The Impact of Karl Deisseroth on Optogenetics and Neuroscience
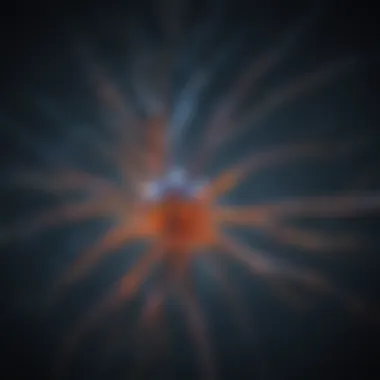
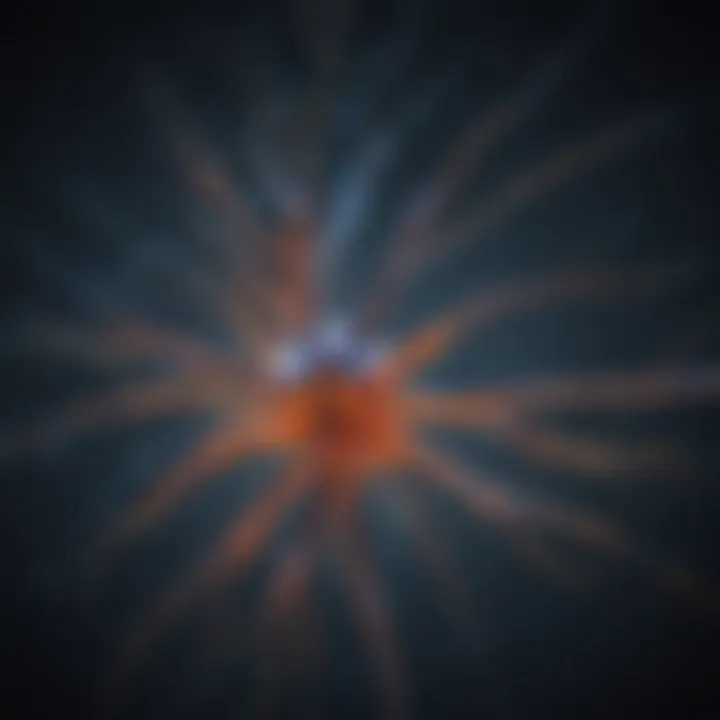
Intro
Karl Deisseroth stands at the vanguard of neuroscience, particularly through his monumental contributions to optogenetics. This powerful technique leverages light to control and manipulate neuronal activity, fundamentally altering our understanding of brain functionality. By bridging biology with engineering, Deisseroth's work has opened doors to explore complex behaviors and brain disorders with unprecedented precision.
As we delve into the intricacies of his research, a clear narrative emerges—one that underscores the scientific rigor, innovation, and ethical considerations that accompany such groundbreaking advancements. This article aims to provide a comprehensive exploration of Deisseroth's contributions, dissecting various aspects of optogenetics, from foundational mechanisms to applicable methodologies.
Research Methodology
Description of Research Design and Approach
The research design employed in studying optogenetics, particularly under Deisseroth's influence, encompasses both experimental and theoretical frameworks. His pioneering work begins with identifying light-activated proteins, such as channelrhodopsins, which are crucial for manipulating neuronal excitability. This biological insight forms the backbone of optogenetic methodologies.
Deisseroth's approach often integrates in vivo studies with advanced imaging techniques, allowing researchers to visualize and manipulate neuronal circuits in real-time. By employing laser light to target specific neurons, significant advances have been made in understanding the mechanistic underpinnings of various brain functions and dysfunctions.
Materials and Methods Used in the Study
The materials and methods utilized in Deisseroth's research are as multifaceted as the discipline itself. Key components include:
- Channelrhodopsins: Genetically engineered proteins that respond to blue light, allowing precise control of neuronal firing.
- Optical fibers: These are inserted into brain regions to deliver light to targeted cells, enabling real-time manipulation of neural circuits.
- Genetic tools: Techniques such as CRISPR/Cas9 are employed to modify genes in order to express these light-sensitive proteins selectively.
- Imaging systems: Methods like two-photon microscopy provide insights into the dynamics of neuronal activity during optogenetic stimulation.
These elements combined create a robust framework for conducting cutting-edge research that pushes the boundaries of neuroscience.
"Optogenetics offers unparalleled precision to probe the brain's intricate wiring, allowing us to untangle the mysteries of neuronal networks."
Discussion and Interpretation
Interpretation of Results in the Context of Existing Literature
Deisseroth's findings frequently reinforce or challenge established notions in neuroscience. For instance, the ability to activate or silence specific populations of neurons sheds light on their distinct roles in cognition and behavior. Such studies enhance our understanding of neurological disorders, linking particular neural circuits to conditions such as epilepsy and depression.
As more researchers adopt optogenetic techniques, the collective knowledge grows. Data derived from these experiments contribute to a more nuanced comprehension of brain functions, akin to piecing together a complex jigsaw puzzle where each piece represents a neuron or a neural pathway.
Implications for Future Research or Practical Applications
As we peer into the future, the implications of Deisseroth’s work are staggering. The continued development of optogenetic tools promises to lead to new therapeutic strategies for treating neurological and psychiatric disorders. Moreover, as ethical frameworks evolve alongside technological advancements, it becomes imperative to consider the ramifications of manipulating brain activity on human and animal subjects. The dialogue between scientific innovation and ethical constraints will shape the path forward, ensuring that the momentum generated by figureheads like Deisseroth serves both scientific and societal needs.
Prologue to Optogenetics
Optogenetics has carved a unique niche in the scientific landscape, providing unprecedented insights into the workings of the brain and the intricate dynamics of neuronal circuits. In a world where understanding the minute details of neural interactions has been a daunting task, optogenetics offers a light-based approach that fundamentally changes the game. By enabling researchers to control and manipulate neuronal activity with precision, this technique unlocks avenues for exploration that were once thought to be out of reach.
Definition and Overview
At its core, optogenetics combines optics and genetics to turn neurons into light-sensitive cells. Scientists employ this technique by introducing genes from algae into specific neuron types, equipping them with proteins called channelrhodopsins that respond to light. Hence, when a flash of light hits these cells, they either activate or inhibit, allowing scientists to probe the effects of activating particular neurons on behavior and brain function. This method serves not just as a novel research tool but also as a bridge that links genetic manipulation with tangible effects on neuronal networks.
Such a cutting-edge technique opens doors to understanding various brain functions, from the basics of sensory perception to the underpinnings of psychological disorders. By controlling neuronal activity with light, researchers can observe immediate causative effects, giving a clearer picture of how the brain processes information.
Historical Context
The journey of optogenetics is a testament to the convergence of diverse scientific domains—from molecular biology to neuroscience. The roots trace back to early studies in algae, specifically Chlamydomonas reinhardtii, which revealed how certain proteins could respond to light. This foundational work set the stage, but it was the collaboration between Carl Deisseroth and his colleagues that truly distilled this potential into practical application.
In the mid-2000s, Deisseroth, alongside Edward Boyden and Karl Deisseroth, recognized the possibility of utilizing these light-sensitive proteins for deep-seated neural manipulation. The publication of their groundbreaking research marked a turning point. Shortly after, optogenetics gained traction in laboratories around the world, garnering attention for its capacity not only to illuminate the complexities of the brain but also to challenge previously held notions about brain functionality and behavior.
As researchers delved deeper, the significance of this technique began to unravel—allowing them to link specific neural circuits to behaviors, integrate sensory inputs with motor outputs, and investigate the anomalies present in neurological disorders. Thus, the historical context surrounding optogenetics is not simply about establishing a novel technique; it's about reshaping our understanding of neural processes and behaviors.
"Optogenetics allows us to dissect complex behaviors and mental states at a level of detail never before possible."
In summary, the intersection of genetics and optics in neuroscience signifies a monumental advance in our quest to decode the enigma of brain function. With each advancement, optogenetics continues to challenge preconceptions, offering a clearer lens through which we can study the most complex organ in the body.
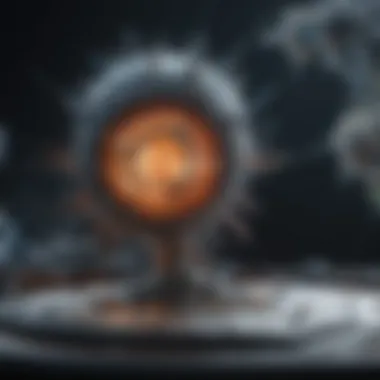
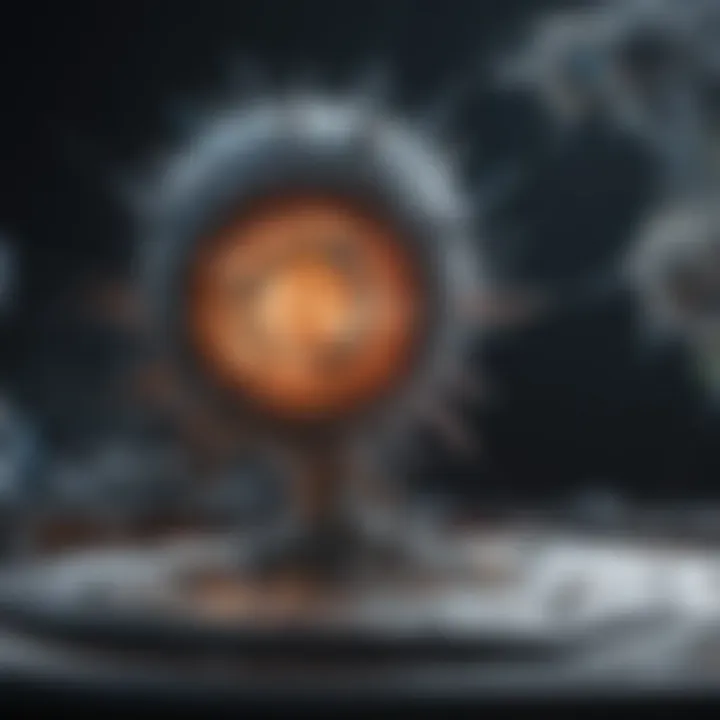
Karl Deisseroth: A Brief Biography
Karl Deisseroth has emerged as a pivotal figure in paving the path for advancements in optogenetics, a field that fundamentally reshapes our understanding of neuronal function and behavior. Knowing about his life, education, and career provides valuable insights into the driving forces behind his innovations. By exploring Deisseroth’s biography, we begin to appreciate not only his scientific breakthroughs but also the personal experiences and intellectual rigor that have informed his pursuits in neuroscience.
Early Life and Education
Karl Deisseroth was born in 1971 in the state of California, where his curiosity about the brain ignited at a young age. Growing up, he actively engaged with various scientific disciplines, but it was during his undergraduate years at Stanford University that his passion for neuroscience truly blossomed. There, he pursued a degree in biology, fostering a strong foundational knowledge that he would later build upon in his groundbreaking work.
After completing his undergraduate studies, Deisseroth took a detour by enrolling in medical school, also at Stanford. This dual training in both medicine and neuroscience equipped him with a unique perspective, blending clinical insights with research-driven techniques. His early focus was on psychiatry, which ultimately guided his research orientation towards understanding the underlying neural mechanisms of mental disorders.
Deisseroth's education culminated in a PhD in neuroscience, where he started investigating neural circuits and their functional implications. His rigorous academic journey laid the groundwork for his innovative leaps in optogenetics.
Career Milestones
Deisseroth's career is characterized by a series of remarkable milestones that illustrate his profound impact on neuroscience. After completing his medical and doctoral studies, he began his postdoctoral work under the mentorship of biochemist Ed Boyden. Together, they embarked on a path that would lead to a scientific revolution: the development of optogenetics.
In 2005, their research culminated in the introduction of channelrhodopsins to neuroscience. This innovative advance allowed researchers to precisely control the activity of nerve cells using light, empowering osciating scientists to explore the intricate workings of the brain like never before. Deisseroth's role in this effort was not merely as a contributor; his insights and experimental design significantly shaped the methodologies that would dominate neuroscience research practices.
Highlights from Deisseroth’s career include:
- 2005: Development of channelrhodopsins, a major breakthrough in optogenetics.
- 2007: His publication detailing the application of optogenetic techniques in freely-behaving mammals drew the attention of the scientific community and set the stage for further exploration.
- 2010s: Deisseroth founded the Stanford Neuroscience Institute, further consolidating his role as a leader in the field.
- Numerous awards and recognitions: His contributions have earned him prestigious accolades, placing him among the leading scientists of his generation.
Overall, understanding Karl Deisseroth’s life and career is key to unraveling the context of his monumental contributions to optogenetics. His journey reflects how academic rigor, curiosity, and interdisciplinary approaches can culminate in groundbreaking innovations that expand the horizons of science.
Mechanisms of Optogenetics
Understanding the mechanisms of optogenetics is pivotal for grasping how this technology has transformed neuroscience. It fundamentally blends biology and engineering, allowing scientists to control and observe neuronal activity with remarkable precision. This section delves into the intricacies of how optogenetics functions, emphasizing its significance through light-activated proteins and neuronal manipulation techniques.
Light-Activated Proteins
At the heart of optogenetics are light-activated proteins, a unique class of proteins that respond to specific wavelengths of light. One of the groundbreaking discoveries in this domain is the identification and characterization of channelrhodopsins, proteins derived from green algae, that facilitate the flow of ions when exposed to light. This ability to induce ion flow is essentially how researchers can manipulate neuronal activity.
These proteins serve as the "gatekeepers" of neuronal firing. By shining light on them, scientists can either activate or inhibit neurons in real time. Imagine being able to switch a light bulb on and off, but instead, you’re finely tuning neurons in a living organism. This opens up a world of possibilities for understanding brain functions.
Benefits of Light-Activated Proteins
- Precision: This method allows for targeted manipulation of specific neuronal populations, which is crucial for deciphering complex neural circuits.
- Temporal Control: With millisecond precision, researchers can control when neurons fire, enabling them to study dynamics that were previously inaccessible.
- Reversibility: The ability to stop or start the activation with light provides a non-invasive way to alter brain activity without permanent changes.
"The ability to manipulate cells using light is akin to a maestro conducting an orchestra, where each neuron plays its unique note in the grand symphony of brain function."
Despite these advancements, researchers also confront challenges. Light penetration in biological tissues can limit effectiveness, as can the need for precise control over light application. These considerations are paramount when designing experiments that will accurately reflect natural brain activity.
Neuronal Manipulation Techniques
The various techniques developed for neuronal manipulation are vital for the practical application of optogenetics. They enable the intricate dance between light and biology to unfold in ways that have changed our understanding of the brain profoundly.
Key Techniques
- In Vivo Techniques: This method involves directly applying optogenetic tools in living organisms, allowing for real-time observation of neuronal behavior in its natural context.
- Fiber Optic Delivery Systems: Using fiber optics, researchers can deliver light to specific brain regions with remarkable accuracy, decreasing the chances of unintended stimulation of surrounding areas.
- Calcium Imaging Integration: Pairing optogenetics with calcium imaging allows simultaneous observation and manipulation, adding another layer of depth to the understanding of neuronal circuits.
The development of these techniques has not only enhanced research methodologies but also broadened the scope for further studies in related fields such as behavioral science and cognitive psychology. By unlocking the mechanisms of neuronal activity in these ways, scientists can gain insights into disorders like epilepsy, depression, and schizophrenia, paving the way for innovative therapeutic strategies.
In summary, the mechanisms behind optogenetics hold tremendous potential. Through the use of light-activated proteins and advanced manipulation techniques, researchers are peeling back the layers of complexities within the brain, allowing for deeper understanding and investigation into the functional architecture of neural systems.
Innovations Introduced by Deisseroth
Karl Deisseroth's contributions to optogenetics mark a significant leap forward in the manipulation of neural circuits. His innovations have galvanized the field of neuroscience, leading to unprecedented insights into how the brain operates. By merging genetic engineering with optical control, Deisseroth opened doors that were previously firmly shut, allowing researchers to delve deeper into the mysteries of neural pathways.
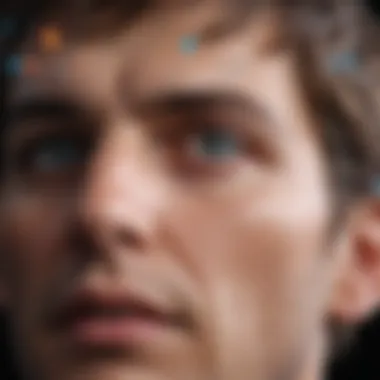
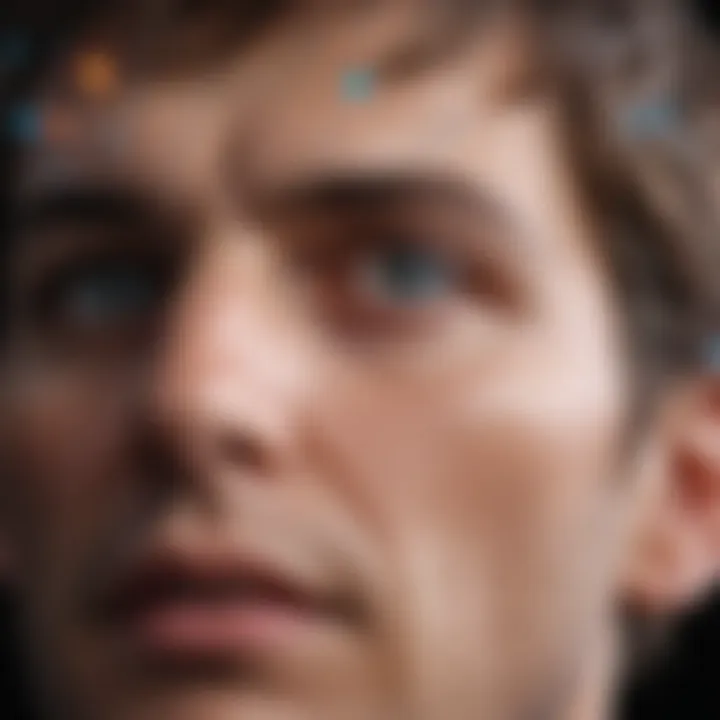
The innovations presented by Deisseroth are not merely academic exercises; they are the foundation for transformative discoveries that have reshaped our understanding of neurobiology and behavior. Through carefully engineered proteins that respond to light, scientists can now modulate neuronal activity with extraordinary precision. This ability to control neurons at will—turning them on or off with mere flashes of light—can lead to fundamental breakthroughs in therapies for neurological disorders, behavioral modification, and more.
Development of Channelrhodopsins
One of Deisseroth's crowning achievements is the development of channelrhodopsins, light-sensitive proteins that are essential for optogenetic techniques. Originally found in algae, these proteins can be genetically introduced into specific types of neurons. Once in place, researchers can use light to activate or inhibit these neurons. The implications are staggering: instead of observing neuron behavior as a passive spectator, scientists can now become active participants in their research.
The versatility of channelrhodopsins extends beyond simple activation. Various types have been engineered to respond to different wavelengths of light, enabling multiplexing in experiments. A common example is the use of blue light to excite channelrhodopsin-2 (ChR2), which leads to depolarization of the target neuron, while green light can be used to activate other light-responsive proteins, like halorhodopsin, that inhibit neural activity. This precision offers the ability to dissect intricate neural circuits and observe their behaviors in real time, elevating the level of understanding in neurophysiology.
Advancements in Genetic Targeting
Another notable advancement that Deisseroth led in optogenetics is the enhancement of genetic targeting techniques. Traditional methods of targeting cells were akin to throwing darts in the dark, relying heavily on chance for successful neuron selection. Deisseroth's innovations incorporated tools such as Cre-Lox recombination, which enables precise control over gene expression in specific tissues or cell types.
With genetic targeting, researchers can achieve a high degree of specificity when applying optogenetic tools. For instance, they can selectively target certain populations of excitatory or inhibitory neurons, a game-changer for dissecting the underlying mechanisms of complex behaviors. Moreover, current advancements allow for the combination of optogenetics with other methods like in vivo imaging, which brings about even richer datasets for understanding brain function.
"The integration of genetic targeting with optogenetics has laid the groundwork for a reconsideration of how we approach neural systems, effectively pushing the envelope of what is scientifically possible."
As a result, the research community is better equipped than ever to study the dynamics of neural circuits and their role in behavior. Deisseroth’s pioneering efforts in both channelrhodopsin development and genetic targeting not only paved the way for advancements in optogenetics but also provided the tools needed for future generations of neuroscientists to explore uncharted territories of the brain.
Applications in Neuroscience
Optogenetics stands at the frontier of neuroscience research, with its innovative applications enabling researchers to dissect the complexities of brain function. Karl Deisseroth's contributions have ushered in a new era, bridging the gap between molecular biology and neuroscience, thus allowing scientists to study neural circuits and behaviors in a far more nuanced manner. The ability to manipulate specific neurons with light paves the way for a host of studies that were previously unattainable, such as real-time observations of neuronal activity and its correlation with cognitive processes.
One major benefit of applying optogenetics in neuroscience is its unmatched precision. Unlike traditional methods that may affect a broad swath of neurons, optogenetics allows for specific targeting of individual cells or groups of cells based upon various criteria. This selective approach helps researchers get to the root of brain functions and behavioral outputs. For instance, one might selectively activate parvalbumin-expressing interneurons to explore their role in anxiety behavior, yielding insights that could translate into therapeutic interventions.
Moreover, the application of optogenetics in neuroscience goes beyond mere observation. It fosters a deeper understanding of how neural circuits underpin behaviors and cognitive processes, enhancing our grasp of the connection between structure and function in the brain. As Deisseroth's techniques have advanced, the exploration of these intricate relationships has gained momentum, making it imperative to dive into specific applications.
Optogenetics Beyond Neuroscience
The impact of optogenetics stretches far beyond the confines of neuroscience, reaching into various fields that can greatly benefit from its innovative approach. As researchers continue to harness this technology, it enables exploration into areas previously deemed challenging or impossible. Optogenetics, fundamentally the use of light to control biological processes, presents adiverse array of applications, each providing unique insights and advancements.
Applications in Vision Research
In vision research, optogenetics opens new doors by allowing scientists to understand and manipulate the complex pathways involved in visual perception. One prominent area of focus is in the treatment of retinal degenerative diseases. Conditions such as retinitis pigmentosa cause progressive loss of photoreceptor cells, leading to vision impairment or even blindness. With optogenetic techniques, researchers can introduce engineered light-sensitive proteins into remaining retinal cells. This modification enables these cells to respond to light, effectively restoring some level of vision in affected individuals.
For example, researchers have successfully used channelrhodopsins to convert non-light-sensitive retinal cells into those that can respond to visual stimuli, bringing significant hope to patients suffering from these debilitating conditions. This approach not only provides a profound therapeutic avenue but also enriches our understanding of how visual information is processed in the brain.
"Optogenetics allows us to manipulate neurons with high precision, leading to breakthroughs that were once mere dreams within the scientific community."
The integration of optogenetics in vision research also extends to fundamental studies on how visual information is transmitted and processed in neural circuits. By precisely controlling the activity of specific types of neurons, scientists can map out the intricacies of the visual pathway — from the retina, through the lateral geniculate nucleus, to the visual cortex. This kind of exploration helps elucidate the fundamental principles governing sight, contributing to advancements in areas such as computer vision and artificial intelligence.
Cardiovascular Studies
Interestingly, the applications of optogenetics do not solely rest within the neural domain; cardiovascular studies are increasingly becoming a promising field of exploration. Heart disease remains a leading global health challenge, prompting a need for novel approaches to understand cardiac function and develop targeted therapies.
Optogenetics facilitates the study of cardiac rhythms and contractility with remarkable precision. Researchers can use light-sensitive proteins to control the activity of specific heart cells, such as pacemaker cells, leading to real-time insight into the electrical signals that orchestrate heartbeats. With a better understanding of these mechanisms, scientists can identify potential therapeutic targets for heart arrhythmias and other cardiovascular disorders.
In preclinical models, optogenetic stimulation has been shown to correct abnormal heart rhythms, raising hopes for non-invasive treatments in the future. Additionally, optogenetic approaches allow detailed studies of the interactions between the nervous system and cardiac function. This critical understanding opens avenues for developing therapies that consider the integral role of the autonomic nervous system in heart health.
Ultimately, the journey of optogenetics beyond neuroscience provides a testament to its versatility. From addressing vision deficits to advancing cardiovascular research, the technology continues to pave the way for groundbreaking scientific discoveries, fostering innovations with the potential to enhance lives across multiple domains.
Ethical Considerations
The integration of optogenetics into various fields raises compelling ethical questions that demand thorough examination. These considerations serve not just to safeguard the integrity of scientific research but also to address broader societal implications. As researchers embrace this pioneering technology, it becomes critical to navigate the moral landscape surrounding its applications.
Implications for Animal Research
Optogenetics predominantly utilizes animal models to explore fundamental neurological mechanisms and potential treatments. While this approach has resulted in groundbreaking discoveries, it is essential to grapple with the ethical ramifications of using animals in research.
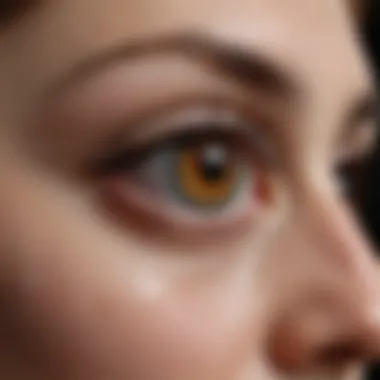
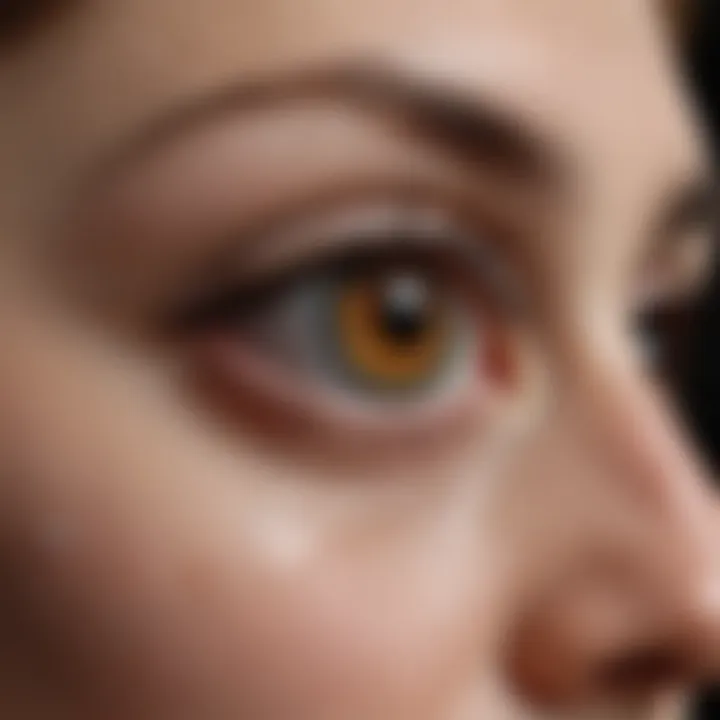
Some significant areas of concern include:
- Welfare of the Animals: It’s imperative to ensure that the wellbeing of animals is prioritized. Researchers must adhere to established protocols to minimize suffering and stress.
- Justification of Research: The scientific community must rigorously justify the necessity of animal experimentation. This means demonstrating that the expected benefits of the research significantly outweigh any negative impact on the animals.
- Alternatives to Animal Models: Some researchers advocate for the development of alternative methods, such as organ-on-a-chip technology or computer modeling, that could replace the need for live animal testing.
As the field advances, ongoing debates about these issues highlight a pressing need for a transformative shift towards more humane methodologies.
Human Applications and Ethics
The potential human applications of optogenetics extend far beyond the confines of animal research. From potential treatments of neurological disorders to possibilities in gene therapy, the promise is enormous, yet so are the ethical considerations.
Key factors include:
- Informed Consent: Before any procedures involving human subjects, it is crucial that informed consent is obtained. This includes assuring participants understand the potential risks and benefits of optogenetic interventions.
- Long-Term Consequences: The long-term impacts of altering neural circuits need thorough examination. Are there unintended changes in personality or cognition? Such inquiries challenge researchers to consider the broader implications of their work.
- Regulatory Frameworks: Establishing regulatory guidelines to govern the use of optogenetics in humans is essential. This ensures that experimentation adheres to stringent ethical standards while fostering innovation.
"The responsibility lies not only with researchers but also with society at large to consider the ethical dimensions of how we apply science in everyday life."
The ongoing conversations on these ethical considerations shape the future of optogenetic applications. We must ensure that the advances in technology do not outpace our ability to responsibly manage their implications.
Future Directions in Optogenetics Research
The field of optogenetics is continuously evolving, driven by advancements in technology and an increasing understanding of neuronal mechanisms. As we venture into the future, the relevance of exploring this area cannot be overstated. The potential applications of optogenetics stretch far beyond neuroscience alone, offering a wealth of opportunities for researchers and medical professionals alike. In this section, the focus is on the latest trends and innovations that mark the frontier of optogenetics, emphasizing how they promise to enhance our understanding of complex biological systems and pave the way for novel therapies.
Emerging Technologies
New techniques in optogenetics are sprouting up like wildflowers. Innovations such as next-generation optogenetic proteins and improved delivery methods show significant promise. Here are some pivotal advancements:
- Novel Opsins: Researchers are developing variants of opsins with unique wavelengths and faster kinetics. For instance, the introduction of new channelrhodopsin derivatives increases the speed of neuronal firing control, thereby allowing for more nuanced studies of brain function.
- CRISPR-based Techniques: Coupling optogenetics with CRISPR technology has opened new avenues for precise manipulation of gene expression. This convergence allows scientists to explore how genes interact with light at unprecedented resolutions.
- Wireless Systems: The introduction of wireless optogenetic technologies reduces the physical constraints on subject movement. This means that experiments can be conducted with more naturalistic behaviors, which can lead to more ecologically valid findings.
"The fusion of optogenetics with AI could provide insights that were unthinkable just a decade ago."
These advancements highlight an exciting shift in how researchers engage with neural circuits, making potential breakthroughs not merely conceivable but likely.
Enhancement of Precision and Control
Precision and control are the twin pillars upon which optogenetics stands. Achieving fine-tuned modulation of specific neuronal populations is what makes optogenetics so invaluable. Here are some areas where enhancement is crucial:
- Spatial Resolution: Future techniques aim at achieving sub-cellular resolution in neuronal targeting. Manipulating precise compartments of a neuron will allow researchers to understand local signaling processes, potentially revealing the intricacies of neural communication.
- Temporal Control: Innovations aimed at increasing the speed of optogenetic stimulation will deepen our understanding of the timing in synaptic transmission. This will enable scientists to explore dynamic changes in neural processing.
- Integration with Behavioral Protocols: Future designs will likely see optogenetics intertwined with advanced behavioral assays, making it possible to assess the impact of light-induced neuronal activity in real-time scenarios. This integration will heighten our ability to link specific neural circuits to behavioral outcomes.
As the future unfolds, these elements of enrichment will be vital for harnessing the full potential of optogenetics, enhancing its application in neuroscience and other fields.
In summary, the future of optogenetics research presents a landscape where emerging technologies and heightened precision promise to transform our understanding of the brain. Karl Deisseroth's foundational work is only the beginning, and as we navigate this dynamic domain, the possibilities seem limitless.
Finale
The conclusion of our exploration into the contributions of Karl Deisseroth to optogenetics serves as a critical reflection on the vast implications of his groundbreaking work. It's not just about the technological advancements, but also the way these innovations physiologically and ethically reshape our understanding of brain functionality. By mastering the interplay of light and neurons, Deisseroth has opened doors to new vistas in neuroscience, which could lead to significant breakthroughs in treatments for neurological disorders.
Summary of Key Insights
To encapsulate the essence of our discussion:
- Innovative Techniques: Deisseroth’s development of channelrhodopsins and genetic targeting methods has revolutionized the way we manipulate neuronal activity. These techniques allow for unprecedented precision in controlling brain circuits.
- Broad Applications: While the primary applications are in neuroscience, optogenetics extends into diverse fields like vision research and even cardiovascular studies. This versatility highlights its significance as a multi-disciplinary tool.
- Ethical Landscape: The ethical dimensions raised by Deisseroth's work demand careful consideration. As we harness these techniques, the implications for animal research and potential human applications must be tabulated.
In a nutshell, these insights encompass the relevance and necessity of Deisseroth’s contributions, underscoring how pivotal his work has been in shaping the trajectory of contemporary neuroscience.
The Legacy of Karl Deisseroth
Karl Deisseroth’s legacy is one etched in the annals of neuroscience history. As a pioneer, he has not only advanced scientific methods but has also instigated a paradigm shift in our understanding of the brain. His vision has inspired a generation of researchers, opening avenues for exploration that were once deemed implausible.
“The future belongs to those who believe in the beauty of their dreams.” — Eleanor Roosevelt
Deisseroth exemplifies this belief, having transformed ambitious dreams into tangible realities through rigorous scientific inquiry.
In terms of practical impact, many therapeutic paradigms originating from his research stand to make profound differences in treating psychiatric and neurological disorders. His work has cultivated a community committed to ethical scientific inquiry, promoting dialogues that bridge innovation with moral responsibility.
As we chart the future, the importance of his contributions cannot be overstated. It serves as a beacon for ongoing research and as a reminder of the powerful intersection between science, ethics, and human welfare.