such as ensuring genomic stability and avoiding tumorigenesis. The ongoing research seeks to improve the safety and efficiency of the reprogramming process while maintaining the pluripotent characteristics of iPS cells.
Implications for future research or practical applications
The implications of advancements in iPS cell technology are profound. They extend beyond basic research to practical applications in therapies for neurodegenerative diseases, heart disease, and diabetes. Moreover, iPS cells could revolutionize drug discovery, allowing for more accurate screening of pharmaceuticals on patient-specific cells. Future research should focus on improving the reprogramming techniques and fully understanding the implications of using iPS cells in therapeutic settings.
"The potential of iPS cells is immense, yet it requires careful navigation of both scientific and ethical landscapes to fully harness its capabilities."
Preamble to iPS Cell Reprogramming
Induced pluripotent stem (iPS) cell reprogramming is a groundbreaking development in the field of developmental biology. It allows researchers to revert somatic cells back to a pluripotent state. This transformative process enables these cells to differentiate into any cell type in the human body. With diverse applications, from regenerative medicine to drug discovery, understanding iPS cell reprogramming is essential for students, researchers, and professionals alike.
The advent of iPS technology marks a significant milestone not only for stem cell research but also for therapeutic interventions. The ability to generate pluripotent cells from adult tissues eliminates many ethical issues related to embryonic stem cells. Furthermore, iPS cells offer a novel avenue for modeling diseases and testing new drugs in a personalized manner. Thus, discussing the mechanisms, methodologies, and implications of iPS cell technology is crucial in the ongoing dialogue in biomedicine.
Definition and Historical Background
Induced pluripotent stem cells are defined as somatic cells that have been genetically reprogrammed to an embryonic stem cell-like state. The landmark discovery in 2006 by Shinya Yamanaka and his team at Kyoto University established a new paradigm in cellular biology. Yamanaka identified four transcription factors—Oct4, Sox2, Klf4, and c-Myc—that could induce reprogramming.
Since this breakthrough, iPS cell technology has evolved considerably. Researchers have refined the methods to enhance efficiency and reduce risks associated with genetic manipulation. Over the years, iPS cells have been used extensively to explore various aspects of cell biology and therapeutic applications. This historical backdrop is vital for understanding how far the technology has come and where it might lead in the future.
Significance in Regenerative Medicine
The significance of iPS cell reprogramming in regenerative medicine cannot be overstated. With their pluripotent capabilities, iPS cells hold the potential to regenerate damaged tissues and organs. This offers hope for patients suffering from degenerative diseases such as Parkinson's, diabetes, or heart failure.
Moreover, personalized medicine is becoming feasible with iPS technology. Patients can have their somatic cells reprogrammed into iPS cells, which can then be differentiated into specific cell types for transplantation. This ability to use patient-specific cells significantly reduces the risk of immune rejection.
Furthermore, iPS cells are a powerful tool in disease modeling, enabling researchers to recapitulate disease mechanisms in vitro. This purpose opens new pathways for drug discovery and toxicity testing without the ethical concerns associated with using animal models.
iPS cell technology provides an unprecedented opportunity to bridge the gap between basic research and clinical applications in regenerative medicine.
In summary, the exploration of induced pluripotent stem cell reprogramming underscores its importance in advancing regenerative medicine. The historical context sets the stage for its applications, which are reshaping our understanding and treatment of various diseases.
Understanding the Mechanisms of Reprogramming
Understanding the mechanisms of reprogramming is vital for advancing induced pluripotent stem cell (iPS) technology. This knowledge provides insight into how somatic cells can be converted back into pluripotent cells. This conversion opens up possibilities for various applications in biomedical research. Knowing these mechanisms also helps in addressing challenges faced in the field and enhances efforts to refine reprogramming techniques.
Key Factors Involved in Reprogramming
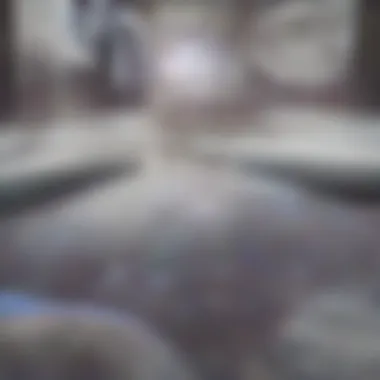
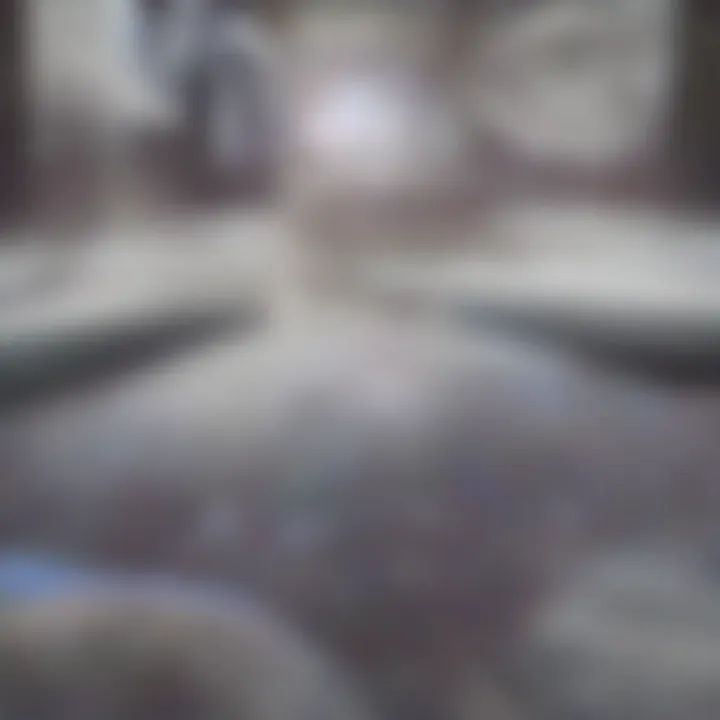
Reprogramming cells requires several critical factors. These factors work together to facilitate the transition of a somatic cell to an iPS cell. The complexity of these interactions can influence the effectiveness of the process.
Transcription Factors
Transcription factors are proteins that enhance or inhibit the transcription of specific genes. They play a crucial role in cellular reprogramming. Key transcription factors like Oct4, Sox2, Klf4, and c-Myc are typically used in this process. The hallmark of these factors is their ability to bind to specific DNA sequences and activate gene expression related to pluripotency. This ability allows them to reprogram somatic cells efficiently. However, the introduction of certain oncogenes like c-Myc can present risks, such as tumor formation.
Epigenetic Modifications
Epigenetic modifications refer to chemical changes to DNA and histone proteins that influence gene expression without altering the DNA sequence. These modifications are essential during the reprogramming process. They can enable or silence gene expressions that promote pluripotency. The key characteristic of epigenetic modifications is their reversible nature, which allows for adaptability in gene expression patterns. This flexibility is particularly advantageous when attempting to achieve stable pluripotency in iPS cells, but it's also a challenging area requiring careful manipulation.
Cellular Microenvironment
The cellular microenvironment consists of various biochemical and physical factors surrounding a cell. This environment significantly influences the reprogramming process. For instance, a supportive microenvironment can enhance the efficiency of converting somatic cells into iPS cells. The key feature of this aspect is that it can vary based on culture conditions, influencing cellular responses. However, the uniqueness of each microenvironment can also introduce complications in achieving consistent outcomes during reprogramming.
Epigenetic Reprogramming Process
The epigenetic reprogramming process is an intricate mechanism that underpins the transition of a somatic cell to a pluripotent state. Understanding this process is fundamental to improving iPS technology.
DNA Methylation Changes
DNA methylation changes involve adding methyl groups to specific sites on DNA. This alteration can silence gene expressions necessary for maintaining a somatic state. During reprogramming to iPS cells, global changes in DNA methylation patterns occur. The main characteristic is that these methylation patterns must be reset to promote pluripotency. Therefore, controlling DNA methylation is crucial for successfully generating stable iPS cells, though this requires precise techniques to avoid unintended silencing of essential genes.
Histone Modifications
Histone modifications involve changes to the proteins around which DNA is wrapped. These changes can significantly impact gene accessibility and transcription. Specific histone modifications can activate or repress genes crucial for pluripotency. The primary advantage of studying histone modifications is their dynamic and reversible nature, making them amenable to manipulation. However, the range of possible modifications can complicate the ability to predict outcomes accurately, necessitating more research and technical refinement in this area.
Studying the mechanisms of iPS cell reprogramming not only furthers scientific understanding but also paves the way for innovative applications across medicine.
Methods of Inducing Pluripotency
The methods of inducing pluripotency are critical for advancing research in induced pluripotent stem (iPS) cell technology. These methods provide a systematic approach to convert somatic cells into pluripotent stem cells. Understanding these techniques is essential for developing effective therapies and studying various diseases.
Pluripotency refers to the ability of a stem cell to differentiate into nearly any cell type in the body, making it a valuable resource in regenerative medicine, drug development, and disease modeling. By exploring different methods, scientists can improve efficiency, minimize risks, and enhance the therapeutic potential of iPS cells.
In this section, we will explore two primary categories of inducing pluripotency: viral vector-based approaches and non-viral approaches, detailing their specific components and contributions to the field.
Viral Vector-Based Approaches
Viral vectors have been extensively employed in the reprogramming of somatic cells to pluripotent status. This section examines two prominent types of viral vectors: lentiviral vectors and retroviral vectors.
Lentiviral Vectors
Lentiviral vectors are a preferred choice for reprogramming due to their ability to integrate into the host genome efficiently. They can transduce non-dividing cells, which broadens their applications in various tissue types.
A significant characteristic of lentiviral vectors is their capability to carry larger genetic materials compared to other viral vectors. This feature allows for the inclusion of multiple reprogramming factors simultaneously. The integrative capacity of lentiviral vectors provides long-term expression of these factors, which is beneficial for sustained reprogramming.
However, these advantages come with potential risks. The insertion of the viral genome can lead to genomic instability or tumorigenesis, raising safety concerns in therapy applications. Therefore, while lentiviral vectors are popular, researchers must carefully evaluate their use in clinical settings.
Retroviral Vectors
Retroviral vectors are another important tool for inducing pluripotency. Their main characteristic is the retroviral integration into the host genome, which allows the stable expression of reprogramming factors. This method gained recognition primarily through the work of Shinya Yamanaka, who used them to generate the first iPS cells.
Retroviral vectors typically have a high infection efficiency, particularly in actively dividing cells, making them suitable for laboratory applications. They are often more simple to generate compared to lentiviral vectors, which appeals to researchers.
On the downside, the use of retroviral vectors poses limitations similar to lentiviral vectors. The risk of insertional mutagenesis remains problematic. Furthermore, they are restricted primarily to dividing cells, which may limit their utility in some applications.
Non-Viral Approaches
Non-viral methods of inducing pluripotency have garnered attention for their potential to mitigate risks associated with viral vectors. This section will cover two notable non-viral approaches: transposon systems and small molecules.
Transposon Systems
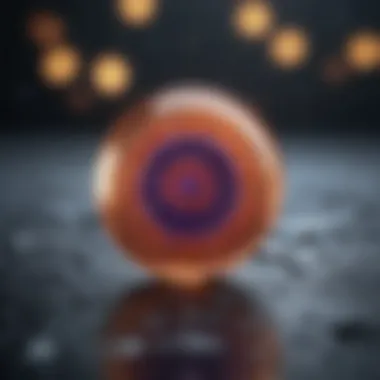
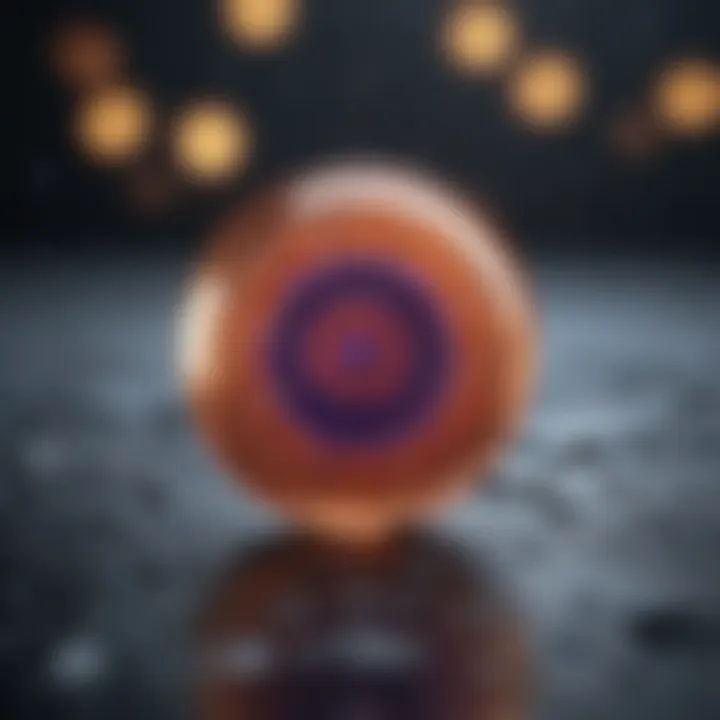
Transposon systems are a robust alternative for delivering reprogramming factors without the accompanying genomic integration issues seen in viral vectors. Transposons, or "jumping genes," can insert themselves into various chromosomal locations, promoting gene expression without permanent alterations.
A primary advantage of transposon systems is their flexibility. They can be easily modified to include different combinations of reprogramming factors. This adaptability makes it simple to test various configurations to optimize pluripotency induction.
Nevertheless, the efficiency of transposon systems can vary, depending on the cell type and selected transposon system. Additionally, the cultural conditions may affect the outcomes, which requires careful management in laboratory settings.
Small Molecules
Small molecules represent yet another innovative strategy in the field of iPS reprogramming. These compounds can modulate signaling pathways and cellular machinery to favor reprogramming. Small molecules can act by enhancing the natural processes of somatic cell reprogramming or by inhibiting differentiation pathways.
One of the main characteristics of small molecules is their low immunogenicity. Unlike viral vectors, small molecules are unlikely to induce immune responses, making them a safer choice. Furthermore, they can be used in combination with other methods to refine the reprogramming process.
However, the primary challenge lies in identifying the right small molecules and establishing effective protocols for their use. The screening process to find optimal candidates can be time-consuming and resource-intensive.
In summary, each method used to induce pluripotency—whether through viral or non-viral approaches—has its advantages and limitations. The diverse strategies open avenues for future advancements in stem cell research and applications, further enhancing the potential of iPS cells.
Applications of iPS Technology
Induced pluripotent stem cells (iPS cells) have revolutionized various facets of biomedical research. The applications of iPS technology span numerous areas, making it invaluable in contemporary science. One of the foremost benefits is its potential for disease modeling, which enables researchers to investigate complex diseases through patient-specific models. This leads to more accurate diagnosis and tailored therapies. Furthermore, cell-based therapies utilizing iPS cells provide the means to regenerate damaged tissues, addressing critical health concerns across diverse medical fields. Finally, iPS cells play a crucial role in drug discovery, helping pharmaceutical companies evaluate new compounds' efficacy and safety efficiently.
Disease Modeling
Neurodegenerative Diseases
Neurodegenerative diseases like Alzheimer’s and Parkinson’s represent a significant challenge in medical research. These conditions are characterized by progressive neuronal loss and complex pathologies that are not fully understood. Utilizing iPS cells sourced from patients with such disorders allows researchers to create models that closely mimic disease conditions in vivo. This specific approach sheds light on the molecular mechanisms underlying these diseases. One distinctive feature of this application is the ability to observe disease progression and cellular behavior over time. This offers substantial advantage for identifying potential therapeutic targets. However, there are some limitations. Models may not always capture the entire complexity of human conditions, making it vital to complement findings with in vivo studies.
Cardiovascular Disorders
Cardiovascular disorders are among the leading causes of morbidity and mortality worldwide. The iPS technology offers a unique approach to study these diseases at a cellular level. By reprogramming somatic cells from patients with heart conditions, scientists can create cardiomyocytes that reflect the patient's pathophysiology. This aspect is particularly beneficial as it allows for personalized study and the testing of treatment responses. The distinctiveness of using patient-derived cells is vital for understanding individual drug responses. There are potential downsides, though. Cellular differences from person to person may lead to variability in research results, making standardization a challenge.
Cell-Based Therapies
Regeneration of Damaged Tissues
The regeneration of damaged tissues is one of the most promising applications of iPS cells. Conditions like spinal cord injuries or severe burns can significantly impact a person’s quality of life. iPS cells provide a renewable source of tissue-repairing cells, which can be directed to differentiate into various cell types needed for regeneration. A key characteristic of this technology is that it can utilize a patient’s own cells, thereby reducing the risk of immune rejection. This becomes a beneficial strategy, especially for personalized medicine approaches. The limitations may include technical difficulties in directing differentiation and maintaining the functionality of regenerated tissues.
Immune Cell Therapy
Another area where iPS technology is making strides is immune cell therapy. iPS cells can be differentiated into various immune cells, paving the way for innovative treatments for conditions like cancer or autoimmune diseases. This specific aspect helps in developing cell therapies tailored to individual patient needs. A distinct benefit is the potential for creating a diverse range of immune cells from a single source. Challenges arise in ensuring these cells maintain their functionality in vivo after transplantation. There is also concern regarding long-term safety and unexpected immune responses that may occur post-therapy.
Drug Discovery and Toxicology Studies
The application of iPS cells in drug discovery offers enormous potential in evaluating the effectiveness of new pharmacological agents. Using patient-derived cells provides insights into how different demographics might respond to new drugs. This aspect allows researchers to predict drug responses based on genetic background and disease state more accurately. Furthermore, toxicology studies benefit from iPS technology by offering a platform for screening drug toxicity before clinical trials. Despite the promise, challenges remain in translating laboratory findings into effective clinical applications.
Advancing iPS technology not only drives scientific discovery but also paves the path towards personalized medicine, offering tailored treatment options to patients based on their unique cellular characteristics.
These applications of iPS technology illustrate the considerable depth and breadth of possibilities it offers in research and clinical settings. Understanding and navigating the complexities of these applications is paramount for the continued evolution of biomedicine.
Challenges in iPS Cell Research
Induced pluripotent stem (iPS) cell research represents a frontier in regenerative medicine. Even with its significant promise, substantial challenges exist. Understanding these challenges is crucial for researchers and professionals engaging in this field. This section will discuss two major challenges: genetic stability and tumorigenicity, along with technical limitations in reprogramming.
Genetic Stability and Tumorigenicity
One of the most pressing issues in iPS research is ensuring genetic stability. When somatic cells are reprogrammed back to a pluripotent state, they must maintain genetic integrity. However, during the reprogramming process, mutations can occur. Research indicates that iPS cells may exhibit karyotypic abnormalities and genetic drift over time.
Tumorigenicity is another concern. Tumorogenic cells can arise due to mutations or incomplete reprogramming. If not controlled, these cells can contribute to tumor formation upon transplantation. Data suggests that enhancing genetic stability should be a priority in iPS cell applications. Various studies advocate stringent genetic monitoring to mitigate risks associated with tumorigenicity in these cells.
"Genetic stability is a cornerstone for the safe application of iPS cells in clinical settings."
Ensuring that iPS cells are genetically sound is vital for both research and clinical use. This involves being meticulous about the cell lines used and continually assessing mutation rates.
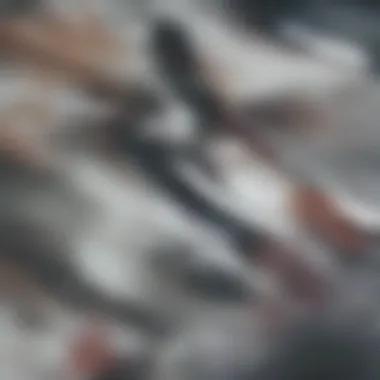
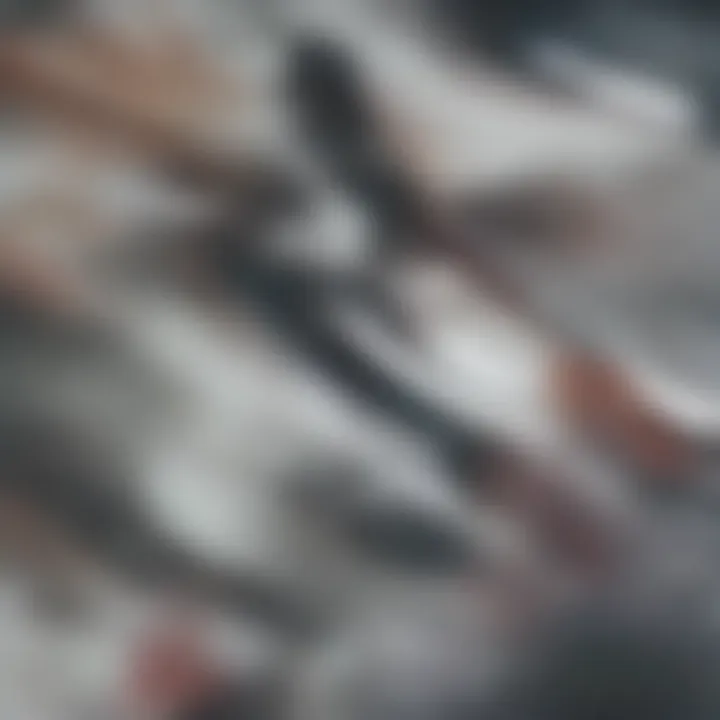
Technical Limitations in Reprogramming
While there are several methods to induce pluripotency, each comes with distinct technical hurdles. Viral vector-based approaches, for example, are effective but carry risks associated with insertional mutagenesis – a process where the introduction of the viral DNA into host DNA can disrupt normal cellular functions.
On the other hand, non-viral methods, like small molecules or transposon systems, show promise. Yet, these techniques often struggle with efficiency and reproducibility. Variability exists in outcomes depending on cell type and reprogramming protocols. The challenge remains to develop methods that are both safe and reliably effective across diverse somatic cell types.
Advancements in technology may help mitigate these challenges. Continued research and optimization of reprogramming techniques is required to improve efficiency, stability, and safety. This is paramount for the integration of iPS cells into therapeutic applications and for their legitimate use in personalized medicine.
Ethical Considerations in iPS Research
The topic of ethical considerations in induced pluripotent stem (iPS) cell research is fundamental to understand its impact on society and science. This section addresses key ethical issues that arise from the use of iPS cells in research and their potential applications in medicine. As iPS cells hold transformative potential in regenerative medicine, it is crucial to navigate the ethical landscape that accompanies their development and usage. Ensuring ethical integrity not only protects individuals involved in research but also encourages public trust in scientific innovations.
Source of Somatic Cells
Induced pluripotent stem cells are derived from somatic cells. The choice of these cells raises ethical questions regarding their sourcing. Somatic cells can be obtained from various tissues, including skin, blood, and other readily accessible locations in the body. While this process might seem straightforward, it prompts discussions surrounding consent, ownership of biological materials, and potential commercialization of human tissues.
When obtaining somatic cells, it is crucial to ensure that the individual providing the sample gives informed consent. This means that they are fully aware of how their cells will be used, the potential risks involved, and the implications of their use in research and therapy. Informed consent represents a cornerstone of ethical research practices and respects the autonomy of individuals.
Moreover, ethical concerns may arise if the cells are collected from vulnerable populations or without proper oversight. Exploiting the disadvantaged can create a landscape of distrust in the scientific community. Researchers must approach these practices with sensitivity, avoiding any appearance of coercion or manipulation. This highlights the need for ethical review boards to evaluate research proposals and ensure compliance with ethical standards.
Consent Issues in Research
Consent issues in iPS research are integral to ethical considerations. The importance of obtaining informed consent cannot be overstated. Consent must not only be informed but also voluntary, meaning individuals should not feel pressured to provide their biological materials for research purposes. Consent forms must be clear, concise, and devoid of complex language, to ensure understanding.
Additionally, the storage and future use of donated samples present further consent challenges. Participants should be aware that their cells may be used for various research purposes beyond the initial study. They must have the option to opt-in or opt-out of such extended use. Researchers should be transparent about the future applications of iPS cells, which can include drug testing, disease modeling, and even genetic studies. This transparency is essential for maintaining trust between researchers and the public.
Moreover, there can be cases where consent becomes complicated, such as when research involves deceased individuals or samples from biobanks. At times, navigating these scenarios requires balancing respect for the deceased’s legacy and the rights of their families. Ethical frameworks must evolve to address these complexities and ensure that consent remains a priority across diverse contexts.
"Ethical awareness in iPS cell research is vital for fostering public trust and ensuring the responsible use of scientific advancements."
In summary, ethical considerations in iPS research, including the sourcing of somatic cells and consent issues, are paramount in shaping the future of regenerative medicine. Addressing these concerns helps to uphold the ethical standards necessary for progress in biomedicine.
Future Directions in iPS Research
The field of induced pluripotent stem (iPS) cell research is rapidly evolving, offering promising future directions that are crucial for both basic science and therapeutic applications. As advancements continue to emerge, it is essential to analyze these trends and their potential implications. Understanding the future of iPS cell research will guide scholars, researchers, and clinicians in harnessing the full capabilities of this technology.
Advances in Reprogramming Techniques
One critical aspect of future iPS research is the improvement of reprogramming techniques. Current methods, notably viral vector systems, can introduce complications such as genetic instability and tumorigenicity. Emerging techniques aim to address these issues. For instance, researchers are exploring the use of mRNA-based methods. These techniques allow for the temporary expression of reprogramming factors without altering the host genome. Additionally, the development of small molecules that can induce pluripotency is gaining traction. Such approaches would reduce potential risks associated with traditional viral methods, thereby promoting safer applications in regenerative medicine.
Furthermore, the efficiency of reprogramming also remains a focal point. Innovations in techniques such as direct reprogramming, which allows somatic cells to convert to specific cell types without passing through a pluripotent phase, are on the rise. This advancement could streamline cell replacement therapies, increasing the speed at which treatments can be developed for various conditions. As these techniques enhance efficiencies and safety profiles, they may increasingly facilitate clinical applications.
Integration of iPS Cells in Personalized Medicine
Another pivotal direction for iPS research involves the integration of iPS cells into personalized medicine. This approach offers a framework where therapies can be tailored to an individual's genetic background, improving their effectiveness and minimizing adverse effects. For instance, iPS cells derived from a patient’s own tissues can model diseases through patient-specific cell lines.
The utilization of iPS cells could enable more precise drug testing, allowing clinicians to gauge therapy responses before clinical interventions. In serious conditions like cancer or genetic disorders, this method could present significant benefits in determining the most effective treatment plans.
Moreover, iPS technology can contribute to the field of regenerative medicine by providing patient-specific cells for transplantation. These cells can potentially reduce the risk of rejection and optimize outcomes compared to traditional donor cell sources. However, these benefits come with challenges such as ensuring the proper differentiation of cells and scaling production for clinical use. Addressing these issues is critical for the successful implementation of iPS-derived therapies in personalized medicine.
In summary, the future of iPS cell research is bright, marked by ongoing advancements in reprogramming techniques and integration into personalized medicine. As these developments unfold, they promise to revolutionize therapeutic approaches, enhancing the efficacy of treatments tailored to individual patient needs.
Culmination
This article has presented comprehensive insights into the transformative nature of induced pluripotent stem (iPS) cell reprogramming. The significance of iPS technology cannot be understated. It not only provides a renewable source of pluripotent cells but also opens new avenues in regenerative medicine, modeling diseases, and drug development. By utilizing somatic cells, iPS reprogramming allows researchers to bypass many ethical dilemmas associated with embryonic stem cells, paving the way for innovative approaches in various fields.
Summary of Key Findings
The exploration of iPS cell reprogramming has highlighted several key points:
- Fundamental Mechanisms: The mechanisms underlying reprogramming, including transcription factors and epigenetic changes, are pivotal to understanding pluripotency.
- Innovative Applications: iPS cells offer promising applications in modeling diseases, enabling the development of targeted therapies, and facilitating drug discovery and toxicology studies.
- Challenges Encountered: Despite the progress, challenges such as genetic stability and technical limitations remain. Addressing these is crucial for the advancement of iPS technology.
- Ethical Insights: Ethical considerations are integral to the research landscape. Ensuring informed consent and defining the source of somatic cells are important for maintaining public trust.
Impact on Future Research
Looking ahead, the implications of iPS cell technology on future research are profound. Potential advancements include:
- Improved Reprogramming Techniques: Focused research on enhancing the efficiency and safety of reprogramming is essential. New methods may decrease the likelihood of tumorigenicity and cell line variability.
- Personalized Medicine Integration: As iPS technology continues to evolve, its integration into personalized medicine frameworks could revolutionize treatment protocols tailored to individual genetic profiles.
- Broader Applications in Various Fields: The adaptability of iPS cells could lead to wide-ranging applications not just in medicine, but also in toxicology testing, organ transplantation, and basic science studies.
The enduring impact of iPS cell reprogramming on biomedical research offers a lantern of hope for upcoming therapies and innovations, enhancing our ability to tackle complex health issues.