In-Depth Insights on Infrared Detectors
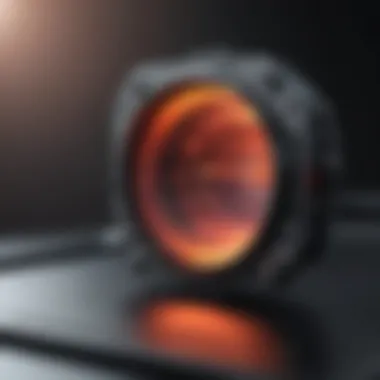
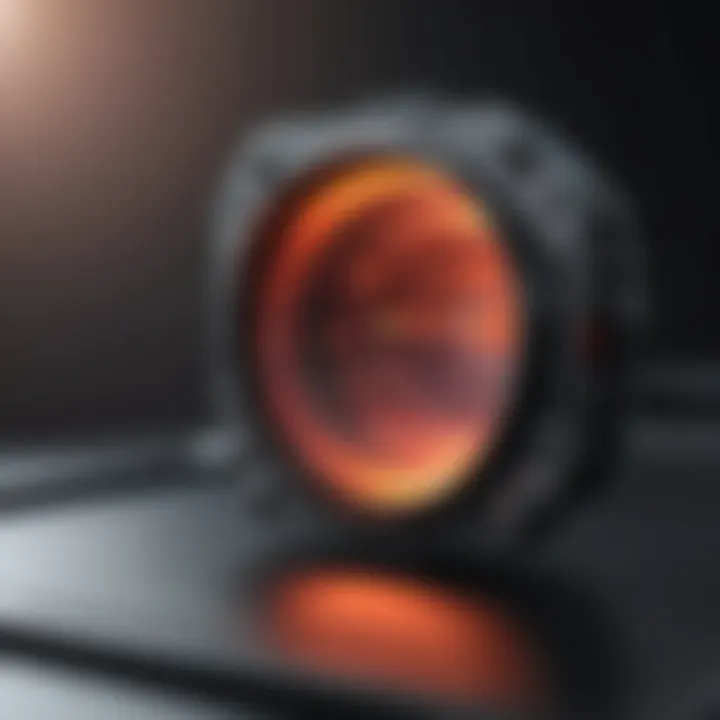
Intro
Infrared detectors are vital components in a range of applications, spanning various industries. They function by detecting infrared radiation, which is a type of electromagnetic radiation with wavelengths longer than visible light. These detectors play a crucial role in military technologies, environmental monitoring, medical applications, and industrial settings. Understanding the mechanisms, capabilities, and advancements in infrared detector technology can provide significant insights into their importance in modern society.
To structure this inquiry, we will first discuss the principles of operation underlying infrared detectors, followed by classification into different types. This will be enhanced by a detailed examination of applications, focusing on how infrared technology is utilized in diverse fields. Finally, recent innovations will be highlighted to reveal the ongoing evolution of these detectors. Each of these components is essential for grasping the full scope of infrared detection technology in contemporary use.
Prelims to Infrared Detectors
Infrared detectors play a crucial role in various technological applications today. Their importance can be seen in the military, medical, industrial, and environmental sectors. Understanding how these detectors operate and their historical context helps in appreciating their evolution and significance in todayโs world. The mechanics behind infrared detection enhance both security measures and medical diagnostics, making them indispensable tools in modern society.
Definition and Overview
Infrared detectors are devices that sense infrared radiation, which is not visible to the human eye but is emitted by warm objects. These detectors convert this radiation into an electrical signal, allowing for the measurement of temperature or the detection of objects based on their heat signatures. Generally, they are categorized into two main types: thermal detectors and photon detectors. Thermal detectors respond to changes in temperature, while photon detectors operate by interacting with the incoming photons directly.
One of the primary applications is in temperature monitoring. The ability to assess heat from objects can provide critical data in medical diagnostics, ensuring rapid and non-invasive assessments of patients. In the military framework, these detectors are crucial for surveillance and reconnaissance missions. Their sensitivity to heat enables detection of concealed objects, which can improve mission outcomes.
Historical Development
The trajectory of infrared detector technology can be mapped back to the mid-20th century. The early infrared detectors, developed from thermocouples and bolometers, emphasized basic thermal sensitivity. Bolometers, in particular, were significant for their ability to detect minimal changes in thermal radiation. Their performance was primarily limited by the ambient temperature and material properties.
Eventually, advancements in materials science and semiconductor technology spurred the development of more sophisticated detectors. The introduction of thermoelectric materials and semiconductor constructs, like InGaAs and HgCdTe, marked a significant leap forward in detection capabilities. These semiconductor-based detectors combined sensitivity with compact size, making them highly versatile and supporting broader applications beyond military use.
As industries sought improved precision in measurement, the shift towards integrating infrared detection in civilian sectors began gaining traction. Today, infrared detectors are ubiquitous, woven into various applications such as autonomous vehicles, smart home devices, and medical imaging technologies. The journey from basic thermal detection to advanced integrated systems illustrates the field's dynamic evolution and the ongoing quest for improved performance.
Physics of Infrared Detection
The physics of infrared detection serves as the foundation for understanding how these devices operate. Infrared detectors translate infrared radiation into electrical signals, allowing for various applications across multiple sectors. Understanding the basic principles, material properties, and quantum efficiency of infrared detectors provides insight into their performance and capabilities. These insights are crucial for the development and optimization of new technologies that leverage infrared sensing, ensuring that advancements in this field continue to meet the growing demands of industry and society.
Basic Principles
Infrared detectors function by sensing the thermal radiation emitted by objects. This radiation is a result of molecular vibrations and is dependent on the temperature of the object. The primary working principle hinges on the interaction of infrared photons with the detector material, leading to either a change in the temperature or excitation of electrons.
- Thermal Detection: In thermal detectors, temperature change is the key. The detector absorbs infrared radiation, which increases its temperature. This change is measured and converted into a signal.
- Photon Detection: Photon detectors rely on the conversion of incident photons into electrical signals. They work on semiconductor physics, where the absorption of a photon excites an electron, allowing current to flow.
- Examples include bolometers, which utilize resistive elements that change resistance based on temperature changes.
- Typical examples are photodiodes and photoconductors, which offer high sensitivity to infrared light.
This fundamental understanding of detection methods guides the design and application of infrared detectors across various fields.
Material Properties
The material used in infrared detectors plays a pivotal role in their performance. Different materials exhibit unique properties that affect sensitivity, response time, and detection range.
- Semiconductor Materials: Common semiconductors include indium gallium arsenide (InGaAs) and mercury cadmium telluride (HgCdTe). Each has distinct characteristics suited to specific applications, such as the ability to detect near-infrared wavelengths or mid-infrared wavelengths effectively.
- Thermal Conductivity: Materials with low thermal conductivity are often preferred in thermal detectors. Low conductivity ensures that detectable temperature changes are not dissipated too quickly, thereby increasing sensitivity.
- Band Gap Energies: The band gap energy of semiconductor materials is crucial for determining their response to infrared radiation. Materials with appropriate band gaps can effectively absorb and convert infrared photons into electrical signals.
These material properties are essential for engineers and researchers when designing detectors that meet specific application criteria.
Quantum Efficiency
Quantum efficiency is a critical metric in the performance of infrared detectors. It measures the effectiveness of a detector in converting incident photons into measurable electrical signals.
- Definition: Quantum efficiency ( QE) is defined as the ratio of the number of charge carriers generated to the number of incident photons.
- Impact on Sensitivity: Higher quantum efficiency translates to increased sensitivity, making it crucial for applications requiring precise measurements, such as medical diagnostics or environmental monitoring.
- Optimization: The optimization of quantum efficiency involves material engineering and device design. By enhancing the properties of detector materials, researchers can produce detectors with improved quantum efficiencies, making them more suitable for diverse applications.
To sum it up, the physics governing infrared detection involves a complex interplay of principles, material characteristics, and efficiency metrics. These elements are interrelated and impact the overall function and utility of infrared detectors in various sectors.
Types of Infrared Detectors
Understanding the different types of infrared detectors is vital in comprehending their applications and how they operate in various contexts. Each type has unique characteristics, advantages, and limitations, influencing their use in military, industrial, medical, and environmental settings. By categorizing infrared detectors into thermal, photon, and semiconductor types, one can appreciate their individual contributions to technology.
Thermal Detectors
Thermal detectors respond to changes in temperature caused by incident infrared radiation. This ability makes them indispensable in applications where thermal sensitivities are required. Within thermal detectors, bolometers and pyroelectric detectors are prevalent.
Bolometers
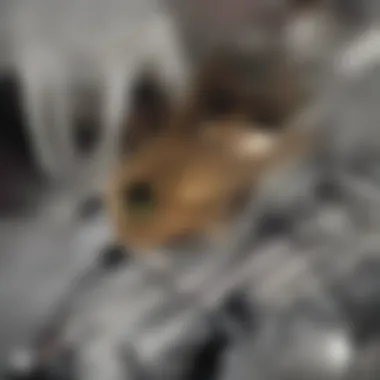
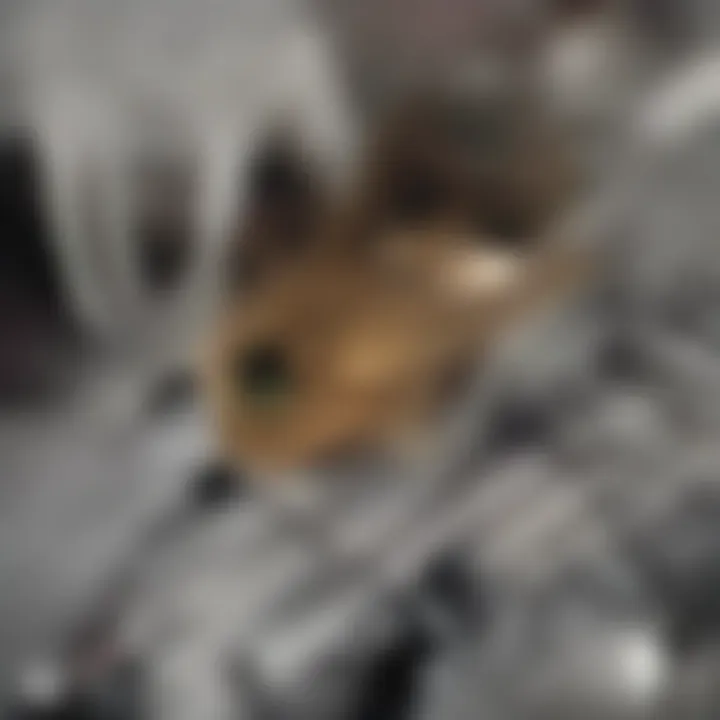
Bolometers measure the change in temperature of a material due to absorbed infrared radiation. Their key characteristic is high sensitivity, which allows them to detect even minute temperature shifts. This sensitivity makes bolometers a popular choice in astrophysics and sensitive imaging applications. The unique feature of bolometers is their ability to operate across a wide spectrum, making them versatile for different uses.
However, there are disadvantages. Bolometers typically require cooling to reach optimal performance, which can complicate their implementation in portable devices. This cooling requirement sometimes limits their application in settings where size and weight are critical considerations.
Pyroelectric Detectors
Pyroelectric detectors sense changes in temperature by utilizing materials that exhibit a spontaneous polarization change. Their key characteristic is the ability to operate at room temperature, making them more suitable for various practical applications. This aspect of pyroelectric detectors allows for easy integration into consumer electronics, such as motion detectors in security systems.
Nevertheless, these detectors can only measure dynamic temperature changes, not static ones. This limits their functionality in scenarios where a constant temperature infrared source is present, potentially restricting their range of applications.
Photon Detectors
Photon detectors operate by directly detecting photons of infrared light. They provide great precision and accuracy, making them valuable in scientific research and various commercial applications. This category includes photodiodes and photoconductors, each serving unique purposes.
Photodiodes
Photodiodes generate an electric current in response to incident infrared photons. Their essential feature is high speed and responsiveness. These properties make photodiodes an excellent choice for applications requiring fast response times, such as communication and signal processing systems. Photodiodes are also widely used in optical fiber technology and infrared spectroscopy.
A notable advantage of photodiodes is their ability to function without the need for cooling, which simplifies their integration into devices. Nonetheless, they can have limitations regarding sensitivity to low-intensity infrared signals, which may affect performance in specific applications.
Photoconductors
Photoconductors change their electrical conductivity when exposed to infrared radiation. This characteristic allows for their use in various applications, such as thermal imaging and night vision. They are particularly effective in environments where high sensitivity is not as critical.
The unique feature of photoconductors is their ability to operate over a broad spectral range, but they often suffer from slow response times compared to photodiodes. This makes them less suitable for applications where rapid signal processing is essential.
Semiconductor Detectors
Semiconductor detectors function based on semiconductor materials to detect infrared radiation. They are notable for their efficiency and compact size. Two prominent types in this category are InGaAs and HgCdTe detectors.
InGaAs Detectors
InGaAs detectors are sensitive to infrared radiation in the 0.9 to 2.6 micrometer range. Their key characteristic is the ability to operate under room temperature conditions while maintaining good efficiency. This feature makes them suitable for fiber-optic communications and remote sensing applications.
A significant advantage is their relatively low noise level, enhancing signal quality. However, InGaAs detectors can be sensitive to temperature variations, which may require careful consideration in certain environments.
HgCdTe Detectors
HgCdTe detectors cover a wide wavelength range, from near-infrared to long-wave infrared. Their key characteristic is tunability, which allows for adjustments to the active region's wavelength sensitivity by changing the composition. This flexibility makes HgCdTe detectors valuable in defense and industrial applications.
While HgCdTe detectors excel in various conditions, they often require cooling for optimal performance, necessitating additional components that could hinder their use in compact devices.
In summary, the landscape of infrared detectors is diverse, with thermal, photon, and semiconductor types designed for specific use cases. Each has advantages and disadvantages, which influence their levels of effectiveness in various applications.
Design Parameters
The design parameters of infrared detectors are crucial in determining their effectiveness and application in varying environments. These parameters define how detectors perform in terms of efficiency, speed, and reliability. Understanding design parameters helps in selecting the right type of infrared detector for specific tasks. It allows engineers and scientists to tailor the characteristics to meet specific needs, whether in military, medical, industrial, or environmental use.
Sensitivity
Sensitivity refers to the ability of an infrared detector to detect low levels of infrared light. It is a significant design parameter that impacts the performance of detectors in low light situations. High sensitivity indicates that the detector can identify minute changes in temperature or light, which is vital in applications such as thermal imaging in medical diagnostics and surveillance in military contexts. Factors contributing to sensitivity include the material properties of the detector and its operational environment. Sensitive infrared detectors often employ special materials or configurations that enhance their capability to pick up faint signals.
Response Time
Response time is the duration taken by an infrared detector to react to changes in infrared radiation. Fast response times are crucial in applications where rapid detection of temperature changes is necessary. For example, in industrial processes, detecting anomalies quickly can prevent safety hazards or equipment failures. Response times can vary significantly among different types of detectors. Thermal detectors generally exhibit slower response times, while photon detectors tend to respond much more quickly. Manufacturers may optimize design for speed without compromising sensitivity, balancing these factors based on intended applications.
Temperature Range
The temperature range defines the interval over which an infrared detector can operate effectively. This is largely influenced by the materials used in the detector and its construction. Some detectors function well at very low temperatures, while others might excel in high-temperature environments. For instance, InGaAs detectors can operate effectively at temperatures ranging from -40ยฐC to 60ยฐC. Selecting a detector that matches the operational temperature range of its application is vital for ensuring reliable performance and longevity.
Noise Characteristics
Noise characteristics play a central role in the performance of infrared detectors. Noise can interfere with the signal detected, diminishing the clarity and reliability of the readings. Understanding the sources of noiseโbe it environmental or intrinsic to the detector itselfโis critical. This is important in settings where precision is paramount, such as in medical diagnostics or environmental monitoring. Engineers take steps to minimize noise through specific design choices and careful material selection, allowing the detector to function as effectively as possible.
Applications of Infrared Detectors
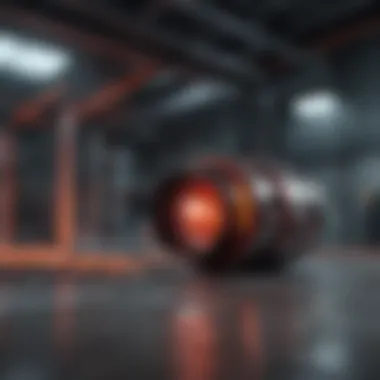
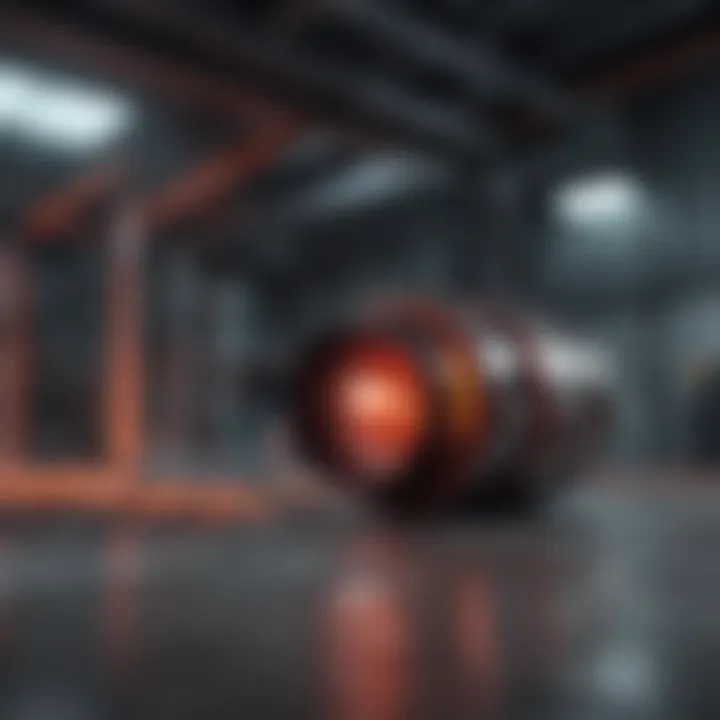
The applications of infrared detectors are vast and varied, impacting numerous fields from military operations to environmental monitoring. Their ability to detect thermal radiation makes them essential for various technologies. These detectors serve critical roles in surveillance, industrial processes, medical diagnostics, and research into climate change. Each application showcases specific benefits and considerations that highlight their significance in modern technology.
Military and Defense
Surveillance Systems
Surveillance systems are crucial in military settings. Infrared detectors enhance their effectiveness by allowing visibility in low-light conditions. The primary characteristic of these systems is their ability to operate day and night, making them reliable for securing borders and sensitive areas.
A unique feature of surveillance systems is their capability for real-time monitoring. This leads to quicker responses to potential threats. However, a challenge lies in the potential for false alarms driven by environmental factors, which can distract from genuine security risks.
Guided Missiles
Guided missiles significantly benefit from infrared detection technology. The essential aspect of guided missiles is their precision targeting, which is greatly enhanced by the ability to lock onto heat signatures of their targets. This characteristic makes them a suitable choice for modern warfare strategies.
These missiles often employ infrared detectors to distinguish between live targets and decoys, providing a tactical advantage. Yet, there are disadvantages, such as susceptibility to countermeasures that aim to obscure heat signatures.
Industrial Applications
Process Control
In industrial settings, infrared detectors play a key role in process control. They allow monitoring of temperature variations in manufacturing processes, which ensures efficiency and quality. The primary characteristic of process control systems is their capacity for continuous observation, identifying anomalies in real time.
This continuous monitoring can lead to improved product quality and reduced waste. Nonetheless, installation and maintenance costs can be significant, which may limit implementation for smaller enterprises.
Leak Detection
Leak detection is another vital application of infrared detectors. They are integral to identifying harmful leaks in various systems, including gas pipelines and chemical plants. A key characteristic is the high sensitivity that these detectors can achieve, enabling them to spot even minor leaks before they escalate into serious issues.
The unique feature of this application is its preventive capability, which is crucial for safety and environmental protection. On the downside, reliance on infrared detection also demands regular calibration and testing to ensure accuracy.
Medical Diagnostics
Thermal Imaging
Thermal imaging is a prominent application in the medical field. Infrared detectors allow for non-invasive observation of body temperature variations, aiding in diagnosing conditions such as infections and inflammation. One key characteristic is the ability to provide visual maps of temperature distribution on the body, facilitating quick assessments.
This non-invasive method is beneficial, reducing the need for invasive procedures. However, interpretation of thermal images can be subjective, leading to potential diagnostic errors.
Non-Invasive Procedures
Infrared detection technology is also used in various non-invasive procedures, such as vascular imaging. These procedures offer easy monitoring of blood flow and vascular health. A significant characteristic is the elimination of needles or other invasive tools, providing comfort to patients.
This non-invasive approach is beneficial in increasing patient acceptance of diagnostic procedures. However, some limitations exist, such as the resolution of imaging which can affect the detection of subtle anomalies.
Environmental Monitoring
Pollution Detection
Infrared detectors have become essential in pollution detection, allowing scientists and governments to monitor air quality and emissions. The critical aspect is their sensitivity to specific gases, which makes them effective for identifying harmful pollutants in urban environments.
The unique feature of using infrared technology for pollution detection is its capacity for real-time data collection, which aids in timely interventions. One challenge, however, is that external factors, like weather conditions, can influence the accuracy of readings.
Climate Research
Climate research also benefits from infrared detection technologies. These detectors can assess temperature changes over large areas, providing crucial data for understanding climate phenomena. A key characteristic of their use in this field is the extensive range of data they can collect across various environments.
The value of infrared detectors in climate research is their ability to yield insights into global warming and its impacts on ecosystems. Nonetheless, there are challenges related to data processing and interpretation, which require robust analytical tools.
Recent Advancements
Recent advancements in infrared detectors are significant not just for technological innovation but also for enhancing existing applications across various fields. These advancements often leverage new materials and integration with cutting-edge technologies. The impact can be seen in improved performance, greater efficiency, and the development of new use cases that were not viable before.
Nanotechnology in Infrared Detectors
Nanotechnology has become a game-changer in the field of infrared detectors. By manipulating materials at the nanoscale, researchers can enhance the sensitivity and response time of detectors. Notably, nanoparticles can create unique optical properties, which improve light absorption and quantum efficiency. This tailored design allows for more compact and efficient detectors, which can be critical in applications such as thermal imaging and spectroscopy.
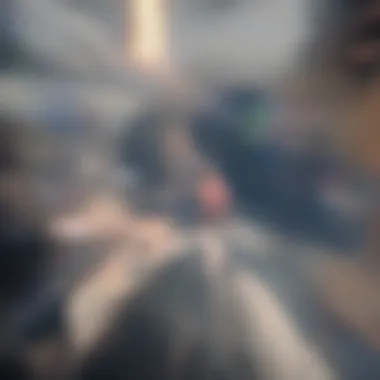
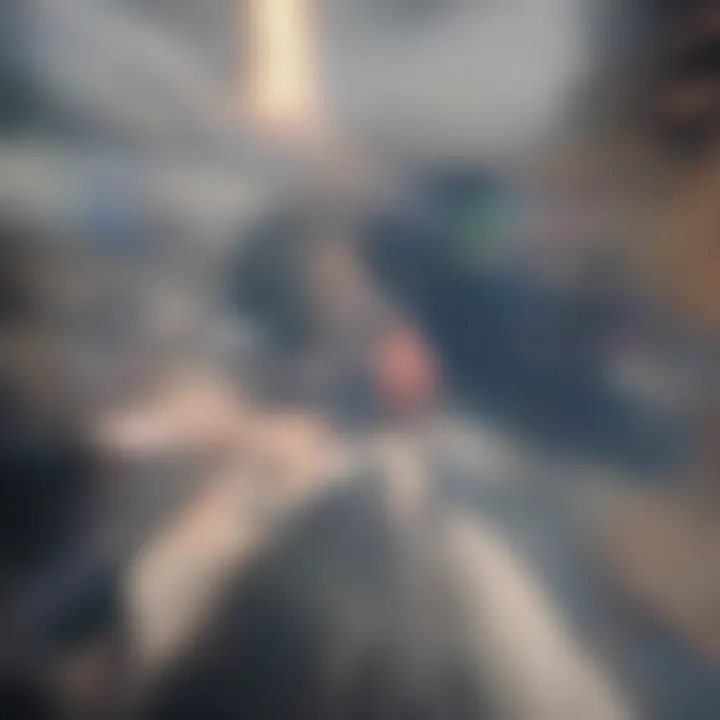
The use of nanotechnology also allows for innovations like nanowire and nanosheet detectors, which offer high performance in a small footprint. While there are challenges associated with scalability and reproducibility of nanomaterials, the benefits may outweigh these issues in the long run. High sensitivity at a lower cost could lead to broader adoption.
Integration with Other Technologies
Machine Learning
Machine learning plays a crucial role in enhancing the capabilities of infrared detectors. It can analyze vast datasets quickly. This capability allows for real-time processing of infrared signals, thus improving interpretation and response. One key characteristic of machine learning is its adaptability; it can improve model accuracy over time by learning from new data inputs. This means ongoing enhancement of detection algorithms, making them more efficient.
A unique feature of integrating machine learning is its ability to automate decision-making in applications. For instance, in surveillance systems, machine learning can discern between objects and classify potential threats, significantly reducing response times. However, challenges exist such as the need for large labeled datasets for training, which may limit some applications in specific fields.
Sensor Fusion
Sensor fusion combines data from multiple sensors to create a comprehensive view of a given situation. In infrared detection, this can enhance object detection and environmental analysis. The key characteristic here is its ability to provide contextual information, leading to better decision-making. Sensor fusion incorporates not just infrared data but also input from visible light cameras, LIDAR, and other sensors to achieve a holistic sense.
A unique advantage of sensor fusion is the redundancy it provides. If one sensor fails or provides inaccurate data, others can compensate to prevent failure of the overall system. However, integration complexity can pose challenges. The need for advanced algorithms to interpret data from diverse sources also requires significant computational power. Despite these challenges, its benefits in accuracy and reliability continue to drive interest in its adoption.
"Recent advancements in infrared detectors not only bolster existing technology but also pave the way for new opportunities in various sectors."
Challenges and Limitations
Understanding the challenges and limitations faced by infrared detectors is crucial for both future research and practical applications. These detectors, while highly useful across numerous sectors, also come with performance issues and manufacturing hurdles that can impact their effectiveness and adoption. Analyzing these obstacles helps to better appreciate their functionality and guides improvements for future enhancements.
Performance Limitations
Performance limitations in infrared detectors can stem from various factors, which directly influence their efficiency and reliability. One of the primary concerns is the sensitivity of the detectors. Sensitivity determines how effectively a detector can respond to low levels of infrared radiation. When the sensitivity is suboptimal, the detector might fail to capture crucial signals, rendering it less useful in sensitive applications like surveillance or medical diagnostics.
Another limitation is the response time. Infrared detectors need to react quickly to changing thermal environments or moving objects. When this response is slow, it restricts the functionality, especially in real-time applications. For instance, in guided missile systems, any delay in detecting and processing infrared signals can compromise the success of tracking targets.
Finally, noise characteristics significantly affect detector performance. Noise can be intrinsic or extrinsic, and high levels of noise can lead to false readings. This can result in erroneous conclusions in medical imaging or environmental monitoring, leading to a mistrust in detector capabilities. The overall performance thus hinges on overcoming these limitations.
Manufacturing Challenges
Manufacturing infrared detectors poses distinct challenges that can affect availability and cost. One major issue is the complexity of the materials involved. Semiconductor materials, like those used in Indium Gallium Arsenide (InGaAs) detectors, require precise fabrication techniques. Variances in material quality or composition can lead to inconsistencies in detector performance, necessitating rigorous quality assurance processes.
Another challenge is scaling production to meet increasing demand, especially in high-tech applications. This often demands advanced manufacturing technologies, which may not be widely accessible. High production costs can make it economically unfeasible to implement these detectors in lower-budget projects, despite their potential benefits.
Additionally, as technology advances, the need for compatibility with existing systems creates a barrier to innovation. Newer detectors may not always be easily integrated with older systems, leading to increased development time and costs. These manufacturing challenges can hinder the wider use of infrared detectors, despite their demonstrated advantages.
Future Directions
The future of infrared detectors is promising, influenced by rapid technological advancements and growing demands across multiple sectors. These detectors are essential in applications requiring sensitive detection and precise measurements, thus, the direction they take is crucial for future innovations. Understanding emerging technologies and potential applications in this field can shape both present and future systems, ensuring they meet the needs of society.
Emerging Technologies
Emerging technologies are greatly impacting the development of infrared detectors. One significant advancement is the integration of nanotechnology, which enhances the sensitivity and performance of these devices. By using nanomaterials, researchers are creating detectors that can operate at lower temperatures while maintaining high efficiency. This is vital for applications in remote sensing and military surveillance.
Another noteworthy advancement is the development of hybrid detectors which combine the features of both thermal and photon detectors. These hybrid systems promise improved responsiveness and functionality, enabling better performance in varying conditions.
- Advancing materials: New semiconductor materials like graphene are being explored for their unique properties in infrared detection.
- Optoelectronic integration: Integrating infrared detectors with photonic circuits can lead to miniaturized and more efficient devices.
Potential Applications
The potential applications for advanced infrared detectors are vast and varied, each with its unique challenges and opportunities. Some key areas include:
- Smart Cities: Infrared detectors can monitor air quality and temperature within urban environments, contributing to real-time environmental management.
- Healthcare: In medical diagnostics, they may lead to breakthroughs in non-invasive procedures by allowing precise temperature readings in thermal imaging technologies.
- Autonomous Vehicles: Integrating infrared detectors into self-driving systems can enhance night vision and obstacle detection, improving safety and operation in low-light conditions.
- Agriculture: They can be used for detecting plant health by monitoring thermal emissions, allowing farmers to optimize resources.
The integration of infrared detectors into various sectors can significantly enhance operational efficiency and safety, making continual upgrades in this field vital for future success.
Closure
The conclusion section serves as a crucial component of this article on infrared detectors, encapsulating the essential findings and insights discussed throughout. By summarizing key points, this section reinforces the significance of infrared detectors in various fields and highlights the ongoing technological evolution influencing their usage. The advancement in infrared detector technology impacts a wide array of applications, including military operations, medical diagnostics, and environmental monitoring.
The benefits of mastering the knowledge of infrared detectors extend beyond comprehension of their operation. Understanding their functionality is vital for researchers and professionals seeking to implement advanced solutions in their respective fields. This article reiterates the necessity for continuous research in this domain, which will foster innovation and enhance performance metrics associated with infrared technologies.
Summary of Key Points
- Infrared detectors play a critical role in numerous industries such as military, medical, and environmental.
- The diverse types of detectors include thermal, photon, and semiconductor varieties, each with unique characteristics.
- Design parameters such as sensitivity and response time are pivotal for optimal performance.
- Recent advancements, including nanotechnology and technology integration, have addressed existing challenges.
- Future trends show promising potential applications that can revolutionize current methodologies and practices.
Implications for Research and Technology
The implications of this study are significant. Infrared detectors present numerous opportunities and challenges for researchers. One implication is the increased demand for high-performance detectors. This demand pushes research toward optimizing detector efficiency and reducing noise, improving the overall reliability. Emerging technologies, like machine learning, can also enhance data analysis and sensor performance.
Moreover, the integration of detection systems with various platforms can lead to innovative applications, fostering a multidisciplinary approach to problem-solving. Overall, the research landscape surrounding infrared detectors is ripe for exploration, making it a valuable area of focus for academia and industry alike.