Exploring Modern Techniques in Analytical Chemistry
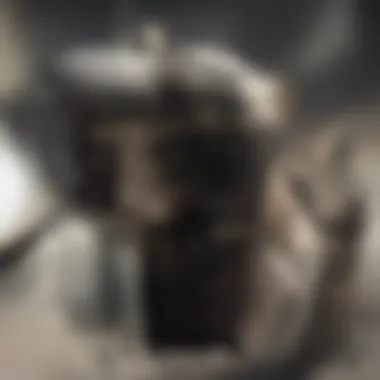
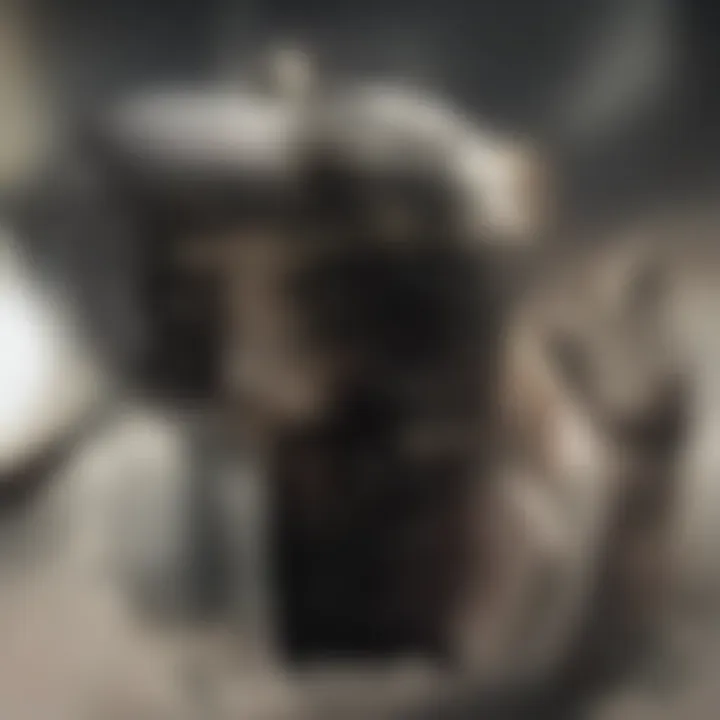
Intro
Analytical chemistry is a dynamic field that intersects with various scientific disciplines. It focuses on the techniques and methods for analyzing substances, determining their composition, and understanding their properties. As technology advances, so does the toolkit available to chemists. This article aims to explore modern methodologies, applications, and the various challenges faced in analytical chemistry today.
By examining fundamental principles and sophisticated instrumentation, we will illuminate the importance of analytical techniques in both research and industrial applications. Furthermore, case studies will offer concrete examples of how analytical chemistry informs decision-making processes across different sectors. As a result, readers can gain a deeper appreciation of the critical role this discipline plays in advancing scientific knowledge and industry practices.
Research Methodology
Description of Research Design and Approach
To understand the contemporary landscape of analytical chemistry, a comprehensive review of existing literature was conducted. This approach examined various studies to highlight the evolution of techniques and their practical applications. Through this literature review, the article contextualizes current methodologies within a historical framework, enriching our understanding of progress in the field.
In addition to literature analysis, interviews with seasoned professionals were carried out. These interviews provided insight into the practical challenges researchers face and the innovative solutions being developed.
Materials and Methods Used in the Study
The studies reviewed utilized a variety of instrumental techniques such as:
- Chromatography: Including gas chromatography (GC) and liquid chromatography (LC).
- Spectroscopy: Covering mass spectrometry (MS), nuclear magnetic resonance (NMR), and UV-Vis spectroscopy.
- Electrochemical Methods: Analyzing chemical species through their electrical properties.
Each method was chosen for its specific strengths in analyzing different types of substances, from complex biological samples to industrial chemicals. The integration of these techniques illustrates a multidisciplinary approach necessary for tackling modern analytical challenges.
Discussion and Interpretation
Interpretation of Results in the Context of Existing Literature
The results gathered from various studies indicate significant advancements in the precision and accuracy of analytical techniques. For instance, developments in mass spectrometry have enhanced detection limits, allowing for the analysis of trace components in intricate mixtures. According to recent findings, these advancements help address challenges such as environmental monitoring and food safety inspections.
Implications for Future Research or Practical Applications
Looking into the future, analytical chemistry is poised to respond to emerging global challenges. As the demand for rapid testing increases—particularly in areas like pharmaceuticals and environmental safety—research must continue to innovate. Enhanced automation and artificial intelligence may play roles in streamlining analytical processes.
"The innovative use of analytical chemistry is not just about observing but predicting outcomes that impact our daily lives."
Moreover, aligning analytical advancements with sustainability efforts will require collaboration across industries. Understanding the implications of these methods on public health and safety remains a central concern for future research and application.
Preamble to Analytical Chemistry
Analytical chemistry is a branch of chemistry focused on the qualitative and quantitative assessment of chemical substances. It plays a critical role in various fields, including pharmaceuticals, environmental science, and food safety. Understanding the principles behind it enables professionals and researchers to apply these techniques effectively in their work, which can lead to significant advancements in various domains.
Definition and Scope
Analytical chemistry involves systematic approaches to investigate the composition of materials. It seeks to answer questions such as what substances are present in a sample and in what amounts. The scope of analytical chemistry covers a range of methods, including both instrumental and non-instrumental techniques. Instrumental methods rely on advanced tools like chromatography and spectroscopy, while non-instrumental methods may include titrimetry. The field is inherently interdisciplinary, intersecting with biology, physics, and materials science. Professionals employ these methods to enhance product safety, ensure regulatory compliance, and drive research.
Historical Development
The roots of analytical chemistry trace back to ancient practices. Initially, techniques were rudimentary and closely tied to alchemical traditions. Over time, significant milestones marked the evolution of the field. The 19th century witnessed the emergence of quantitative analysis methods, particularly via volumetric analysis. In the 20th century, technological advancements led to the development of sophisticated instruments, enabling precise measurements and complex analyses.
The introduction of gas chromatography by Archer J. P. Brown in the 1950s exemplifies a pivotal moment in analytical chemistry, expanding the possibilities for separating and identifying chemical compounds. Further advancements included the advent of mass spectrometry, enhancing the capability for molecular analysis. Today, analytical chemistry continues to evolve, driven by advancements in technology and an increasing demand for accurate measurements in various industries.
Fundamental Principles of Analytical Chemistry
The field of analytical chemistry is grounded in specific principles that guide the processes of examination and quantification of substances. Understanding these fundamental principles is crucial for students, researchers, educators, and professionals working in various scientific fields. Analytical chemistry not only provides a framework for understanding chemical processes but also serves as a bridge between theoretical knowledge and practical applications. The principles discussed here—such as quantitative versus qualitative analysis and the significance of accuracy and precision—are foundational in achieving reliable and valid results in any analytical endeavor.
Quantitative vs Qualitative Analysis
Quantitative and qualitative analyses represent two fundamental aspects of analytical chemistry. Quantitative analysis focuses on determining how much of a particular substance is present in a sample. This can involve measurements of mass, concentration, or volume. Techniques such as gravimetric analysis or titrations are commonly used for quantitative determinations. This approach is particularly important in contexts where precise measurements are crucial, such as in pharmaceuticals, where drug dosages must be accurately controlled to ensure safety and efficacy.
On the opposite end, qualitative analysis aims to identify the components present in a sample. It does not focus on how much of a substance is there, but rather on the nature of the components. Methods including chromatography and spectroscopy can be used for qualitative assessments. Understanding the components of a mixture can inform further investigations and lead to more targeted quantitative analyses.
"The distinction between qualitative and quantitative analysis is fundamental in guiding scientists toward the appropriate methodology based on the objectives of their work.”
The balance of qualitative and quantitative analysis fosters a comprehensive understanding of samples. Therefore, choosing the correct approach is vital depending on the research question posed or the problem that needs solving. Both forms of analysis complement each other and are often used in tandem in various applications, from environmental monitoring to forensic science.
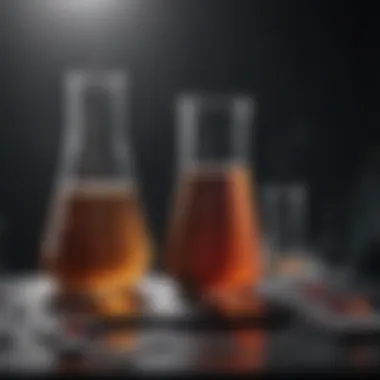
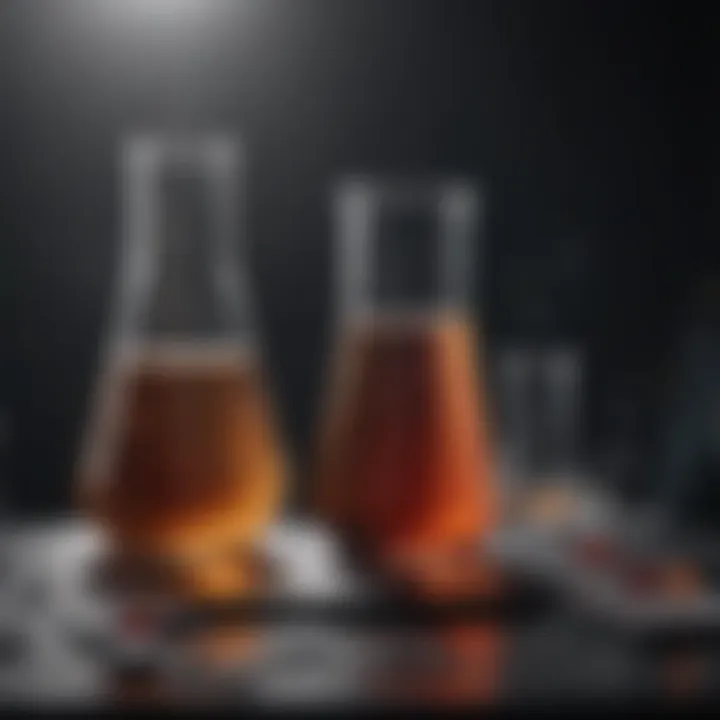
Accuracy and Precision
Accuracy and precision are cornerstones of any analytical process. Accuracy refers to how close a measured value is to the actual or true value. It indicates the correctness of an analysis. A highly accurate analytical method yields results that reflect the true quantity or identity of the substance being measured.
In contrast, precision refers to the repeatability of measurements—how consistently a method produces the same results under the same conditions. A precise method will give similar results, even if they are not necessarily close to the true value. High precision does not guarantee accuracy, but achieving both is desirable for any analytical process.
To improve accuracy, it is essential to calibrate instruments, standardize methods, and apply corrections for systematic errors. Precision can be improved through proper technique, such as ensuring that samples are homogeneously mixed and that equipment is properly maintained.
In summary, while accuracy ensures that results are correct, precision ensures that they are reliable. The interplay between these two factors is vital for achieving trustworthy results in analytical chemistry, underscoring the importance of careful method development and validation.
Common Analytical Techniques
Analytical techniques are essential. They play a crucial role in evaluating chemical compositions. These methods allow chemists to separate, identify, and quantify substances in samples. The effectiveness of these techniques can greatly influence research outcomes. Different situations require specific methods. Understanding each method helps in choosing the right approach for given objectives.
Chromatography
Chromatography is a versatile technique. It separates mixtures based on component interactions with stationary and mobile phases. The importance of chromatography lies in its broad applicability across various fields, such as pharmaceuticals, environmental science, and food safety. It provides precise results, making the technique an integral part of analytical chemistry.
Gas Chromatography
Gas Chromatography (GC) is particularly useful for volatile compounds. It has high sensitivity and resolution. GC allows the separation of gases and vapors quickly. Its key characteristic is the use of gas as the mobile phase. This makes it a beneficial option for volatile analytes. One unique feature is its ability to analyze complex mixtures with ease. However, GC may not be suitable for non-volatile samples or thermally unstable compounds, limiting its scope.
Liquid Chromatography
Liquid Chromatography (LC) addresses the challenges of non-volatile substances. This method uses a liquid mobile phase, providing flexibility in sample types. It is widely popular due to its broad application. One important feature is the variety of detectors available. These detectors can enhance sensitivity and selectivity. Some disadvantages include longer run times compared to GC and potential solvent usage concerns.
Thin-Layer Chromatography
Thin-Layer Chromatography (TLC) is simpler and cost-effective. This technique allows for quick qualitative analysis. TLC involves a stationary phase coated on a plate and uses a solvent as the mobile phase. Its key characteristic is ease of use. It is an ideal choice for rapid screening of compounds. However, TLC lacks the quantitative precision of other techniques. It is more suitable for preliminary analysis rather than detailed measurements.
Spectroscopy
Spectroscopy encompasses various techniques to analyze the interaction of light with matter. It is critical for identifying chemical structures and quantifying substances. The importance lies in its fast and non-destructive nature, which enhances experimental efficiency.
UV-Vis Spectroscopy
UV-Vis Spectroscopy focuses on ultraviolet and visible light absorption. It is popular due to its simplicity and speed. The key feature is its ability to provide quantitative results with minimal sample preparation. One advantage is its wide applicability in different fields, including biochemistry and environmental analysis. However, it requires the sample to be in solution form, which limits some analyses.
Infrared Spectroscopy
Infrared Spectroscopy (IR) is essential for identifying functional groups in molecules. This technique measures vibrational transitions in molecules. Its key characteristic is the ability to provide structural information quickly. IR is widely used in organic chemistry for its efficiency. However, complex mixtures may give overlapping signals, complicating analysis.
Nuclear Magnetic Resonance Spectroscopy
Nuclear Magnetic Resonance Spectroscopy (NMR) offers detailed information about molecular structure. It uses magnetic fields and radio waves to observe nuclei. This technique is highly beneficial for organic compounds. One of its unique features is the ability to provide information about chemical environments. However, NMR requires relatively large sample sizes and can be expensive, which may limit its widespread use.
Advanced Instrumentation in Analytical Chemistry
Advanced instrumentation is pivotal for the progress of analytical chemistry. It encompasses a range of techniques that make analysis more precise, efficient, and cost-effective. As the demands of research and industry grow, the need for sophisticated tools increases. This section delves into two major techniques: mass spectrometry and electrochemical analysis, highlighting their importance and applications in analytical chemistry.
Mass Spectrometry
Mass spectrometry (MS) is a powerful analytical technique used for identifying the composition of a sample by measuring the mass of its molecules. This method has become indispensable in various fields, including pharmaceuticals and environmental science.
The process involves ionizing chemical species and sorting the ions based on their mass-to-charge ratio. The ability to analyze complex mixtures makes mass spectrometry an essential tool. It allows for:
- Molecular Weight Determination: MS provides accurate molecular weights, aiding in the identification of unknown substances.
- Structural Analysis: Information gained from fragmentation patterns enables the deduction of structural details of molecules.
- Sensitivity and Speed: Modern mass spectrometers can detect minute quantities of analytes in fractions of a second, a crucial factor in research where time and precision are critical.
In the pharmaceutical industry, mass spectrometry is commonly used for analyzing drug metabolism and development processes. By obtaining detailed profiles of bioactive compounds, researchers can forecast a drug's behavior in biological systems. This leads to more effective and safer drugs.
Mass spectrometry stands at the intersection of speed and accuracy, transforming how we understand chemical compositions.
Electrochemical Analysis
Electrochemical analysis utilizes electrical measurements to study chemical processes occurring at the interface of electrodes and electrolytes. It is particularly significant in areas like environmental monitoring, food safety, and clinical analysis.
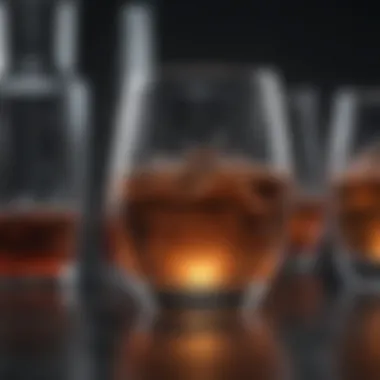
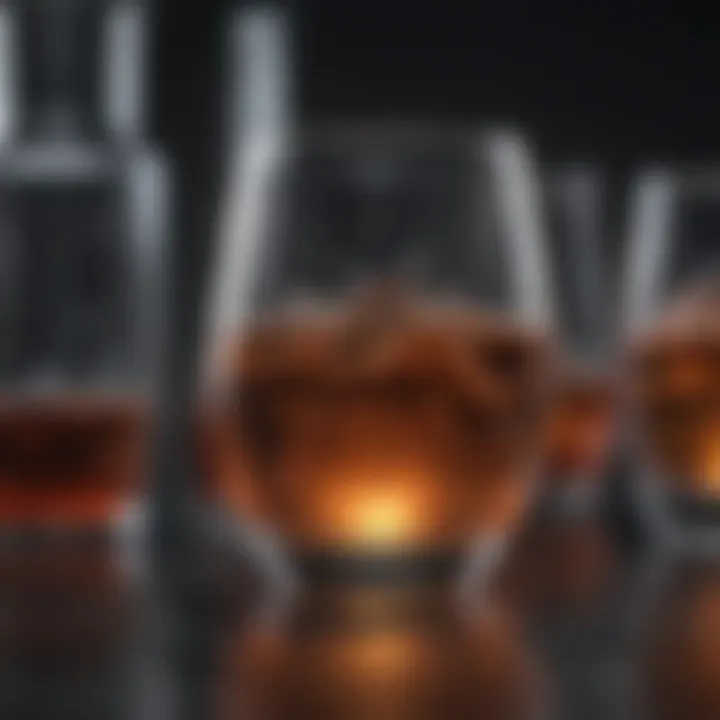
The common techniques include potentiometry and voltammetry, which provide real-time data regarding chemical species. The benefits of electrochemical analysis include:
- High Sensitivity: Electrochemical methods can detect low concentrations of substances, making them suitable for trace analysis.
- Cost-Effectiveness: These techniques often require relatively simple equipment compared to other advanced methods.
- Versatile Applications: From monitoring heavy metals in water to glucose monitoring in diabetes management, electrochemical methods have versatile implementations.
In environmental chemistry, electrochemical sensors enable the monitoring of contaminants in real time. This is crucial for ensuring compliance with safety standards and protecting public health. The integration of these methods in various fields illustrates the significant role of electrochemical analysis in advancing analytical chemistry.
In summary, advanced instrumentation is integral to enhancing analytical methodologies. Mass spectrometry and electrochemical analysis exemplify how advanced tools lead to more efficient, accurate, and reliable results in diverse applications.
Applications of Analytical Chemistry
Analytical chemistry plays a crucial role in various fields, enabling professionals to gather, interpret, and use data efficiently. The applications of analytical chemistry are extensive, influencing sectors such as healthcare, environmental science, and food safety. Its significance lies not only in enhancing quality control but also in ensuring public health and safety. As we explore the different ways analytical chemistry is applied, we focus on its contributions and the considerations required in each area.
Pharmaceutical Industry
In the pharmaceutical field, analytical chemistry is fundamental for drug development and quality assurance. The ability to identify the composition of compounds and measure their effective concentration is essential for creating safe and effective medications. Analytical techniques, such as High-Performance Liquid Chromatography (HPLC) and Mass Spectrometry (MS), are routinely employed for both drug formulation and stability testing.
Through methodical validation and testing, these techniques help in:
- Determining active ingredients
- Analyzing impurities
- Confirming the stability of drugs
Moreover, regulatory compliance demands rigorous testing to ensure that pharmaceutical products meet strict standards. Various agencies, like the Food and Drug Administration (FDA), rely on analytical chemistry to validate drug safety and efficacy before they can enter the market.
Environmental Monitoring
Analytical chemistry also holds a pivotal place in environmental monitoring. With the growing concern for pollution and ecological health, the ability to monitor environmental samples is more critical than ever. Techniques in this area help assess water, air, and soil quality, identifying contaminants that may pose risks to human and ecological health.
Key analytical methods include:
- Gas Chromatography (GC) for checking air contaminants
- Atomic Absorption Spectroscopy (AAS) for analyzing heavy metals in water
- Biochemical Oxygen Demand (BOD) tests to measure organic matter in water samples
These methods empower regulatory bodies and researchers to monitor compliance with environmental standards and formulate appropriate responses to pollution crises.
Food Safety and Quality Control
The application of analytical chemistry extends significantly into food safety and quality control. Consumers demand assurance that the food they eat is free from contaminants and hazardous substances. Analytical techniques enable the detection of residues, additives, and pathogens in food products.
Commonly used methods include:
- Gas Chromatography-Mass Spectrometry (GC-MS) for detecting pesticide residues
- Fourier Transform Infrared Spectroscopy (FTIR) for identifying food adulteration
- Enzyme-linked Immunosorbent Assay (ELISA) for pathogen detection
By ensuring food quality, these methods help maintain public trust and health. Moreover, they assist in regulatory compliance, helping to prevent foodborne illnesses.
"Analytical chemistry provides the tools required to ensure safety, efficacy, and quality across various industries."
The interdisciplinary nature of analytical chemistry enables it to adapt to the changing needs of these sectors, ensuring ongoing advancements and improvements to techniques applied in real-world contexts.
Emerging Trends in Analytical Chemistry
Emerging trends in analytical chemistry represent a vital aspect of the field, reflecting continual innovation in methodologies and technologies. As the need for faster and more accurate analytical results increases, these trends address the demands of various sectors such as pharmaceuticals, environmental science, and food quality. The modernization of analytical techniques not only enhances productivity but also offers accurate data analysis which is crucial in scientific research and industrial applications.
One of the central focuses in this area is the shift towards more compact and efficient tools for analysis. With increasing pressure on scientists to perform high-throughput screening and real-time analysis, traditional methods often become inadequate. Thus, it is essential to keep abreast of these trends to understand their significant benefits and potential limitations.
Miniaturization of Analytical Techniques
The miniaturization of analytical techniques has emerged as a prominent trend. This process involves reducing the size of devices and samples while maintaining, or even improving, performance levels. Technologies like microfluidics enable precise manipulation of fluids at the microscale. The benefits of miniaturization are notable. It leads to:
- Reduced Material Use: Smaller samples mean less reagent and sample consumption.
- Increased Throughput: Miniaturized systems allow for simultaneous analyses of multiple samples, significantly improving efficiency.
- Enhanced Sensitivity: Smaller volumes can lead to improved signal detection due to reduced noise.
In practical applications, devices such as microarray instruments and lab-on-a-chip systems exemplify miniaturization’s effectiveness. These systems are increasingly utilized in diagnostics, environmental monitoring, and biological studies, transforming how data is collected and interpreted.
Integration with Artificial Intelligence
Another trend is the integration of artificial intelligence with analytical chemistry techniques. As data complexity grows, traditional data analysis methods fall short in extracting valuable insights. Leveraging AI allows for:
- Data Processing: AI algorithms can analyze large datasets, identifying patterns and correlations that may not be evident through manual analysis.
- Predictive Modeling: Machine learning can predict outcomes based on existing data, streamlining processes in drug development and environmental monitoring.
- Automation: AI-driven automation systems minimize human error and enhance reproducibility in experiments.
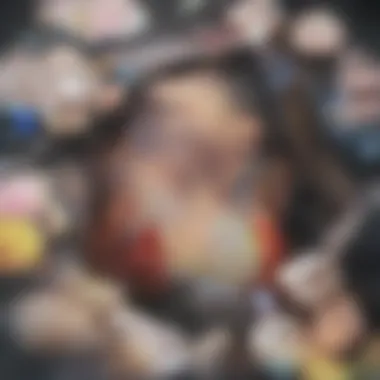
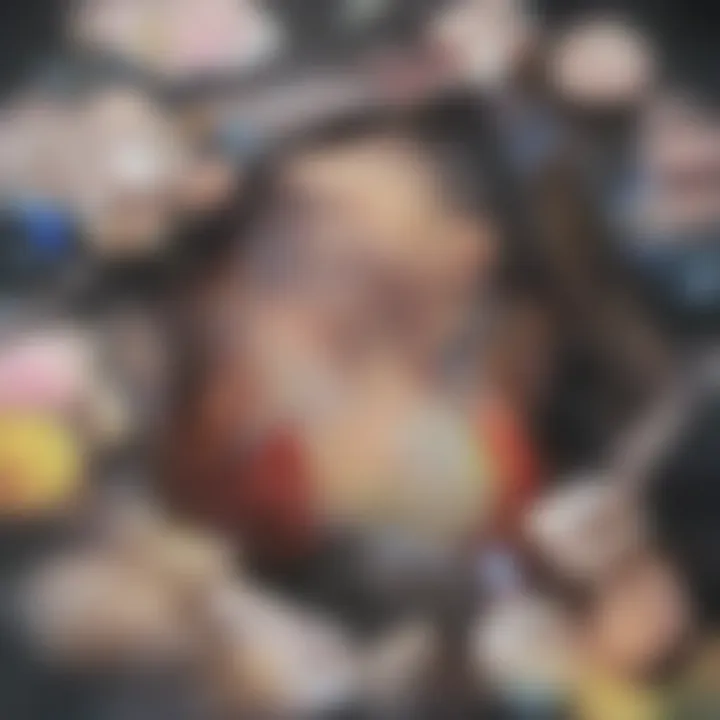
These technological advancements herald a shift towards more sophisticated analysis frameworks. By fusing AI with analytical chemistry, scientists can drive innovation, leading to breakthroughs in various fields, especially those demanding high levels of precision and efficiency.
"Incorporating AI in analytical practices provides tools that not only enhance operational effectiveness but also offer profound insights into complex datasets."
Validation and Quality Assurance in Analytical Practices
Validation and quality assurance are fundamental components in analytical chemistry. They ensure that analytical methods yield reliable, accurate, and reproducible results. This is critical in various fields, such as pharmaceuticals, environmental monitoring, and food safety. For practitioners, understanding these concepts can enhance the credibility of their findings and comply with regulatory requirements.
Quality assurance entails a systematic approach to ensure the quality of results throughout an analytical process. It involves regular monitoring and evaluating all aspects of the laboratory work. Implementing robust quality assurance protocols minimizes errors and deviations that could compromise the integrity of research findings.
"Validation ensures that an analytical method is fit for its intended purpose."
Method Validation Protocols
Method validation is a documented process that confirms an analytical method's suitability for its intended use. The protocols usually focus on parameters such as specificity, linearity, precision, accuracy, detection limit, and robustness. Each of these elements provides insights into how well the method performs under various conditions.
- Specificity refers to the ability of a method to measure the analyte of interest while excluding other components.
- Linearity assesses the method's response across a given range. It confirms that changes in concentration lead to proportional changes in signal.
- Precision indicates the reproducibility of results under defined conditions, while accuracy measures how close results are to true values.
- Detection limit defines the smallest amount that can be reliably measured, and robustness evaluates method performance under varying conditions.
Utilizing these protocols aids laboratories in assuring stakeholders of the reliability of their results.
Regulatory Standards
Regulatory standards are established by organizations such as the FDA, ISO, or EPA. These standards create a framework governing how analytical methods should be validated. Compliance with these standards is often mandatory and protects public health and safety. Each regulatory body might have specific guidelines regarding validation processes.
For example, the FDA mandates drug testing laboratories to adhere to Good Laboratory Practices (GLP), which includes stringent requirements on method validation. These regulations also necessitate that laboratories maintain records of validation tests to ensure accountability and transparency.
Adhering to regulatory standards not only bolsters the credibility of the research but also ensures that findings are accepted by the scientific community and policymakers.
Case Studies Illustrating Analytical Techniques
Case studies that illustrate analytical techniques provide practical examples of how these methodologies are applied in real-world scenarios. They serve as a bridge between theoretical knowledge and practical execution. By examining specific instances, professionals and students can gain insights into the effectiveness of various methods and the challenges encountered during their implementation. This section will discuss two significant case studies: novel drug development and pollution tracking in ecosystems.
Novel Drug Development
The pharmaceutical industry heavily relies on analytical chemistry to ensure drug safety and efficacy. Analytical techniques play a crucial role in each stage of drug development, from initial synthesis to final formulation. In a recent case study, researchers focused on using liquid chromatography paired with mass spectrometry to characterize new drug candidates. This combination enables the identification of compounds present in complex mixtures.
The researchers followed stringent analytical procedures to validate their methodologies, ensuring reproducibility and reliability. They evaluated the pharmacokinetic properties of the drug candidates, analyzing absorption, distribution, metabolism, and excretion. The results highlighted how analytical chemistry facilitates informed decision-making in the development pipeline. The incorporation of these techniques not only accelerates the discovery phase but also addresses regulatory compliance.
Pollution Tracking in Ecosystems
Environmental monitoring is another area where analytical chemistry proves indispensable. A notable case study involved the assessment of heavy metal contamination in freshwater ecosystems. Scientists employed atomic absorption spectroscopy to analyze water samples collected from different sites. This technique allows for the precise quantification of elements like lead, cadmium, and mercury.
Through systematic sampling and analysis, researchers identified contamination sources and levels. The findings prompted immediate action from local authorities, showcasing how analytical techniques can influence policy and environmental conservation efforts. Such case studies underscore the importance of analytical chemistry in safeguarding public health and sustaining ecological balance.
These case studies not only illuminate the practical applications of analytical chemistry but also reinforce the necessity for continual methodological advancements. They demonstrate the profound impact of analytical techniques on drug development and environmental protection. As analytical chemistry evolves, so too does its capacity to address contemporary challenges, driving innovation in both the pharmaceutical and environmental sectors.
Future Directions in Analytical Chemistry
The field of analytical chemistry is on the cusp of significant transformations. As research demands evolve, so too must the methodologies employed in the discipline. Future directions will likely focus on enhancing sustainability, embracing educational advancements, and fostering broader accessibility. These elements are not only trends but essential components that call for immediate attention in both academic and professional settings.
Sustainability in Analytical Practices
Sustainability has become a crucial consideration across various fields, including analytical chemistry. The push towards greener methods aims to minimize waste and reduce environmental impact. Analytical chemists are aware that traditional practices can be resource-intensive, raising concerns about their ecological footprint.
Adopting sustainable techniques involves several strategies:
- Use of Green Solvents: Replacing hazardous solvents with safer alternatives can significantly reduce the health and environmental risks associated with chemical analysis.
- Minimizing Waste: Chemiest can modify methods to use fewer reagents, which not only cuts costs but also lessens the potential for dangerous waste products.
- Energy Efficiency: Innovations in instrumentation should emphasize low-energy operations, contributing to a net decrease in energy consumption.
Furthermore, regulatory organizations are establishing guidelines to ensure that the methods used are not only effective but also responsible. The adoption of sustainable practices will enhance the reputation of analytical chemistry as a forward-thinking field committed to addressing global challenges.
"The future of analytical chemistry is intertwined with sustainability. As we innovate, we must also think of the planet."
Educational Advancements and Accessibility
Education plays a pivotal role in shaping the future of analytical chemistry. Curricula must adapt to include current technologies and methodologies. Educational advancements also need to prioritize accessibility for a diverse audience, ensuring that knowledge and resources are available to all students and professionals.
Some key aspects to consider include:
- Integration of Technology: Online resources, virtual labs, and simulation software are becoming more widespread in teaching. This modern approach accommodates different learning styles and allows students to experiment without physical constraints.
- Collaborative Learning Opportunities: Partnerships between universities and industry can create pathways for students to engage with real-world problems, fostering a practical understanding of analytical techniques.
- Scholarship and Funding: Increased funding for underrepresented students in STEM fields can help bridge the gap in educational access. Scholarships can support future chemists in developing skills necessary for the evolving landscape.
A focus on educational advancement not only strengthens the foundation of analytical chemistry but prepares upcoming generations for the challenges they will face. It is vital that we ensure an equitable approach to education in this field as it grows in complexity and relevance.