Exploring Innovations in Model Tweezers for Science
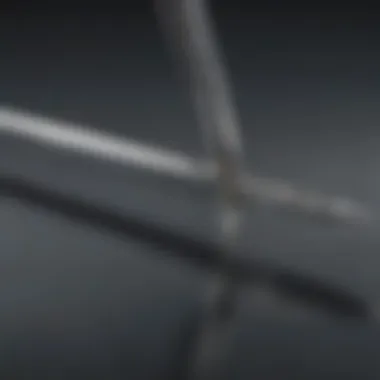
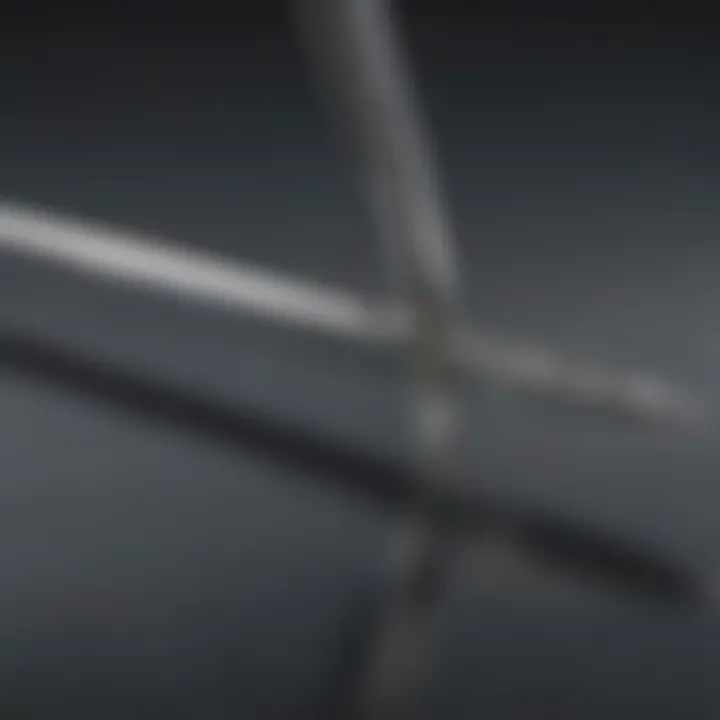
Intro
Model tweezers are indispensable tools in various scientific fields. Their design and functionality have evolved significantly over time, making them crucial for research and experimentation. This article will delve into the innovations behind different models of tweezers, their applications in laboratories, and how they facilitate the research process. By highlighting advancements, this overview aims to provide a clear understanding of the relevance of model tweezers today.
Research Methodology
Description of Research Design and Approach
This article employs a qualitative research design, blending literature review with expert interviews to gather insights on model tweezers' innovations and applications. The purpose is to create a comprehensive overview that captures current trends and future directions in this field. The approach focuses on analyzing existing studies, patents, and developments in the market to ensure a well-rounded perspective.
Materials and Methods Used in the Study
The following methods were employed in the study:
- Literature Review: Examination of academic journals, articles, and industry reports on model tweezers.
- Expert Interviews: Engaging with professionals and researchers who utilize model tweezers in their work.
- Case Studies: Analyzing specific instances where model tweezers contributed to significant discoveries or advancements.
Innovations in Model Tweezers
The advancements in model tweezers stem from a need for precision and efficiency in scientific research. Modern materials such as high-grade stainless steel and carbon fiber have enhanced durability and precision in design. Additionally, technological innovations like digital tweezers, which allow for electronic manipulation of materials, are emerging as game-changers.
Applications in Laboratory Settings
Model tweezers serve multiple purposes in laboratory environments, including:
- Handling Delicate Samples: Essential for manipulating fragile biological specimens.
- Assembly of Miniature Components: Used extensively in electronics and microfabrication.
- Precision Measurements: Facilitate accurate placement in experimental setups.
Using model tweezers can greatly enhance the accuracy of experiments. Their design often includes features like ergonomic handles and fine tips, contributing to better control and reduced strain on the user.
"Innovative models of tweezers not only improve efficiency but also expand the possibilities of what researchers can achieve in their work."
Future Trajectory
As research continues to evolve, the future of model tweezers looks promising. The integration of smart technologies and materials is likely to open new avenues for applications. Researchers may develop tweezers that not only assist with physical manipulation but also include sensors to measure properties during handling.
Epilogue
This overview highlights the significant role of model tweezers in scientific research. Their innovations and applications demonstrate a clear need for these tools in various fields. Moving forward, staying abreast of new technologies will be essential for researchers looking to maximize the effectiveness of their experiments.
Prelims to Model Tweezers
The study of model tweezers is essential in understanding the broader context of scientific equipment and their transformative impacts. These tools, integral to numerous branches of science, facilitate a range of functionalities from material handling to intricate experiments at the molecular level. Their importance cannot be overstated, due to their ability to deliver precision and control in various applications.
Model tweezers differentiate themselves through various designs, materials, and technological adaptations. By thoroughly examining these elements, we can uncover not only their practical uses but also the innovations driving their development. This article seeks to provide a comprehensive overview of model tweezers, their historical evolution, and their role in advancing scientific research.
In the realms of research, education, and professional practices, a solid understanding of model tweezers enhances operational efficiency. By recognizing their diverse functionalities, professionals can make informed decisions when selecting suitable types for specific applications.
Definition of Model Tweezers
Model tweezers refer to tools designed for precision gripping, manipulation, and positioning of small objects and components. These tools come in various designs and can be categorized based on their mechanism and functionality.
The term encompasses both traditional designs, used primarily in manual applications, and advanced models featuring automated technologies. The core objective remains constant: providing researchers with the ability to manipulate materials or samples with high accuracy.
Historical Perspective
The development of tweezers has a long history, tracing back to early civilizations. Initially, they were simple hand-held tools made from available materials, primarily used for beauty and medical purposes. As science progressed, particularly during the Renaissance, the demand for more precise instruments led to the refinement of tweezers.
Significant innovations arose in the late 19th and early 20th centuries with the advent of specialized tweezers for scientific use. During this period, researchers recognized the need for tools capable of handling increasingly delicate specimens, laying the foundation for the sophisticated models we see today. The 21st century has witnessed rapid advancements in tweezers technology, focusing on enhancing functionality through electric and digital innovations. Today, model tweezers are ubiquitous in laboratories and research facilities, supporting a variety of scientific disciplines from biology to physics.
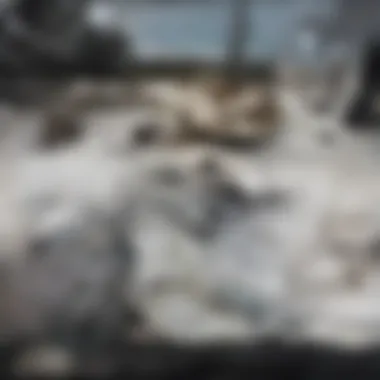
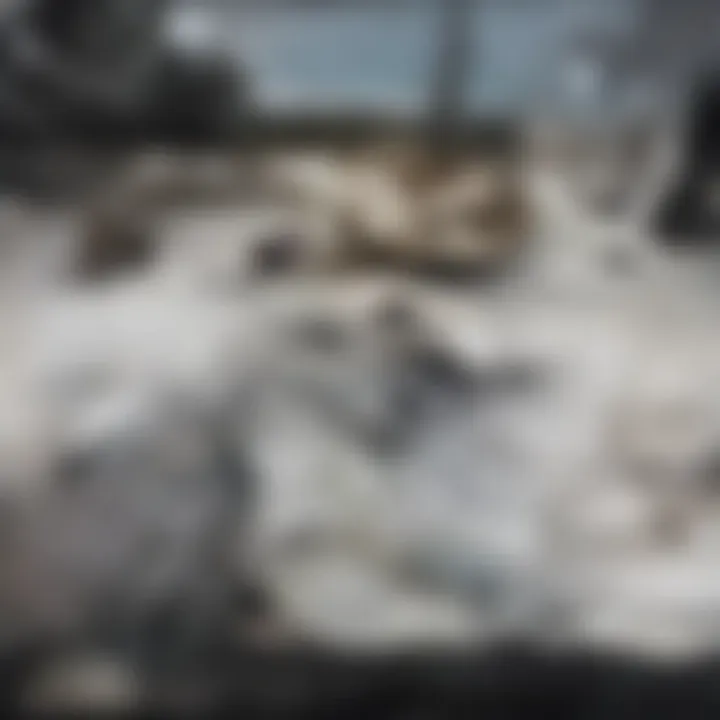
Types of Model Tweezers
The study of model tweezers reveals significant advancements in precision and control. Different types of tweezers cater to diverse applications and offer distinct advantages. Understanding the nuances of each type is crucial for researchers and practitioners. Identifying the right model can enhance the efficiency of tasks and improve the quality of outcomes in scientific experiments. Each type optimizes the handling of specific materials or specimens in unique ways, thereby broadening the scope of research possible with these tools.
Traditional Tweezers
Traditional tweezers are often the first tools introduced for manual operations in various fields. They are typically crafted from metals like stainless steel, offering durability and resistance to corrosion. These tweezers are designed with a simple, yet effective grasping mechanism.
The tips of traditional tweezers can vary in shape, such as pointed, flat, or serrated. This diversity allows for versatility in their use. In the lab environment, traditional tweezers are advantageous for handling small objects, particularly in delicate procedures.
Some key benefits of traditional tweezers include:
- Cost-effectiveness: Compared to more advanced types, traditional tweezers are generally less expensive.
- Ease of Use: Minimal training is required to use these tools efficiently.
- Availability: They are widely available in various shapes and sizes.
However, traditional tweezers have limitations in precision. The manual nature of handling makes them less suitable for tasks requiring high levels of accuracy.
Electric Tweezers
Electric tweezers represent a significant evolution in technology, offering enhanced control and automation. These tools use electric motors to facilitate precise movements and adjust gripping pressure, allowing for a more delicate handling of materials. In various scientific applications, electric tweezers are invaluable for tasks like micro-manipulation and electronic component assembly.
The advantages of electric tweezers include:
- Precision Control: They allow for fine adjustments, making it easier to grasp and position small objects.
- Reduced Fatigue: Automated grasping reduces the strain on hands during prolonged use.
- Customization: Many models offer programmable settings that can cater to specific tasks or user preferences.
Nevertheless, electric tweezers also introduce some challenges. They typically require a power source and can be more complex to operate than traditional models. Understanding the specifications of different electric tweezers can help in selecting the right tool.
Nanomanipulation Tweezers
Nanomanipulation tweezers are specialized instruments designed for manipulating materials at the nanoscale. These tweezers are often controlled through advanced robotics or optical traps, allowing for precision that is unattainable with other types. They play a crucial role in fields like nanotechnology and molecular biology.
This type of tweezers can facilitate several advanced applications, such as:
- Cellular Manipulation: Researchers can precisely position and move cellular components during biological studies.
- Material Testing: Testing the properties of materials at a nanoscale helps in developing new technologies.
- Drug Delivery: Nanomanipulation tweezers assist in targeting specific cells for drug application, improving treatment efficacy.
While nanomanipulation tweezers are groundbreaking, they do require a high level of expertise and specific conditions for operation. This limits their application to specialized research settings. The future for these tweezers looks bright with ongoing advancements in technology, paving the way for broader use in scientific research.
"The evolution of tweezers reflects not only technological advancements but also the need for precision in modern scientific inquiry."
In summary, the types of model tweezers offer unique advantages and specific applications. Understanding these varieties is essential for researchers looking to enhance their experimental accuracy and efficiency.
Design and Material Considerations
The design and material of model tweezers play crucial roles in their functionality and effectiveness in various scientific applications. The right design enhances the ease of use, precision, and control, while the choice of materials substantially impacts durability and performance. Understanding these elements is essential, especially for students, researchers, and professionals who rely on tweezers in their work. A comprehensive evaluation of the design considerations and materials used can yield significant benefits in productivity and efficiency.
Ergonomic Design Features
Ergonomic design features in model tweezers greatly influence their usability. Researchers often require prolonged use of these instruments, making comfort a priority. Ergonomics refers to the science of designing tools that fit the user. Features such as shaped grips or textured surfaces minimize hand strain and maximize control.
Some important ergonomic elements include:
- Grip Shape: The curvature and contour of the tweezers can provide a more natural fit to the fingers, ensuring less fatigue during extensive use.
- Weight Distribution: Balanced weight helps in reducing unnecessary strain on the wrist and fingers, enabling finer movements and better precision.
- Material Texture: Textured handles can prevent slippage, thus improving grip and control, especially in delicate procedures.
Ergonomic designs lead to less discomfort and more efficient use of tweezers. As a result, researchers are able to focus on their tasks without being hindered by tool fatigue, which can greatly optimize workflow and accuracy in experiments.
Materials Used in Construction
The materials chosen for the construction of model tweezers are equally important. They determine not only the longevity of the tools but also their adaptability to various applications. Common materials include stainless steel, plastic, and titanium, each with its own set of advantages.
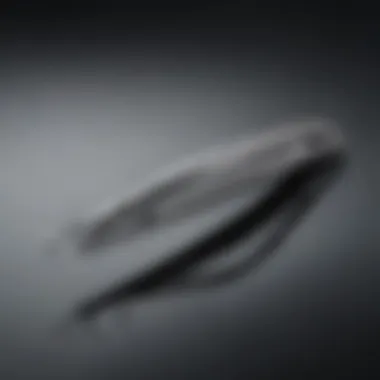
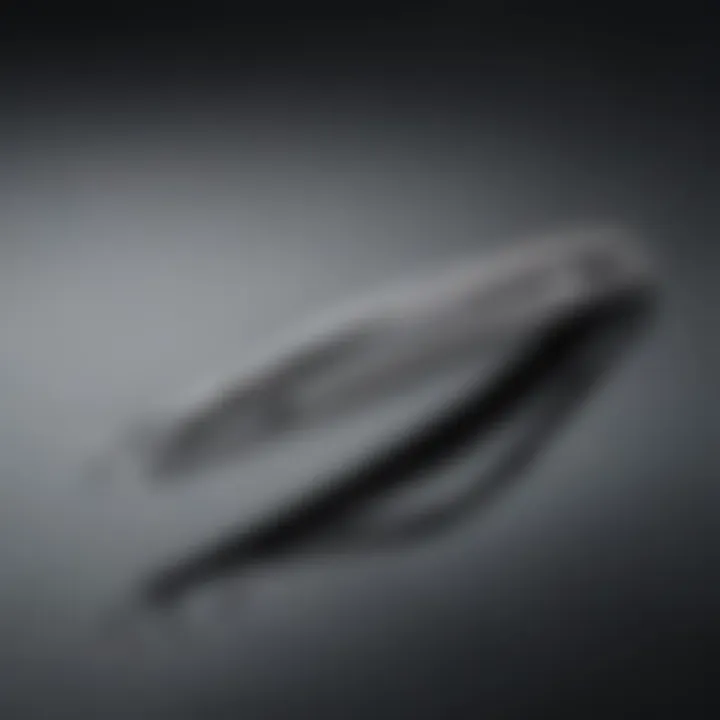
- Stainless Steel: This is a popular choice due to its corrosion resistance and durability. Stainless steel tweezers can withstand harsh laboratory conditions, making them ideal for fieldwork.
- Plastic: Often used for less demanding applications, plastic tweezers are lightweight and resistant to certain chemicals. However, their lack of strength can limit their usage.
- Titanium: Known for its high strength-to-weight ratio, titanium is used in specialized tweezers. Its non-reactive nature makes it suitable for applications involving sensitive materials.
When selecting tweezers, understanding the properties of these materials guides users in making informed choices. Each application may call for different requirements, and knowing the characteristics of available materials helps in optimizing performance.
"The choice of materials can substantially impact the lifespan and reliability of model tweezers in various scientific disciplines."
In summary, the design and materials of model tweezers are integral components that significantly influence their functionality and effectiveness. Considering ergonomic features alongside material properties allows researchers to select the most suited tools for their specific tasks. This understanding not only enhances usability but also contributes to overall research efficiency.
Functional Applications in Scientific Research
The functional applications of model tweezers in scientific research represent a pivotal element that underscores the extensive utility of these instruments. Their area of usage spans several key scientific disciplines, facilitating intricate manipulations that enhance experimental outcomes. By employing various types of tweezers, researchers can explore and manipulate samples at micro and nano scales, leading to innovations and advancements in their respective fields.
Use in Molecular Biology
In molecular biology, tweezers serve as essential tools for dissecting molecular interactions and managing delicate biological constructs. For instance, single-molecule manipulation techniques have been developed using optical tweezers, allowing scientists to observe how enzymes interact with DNA or RNA. Such observations provide valuable insights into fundamental biological processes like transcription and replication.
Tweezers enable researchers to apply controlled forces that can unfold or stretch macromolecules, thus enhancing understanding of their structural properties. This is particularly beneficial during experiments that require precise measurement of molecular dynamics.
Additionally, tweezing techniques have become invaluable in protein folding studies, helping to unravel the complexities of how proteins attain their functional forms. The advancements in this area have far-reaching implications for drug design and therapeutic interventions.
Role in Physics Experiments
In the domain of physics, tweezers are employed to manipulate small particles with great precision. Researchers utilize optical tweezers, which leverage laser beams, to trap and control particles ranging from atoms to small biological entities. This controllable setting allows for experiments that probe fundamental physical principles and particle dynamics under varying conditions.
Tweezers are instrumental in studying phenomena like Brownian motion and colloidal interactions. Physicists can observe how particles behave in response to external forces, leading to a deeper understanding of collective phenomena in various materials. Experimental setups involving tweezers allow researchers without the necessity of complicated machinery, thus simplifying some experimental processes significantly.
Applications in Material Science
Material science has also greatly benefited from the use of model tweezers. They assist scientists in assembling nanostructures and in the manipulation of materials on a nanoscale. By applying tweezing methods, researchers can explore the properties of new materials as they interact under stress and strain.
Microtweezers, for example, are used to position particles in the fabrication of nanoscale devices. These devices often have applications in electronics and photonics. The precision that tweezers afford enables the synthesis of materials with specific and desired properties.
Furthermore, these applications can lead to breakthroughs in developing smart materials that can respond dynamically to environmental changes, which opens a realm of possibilities in product design and engineering.
"Model tweezers are a bridge to understanding complex interactions within biological systems, physical principles, and material properties."
Precision and Control Mechanisms
Precision and control mechanisms stand at the forefront of effective application of model tweezers in scientific research. The ability to manipulate microscopic objects reliably and accurately is essential across various domains, ranging from molecular biology to materials science. These mechanisms enhance the usability of tweezers and ensure they can be effectively integrated into complex experimental setups, where even minor deviations can lead to significant errors.
Calibration Techniques
Calibration techniques are fundamental for establishing the accuracy of tweezers. Without effective calibration, the measurements and manipulations performed may not reflect the desired outcomes. Calibration involves adjusting the device to ensure that it responds correctly to known inputs. This process typically includes the following elements:
- Standardization: Utilizing known reference points helps in aligning the tweezersโ operation with established measurements.
- Regular Testing: Continuous testing against calibration standards is necessary to maintain precision over time.
- Documentation: Keeping detailed records of calibration data ensures that any abnormalities can be traced back and addressed effectively.
These techniques enhance the userโs confidence in the tweezers and allow for sophisticated adjustments that aim for utmost control.
Feedback Systems for Enhanced Control
Incorporating feedback systems is crucial for elevating the function of model tweezers. Feedback allows for real-time adjustments based on the device's performance relative to the expected standards. The main components of feedback systems include:
- Sensors: They provide data about the current state of the tweezers, measuring forces and positions with high precision.
- Control Algorithms: These algorithms process sensor data and make real-time adjustments to the tweezers' actions, ensuring optimal performance while handling delicate specimens.
- User Interface: A well-designed user interface can simplify the interaction with the feedback systems, allowing users to easily monitor and adjust settings as required.
The integration of advanced feedback systems has transformed how researchers interact with model tweezers, providing unprecedented levels of accuracy and control that were previously unattainable.
Challenges in Tweezers Technology
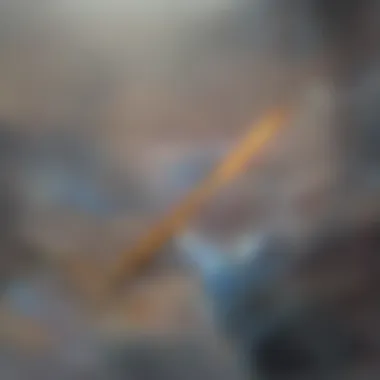
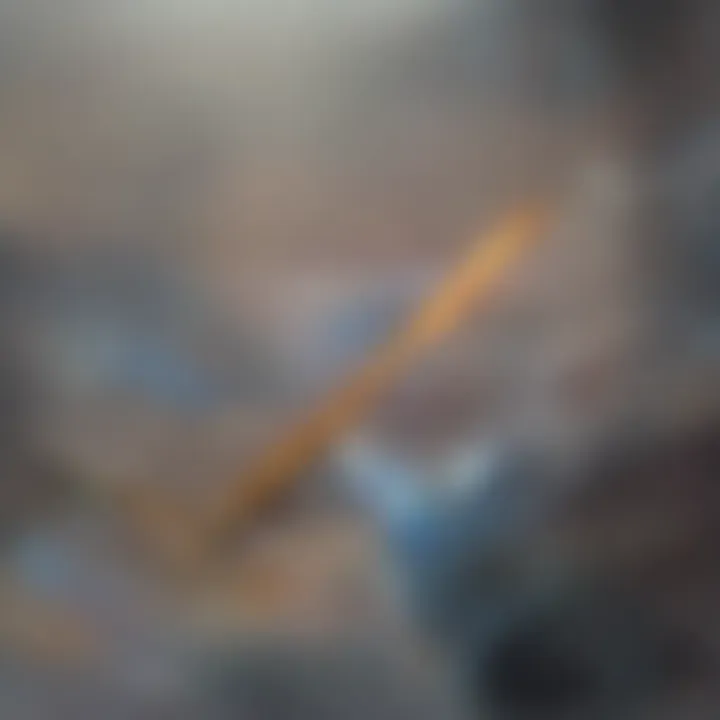
The field of tweezers technology is advancing rapidly, but several challenges hinder its progress. Understanding these challenges is essential for researchers, engineers, and educators. Addressing these issues can lead to improvements in design and functionality, ultimately enhancing the efficacy of scientific research.
Limitations of Current Models
Current models of tweezers, whether traditional or innovative, face several inherent limitations. These limitations can negatively affect their precision and control during experiments. Some key limitations include:
- Material Constraints: Many tweezers are made from materials that may not withstand extreme environmental conditions. Factors such as temperature fluctuations can affect their performance.
- Size Limitations: As scientific research increasingly delves into nanoscale applications, many standard tweezers struggle to meet the size specifications required. This results in a need for specialized models, which may not be widely available or may be underdeveloped.
- User Training: Even advanced tweezers may require significant skill to use correctly. Insufficient training can lead to errors, which, in turn, can compromise experiment results.
- Force Control: Too much or too little force can lead to material damage or failure in manipulation tasks. There is a need for more precise control mechanisms in the design of new models.
Impact of Environmental Factors
Environmental factors play a crucial role in the effective application of tweezers technology. Understanding these influences helps in optimizing their use. Key environmental considerations include:
- Temperature and Humidity: Variations in temperature and humidity can affect the mechanical properties of materials. Tweezers not designed for such conditions may lose effectiveness.
- Electromagnetic Interference: In some scientific applications, electromagnetic fields can disrupt precision tasks. This can be especially relevant for electric tweezers and nanomanipulation tools.
- Contamination: Laboratory environments often have dust, chemicals, or other contaminants. Such materials can affect the integrity of the tweezers and the samples being handled.
"Environmental factors can compromise the results of experiments, so understanding these elements is paramount for success."
In summary, while tweezers technology continues to evolve, limitations of current models and the impact of environmental factors present challenges that must be addressed. Increased awareness and research into these areas can ensure that model tweezers remain a vital tool in scientific exploration.
The Future of Model Tweezers
The evolution of model tweezers represents a significant chapter in the narrative of scientific research tools. As disciplines evolve, so too must the instruments that facilitate inquiry and experimentation. Model tweezers will play an increasingly pivotal role in various fields, ranging from molecular biology to nanotechnology. The focus on precision, control, and efficiency in scientific endeavors suggests that advancements in tweezers technology will continue to reshape research methodologies.
Innovations on the Horizon
Several innovations are on the brink of transforming the functionality and application of model tweezers. One key area of innovation is the integration of advanced materials. New composites that offer greater strength-to-weight ratios can enhance the sensitivity and control of tweezers, allowing researchers to manipulate smaller specimens with increased precision.
In addition, advancements in automation and robotic integration are anticipated. Smart tweezers, equipped with sensors and programmable controls, could enable automated manipulation of materials at the microscopic level. This technology may allow researchers to carry out repetitive tasks more efficiently, minimizing human error and increasing throughput.
Moreover, the incorporation of artificial intelligence may revolutionize how tweezers are used in lab settings. By analyzing real-time data and adjusting actions based on environmental feedback, AI-driven tweezers can optimize every manipulation process. Such capabilities will be invaluable in research where variability and precision are crucial.
Potential Impact on Scientific Disciplines
The ramifications of these innovations will be profound across several scientific disciplines. In molecular biology, for instance, enhanced tweezers could greatly improve the manipulation of DNA strands or proteins, thus facilitating more detailed studies of genetic processes and biochemical interactions.
Similarly, in material science, the ability to handle nanoscale materials more effectively could open new avenues for development. This includes the creation of advanced materials with tailored properties, which could influence both academic research and industrial applications.
In physics, enhanced model tweezers may enable experiments that require meticulous control of quantum-level products. This could lead to significant breakthroughs in our understanding of fundamental particles and forces, pushing the boundaries of modern physics.
"Advancements in model tweezers are not just incremental; they hold the potential to catalyze a paradigm shift in scientific research across multiple fields."
In summary, the future of model tweezers promises a convergence of technology and application that will expand their functional landscape. As these innovations take hold, they will provide researchers with tools that augment existing methodologies while opening the door to new avenues of discovery.
Culmination
In the realm of scientific research, model tweezers are much more than mere tools. They serve as the bridge between theoretical concepts and practical applications. Understanding the intricacies of these devices can enlighten users about their optimal applications in a variety of fields, from molecular biology to material science. The conclusion of this article underlines several key elements of model tweezers.
Summation of Key Points
Model tweezers are versatile instruments that have evolved significantly over time.
- Definition and Types: The basic types, including traditional, electric, and nanomanipulation tweezers, have distinct uses and advantages that cater to specific research needs.
- Design Considerations: Ergonomic designs and material choices are crucial for user comfort and durability.
- Functionality: From calibration techniques to enhanced control systems, these tools deploy cutting-edge technology that improves precision.
- Applications and Innovations: Their application in various scientific disciplines continues to expand, with ongoing innovations promising even greater advancements.
These points reflect an integrated view of how model tweezers function and their importance across various scientific landscapes.
Call for Future Research
As the field of research progresses, the call for future studies on model tweezers becomes more evident. Experts should focus on several areas:
- Interdisciplinary Applications: Investigation into how different scientific fields can leverage model tweezers for their unique problems.
- Technological Advances: Exploration of how artificial intelligence and machine learning can optimize tweezer functionalities.
- Sustainability: Research into eco-friendly materials and manufacturing processes for model tweezers.
"The future of model tweezers lies in innovation that enhances their abilities to tackle evolving scientific challenges."
Advancing this area not only enriches our understanding but also contributes significantly to scientific progress.