An In-Depth Exploration of Mass Spectrometry Techniques and Applications
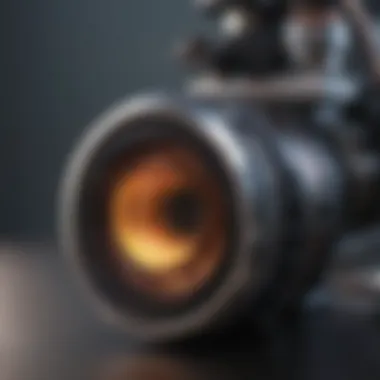
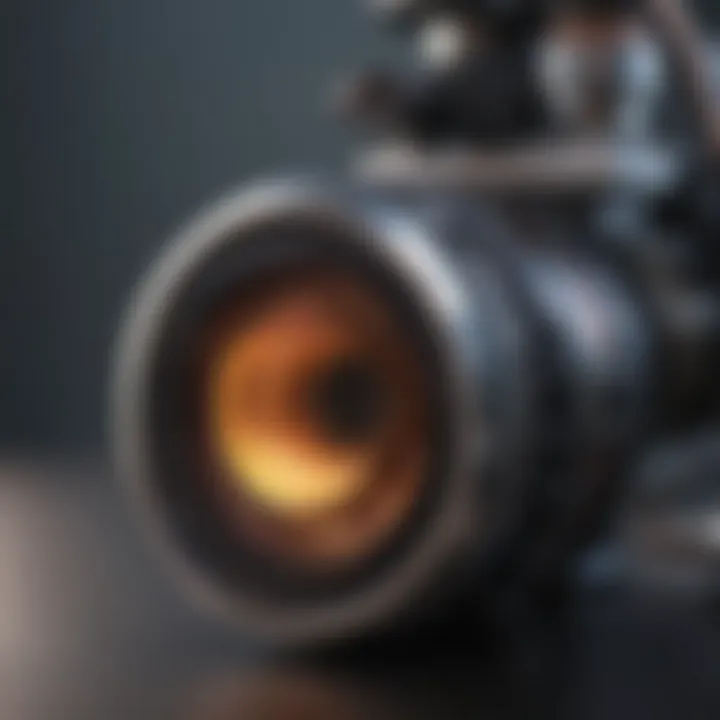
Intro
Mass spectrometry (MS) is an analytical technique that measures the mass-to-charge ratio of ions. It plays an important role in various fields such as biology, chemistry, and environmental science. The elegance of MS lies in its ability to analyze complex mixtures, making it indispensable in research and industry.
As we explore the different aspects of mass spectrometry, we will cover its fundamental principles, components of mass spectrometers, various methods, and practical applications. Additionally, ongoing developments and future directions in this field will be discussed.
This comprehensive guide aims to inform students, researchers, educators, and professionals about the significance of MS and its versatility in addressing modern scientific challenges.
Research Methodology
This section outlines the design and approach taken to explore mass spectrometry techniques and applications. The research methodology includes a systematic review of literature across multiple disciplines that utilize mass spectrometry.
Description of Research Design and Approach
The research design was based on evaluating existing studies, assessing empirical data, and synthesizing findings from academic journals, articles, and conference papers. A qualitative approach provided insights into the practical applications and technological advancements in mass spectrometry.
Materials and Methods Used in the Study
Materials for this study comprised peer-reviewed articles and case studies that highlighted various applications of mass spectrometry. The methods included:
- Compiling databases and catalogs relevant to mass spectrometry.
- Analyzing case studies to understand application effectiveness.
- Reviewing technological advances reported in the literature with respect to innovations in mass spectrometer technologies.
To ensure accurate and up-to-date information, the following databases were essential:
- Google Scholar
- PubMed
- ScienceDirect
By structuring research around these parameters, the study provides a well-rounded understanding of the current landscape of mass spectrometry.
Mass spectrometry is not merely a technique but a gateway to analytical possibilities across numerous scientific disciplines.
Discussion and Interpretation
Interpretation of Results in the Context of Existing Literature
The findings highlight the critical role mass spectrometry has played over the last few decades in various applications, particularly in proteomics, metabolomics, and environmental monitoring. Mass spectrometry continues to adapt and evolve, demonstrating its value in the face of advancing scientific inquiries.
Implications for Future Research or Practical Applications
As mass spectrometry evolves, it presents new opportunities for research. Areas that might benefit include:
- Integration with artificial intelligence for smarter data analysis.
- Development of portable mass spectrometers for field-based applications.
- Expansion into personalized medicine through better biomarker identification.
Intro to Mass Spectrometry
Mass spectrometry is a crucial analytical technique that has a significant impact across various fields such as chemistry, biology, and environmental science. Understanding mass spectrometry allows researchers to analyze molecular compositions, chemical structures, and different substances with high precision. This article aims to provide a comprehensive review of mass spectrometry techniques and applications, focusing not only on its principles but also on its transformative role in scientific research.
Definition and Importance
Mass spectrometry is defined as an analytical method used to measure the mass-to-charge ratio of ions. The importance of this technique lies in its ability to provide detailed information about the molecular weight and structure of compounds. The ability to detect trace amounts of substances makes mass spectrometry invaluable in various applications, including clinical diagnostics, drug development, and environmental analysis. By resolving complex mixtures into distinct components, mass spectrometry enables scientists to identify unknown substances and quantify target analytes.
Historical Context
The journey of mass spectrometry began in the early 20th century, with the development of the first mass spectrometer by J.J. Thomson in 1912. His invention laid the groundwork for future advancements in this field. Throughout the decades, mass spectrometry evolved with technological improvements, leading to various configurations of mass spectrometers. Significant milestones include the introduction of the quadrupole mass filter in the 1950s and the development of time-of-flight (TOF) mass spectrometry in the 1960s. These advancements have greatly expanded the scope of mass spectrometry, establishing it as a fundamental tool in analytical chemistry.
"The history of mass spectrometry is marked by continuous innovation that reflect humanityβs quest for deeper understanding of the molecular world."
Today, mass spectrometry is integral to modern scientific research. Its applications span clinical diagnostics to environmental monitoring, making it one of the foremost analytical techniques utilized in laboratories worldwide.
Core Principles of Mass Spectrometry
Understanding core principles of mass spectrometry is crucial, as they form the foundation for its diverse applications in scientific research. These principles define how mass spectrometry functions, allowing researchers to analyze complex mixtures and derive meaningful data. Mastery of these concepts not only facilitates accurate interpretations but also enhances the efficiency of experiments.
Basics of Ionization
Ionization is the first step in mass spectrometry, translating neutral atoms or molecules into charged particles. This process is vital, as only charged species can be manipulated using electric and magnetic fields. Various ionization techniques exist, each with unique advantages.
- Electron Ionization (EI) - Common in gas phase analyses, it uses electrons to ionize the molecules, producing positively charged ions.
- Electrospray Ionization (ESI) - Frequently employed for large biomolecules, ESI generates ions in solution, making it suitable for analyzing proteins and nucleic acids.
- Matrix-Assisted Laser Desorption Ionization (MALDI) - This method ionizes samples with a laser in the presence of a matrix, often used for analyzing polymers and biomolecules.
Ionization yield, energy input, and the stability of resultant ions are all key factors influencing the reliability of mass spectrometry data.
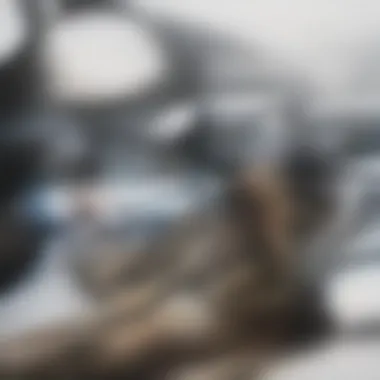
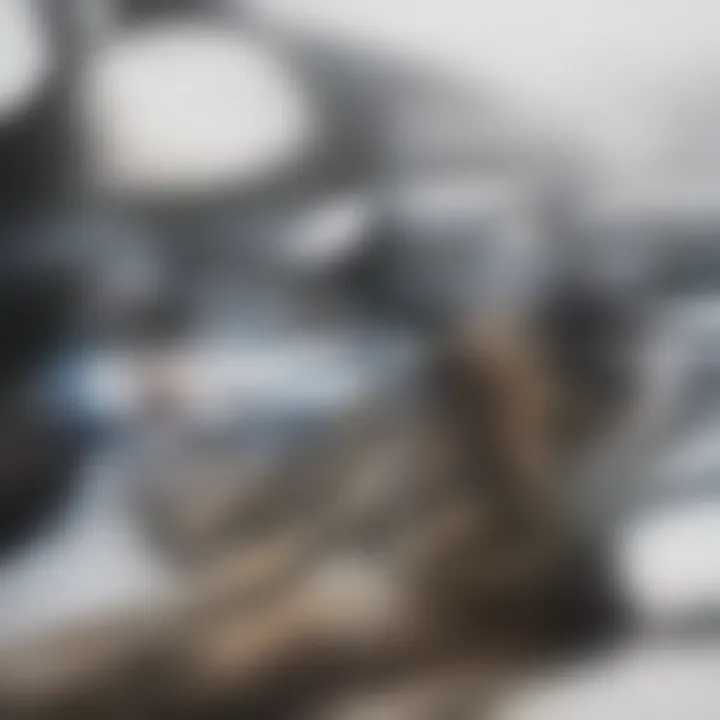
Mass Analyzers
Once ions are generated, mass analyzers measure their mass-to-charge ratios. This analysis is fundamental for identifying and quantifying compounds within a sample. Different types of mass analyzers have distinct operating principles and applications.
- Quadrupole Mass Analyzers - Utilize oscillating electric fields to filter ions based on their mass-to-charge ratio, widely regarded for their speed.
- Time-of-Flight (TOF) Mass Analyzers - Separate ions by measuring the time they take to travel a set distance. This method excels in analyzing high-mass compounds.
- Ion Trap Mass Analyzers - Capture ions in a defined space using electric fields, allowing for various manipulation techniques.
- Orbitrap Mass Analyzers - Measure ions based on their oscillation frequency, providing high-resolution data.
The choice of mass analyzer affects sensitivity, resolution, and speed of analysis, thus impacting overall experimental outcomes.
Detection Methods
The final stage involves detecting and quantifying the ions after analysis. Detection methods must accurately convert ion signals into measurable data, which is critical for reliable mass spectrometry results.
Common detection methods include:
- Faraday Cup Detectors - Measure ion current directly; they provide excellent accuracy but are slower compared to other detectors.
- Microchannel Plate Detectors - Offer high sensitivity and fast response times, commonly used in TOF and other mass analyzers.
- Electron Multiplier Detectors - Amplify ion signals significantly, facilitating the detection of low abundance ions.
Every detection method presents a trade-off between speed, sensitivity, and capability. Choosing the right technique is essential for obtaining meaningful results.
Understanding these core principles enables researchers to make educated decisions on the operational parameters of mass spectrometry, optimizing their data collection processes efficiently.
Types of Mass Spectrometry
Mass spectrometry encompasses multiple methodologies, each offering unique strengths and applications. Understanding these types enhances a researcher's capability to select the appropriate technique tailored to specific analytical challenges. This section delves into four principal types of mass spectrometry: Quadrupole, Time-of-Flight, Ion Trap, and Orbitrap mass spectrometry. Each of these techniques has its distinct operational principles, advantages, and areas where they excel, forming a crucial part of the broader mass spectrometry framework.
Quadrupole Mass Spectrometry
Quadrupole mass spectrometry operates using a four-pole electric field to filter ions based on their mass-to-charge ratio. The configuration of the quadrupole allows for highly selective ion analysis. This makes it particularly beneficial in applications requiring precise quantification of targeted compounds.
Commonly used in conjunction with liquid chromatography, Quadrupole mass spectrometry offers high sensitivity and rapid analysis times. This technique is widely adopted across various fields, including environmental science and clinical research. Considerations for its use include issues related to ion transmission efficiency and potential limitations in resolving power compared to other types.
Time-of-Flight Mass Spectrometry
Time-of-Flight (ToF) mass spectrometry is distinguished by its ability to measure the time ions take to travel a fixed distance. The faster the ion, the smaller its mass. This method delivers high-resolution data and can analyze a wide range of molecular weights, making it adaptable for numerous applications such as proteomics and polymer analysis.
One of the primary benefits of ToF is its capability for high-throughput analysis. However, inherent challenges include the need for precise timing mechanisms and the possibility of ion fragmentation during the analysis, which can complicate data interpretation.
Ion Trap Mass Spectrometry
Ion Trap mass spectrometry utilizes electromagnetic fields to trap ions. The ions can be held and controlled within a defined volume, which allows for extensive manipulation and analysis. This technique is notable for its high sensitivity and ability to perform various mass analysis such as MS/MS, which is crucial for structural elucidation of complex molecules.
Ion traps are versatile and can be integrated with other techniques like gas chromatography, enhancing analytical capabilities. The complexity of the instrumentation and potential issues of ion loss are important factors in its application.
Orbitrap Mass Spectrometry
Orbitrap mass spectrometry is noted for its high-resolution capabilities, as it determines the exact mass of ions based on their oscillation frequencies. This capability provides unprecedented insights into molecular structures, particularly in complex biological samples.
The Orbitrap is advantageous for its compact design and lower costs of operation compared to some traditional setups. It excels in applications such as metabolomics, proteomics, and small molecule analysis. However, understanding the instrument's calibration and data interpretation processes can be intricate and requires careful consideration.
Sample Preparation Techniques
Sample preparation is a critical step in the mass spectrometry process. It impacts the accuracy, reproducibility, and sensitivity of the analytical results. Proper sample preparation techniques ensure that the desired analytes are preserved while removing potential interferences that may complicate the analysis.
The importance of sample preparation cannot be overstated. In many cases, the quality of data obtained is directly tied to how well the sample is prepared. If the matrix is complex, the extraction and purification methods become even more pivotal. Thus, optimizing these techniques influences the success of the entire analysis. This section highlights three primary methods for sample preparation.
Liquid Extraction Methods
Liquid extraction methods involve isolating compounds from liquid samples. They can be categorized into different techniques, such as liquid-liquid extraction or liquid-solid extraction. These methods are particularly useful when working with biological fluids, environmental samples, and chemical solutions.
Key benefits of liquid extraction include:
- Simplicity: Many methods are straightforward and easy to implement.
- Scalability: Techniques can be scaled up or down depending on the sample volume.
- Versatility: Applicable to various matrices, including biological and environmental samples.
However, careful consideration is necessary to select the most appropriate solvent and method for extraction. Improper choices can lead to loss of analytes or co-extraction of interferences that could affect the results.
Solid-Phase Extraction
Solid-phase extraction (SPE) is a widely used sample preparation method offering significant advantages for enhancing the quality of results. This technique utilizes a solid adsorbent to isolate target analytes from complex mixtures.
SPE is favored for several reasons:
- Higher Purity: It reduces the matrix effect often caused by impurities in the sample.
- Improved Sensitivity: By concentrating analytes, SPE increases the detection limits of the method.
- Automation: Many solid-phase extraction systems can be automated, allowing for greater throughput and efficiency.
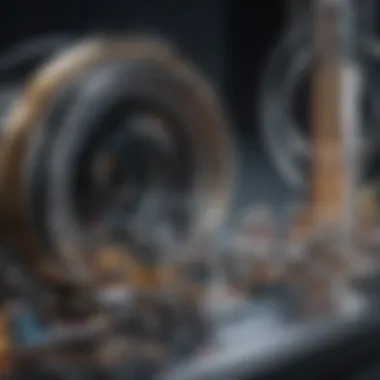
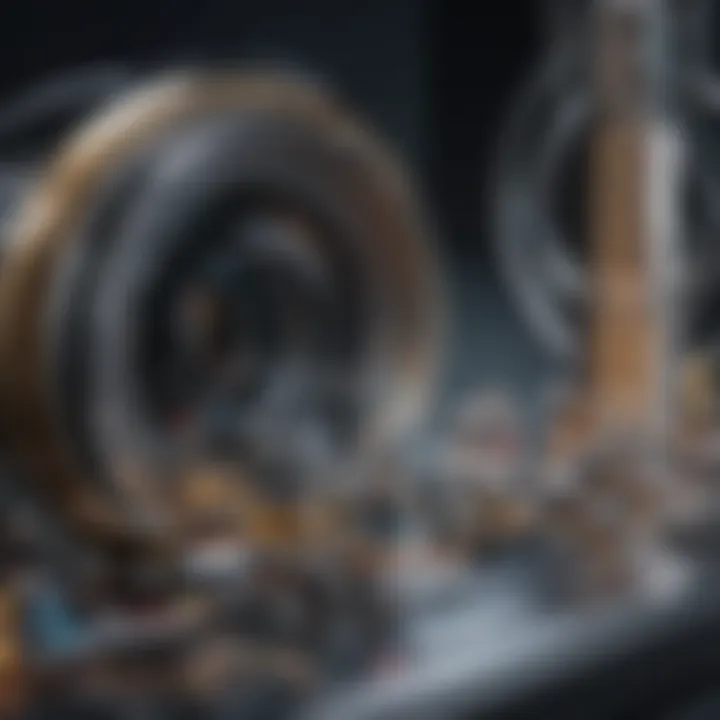
Despite its advantages, SPE also requires optimization of conditions, such as pH, ionic strength, and choice of adsorbent. Each of these factors can significantly influence extraction efficiency.
In-Situ Techniques
In-situ techniques refer to the preparation and analysis of samples as they occur in their natural state. This can provide valuable real-time data and minimize the risk of analyte degradation or loss.
These techniques can be beneficial in specific applications, including:
- Environmental Monitoring: Analyzing soil or water samples without prior manipulation.
- Biological Studies: Reducing changes in cellular components which might occur during traditional preparation.
The major consideration for in-situ techniques involves equipment setup and method reliability. Environmental conditions can affect results, making careful control and calibration necessary to ensure accurate measurements.
Overall, effective sample preparation techniques are vital in mass spectrometry. They ensure high-quality data by minimizing interference and maximizing analyte recovery. Advanced preparation methods continue to evolve, enhancing the capabilities of mass spectrometry in various scientific fields.
Applications of Mass Spectrometry
Mass spectrometry plays an essential role in various fields of research and industry, providing unparalleled analytical capabilities. Its applications extend from biology to environmental science, showcasing its versatility and importance. By accurately measuring the masses of ions, mass spectrometry enables the detection and quantification of a wide array of substances. This section delves into specific applications, underscoring how mass spectrometry addresses critical challenges and drives advancements in scientific inquiry.
Biological Samples Analysis
Mass spectrometry is invaluable in the analysis of biological samples, revolutionizing the way researchers study complex biological systems. It facilitates the identification of biomolecules such as proteins, lipids, and metabolites, enabling detailed insights into biological processes.
One significant advantage of mass spectrometry is its sensitivity, allowing for the detection of low-abundance biomolecules typical in biological samples. Techniques like tandem mass spectrometry enhance specificity, aiding in distinguishing similar molecules. Applications include:
- Metabolomics: Understanding metabolic processes by profiling metabolites in tissues.
- Proteomics: Profiling proteins to understand cellular functions.
- Pharmacokinetics: Studying the absorption, distribution, metabolism, and excretion of drugs.
In clinical settings, mass spectrometry aids in diagnosing diseases, analyzing bodily fluids, and monitoring treatments. The precision and rapidity of results make it a preferred choice in the medical field.
Environmental Monitoring
In environmental science, mass spectrometry plays a critical role in monitoring pollutants and assessing the quality of air, water, and soil. The ability to detect trace levels of contaminants is essential for environmental safety and public health. Mass spectrometry can identify various environmental samples, like:
- Water Samples: Detecting pesticides, heavy metals, and pharmaceuticals.
- Air Quality: Measuring volatile organic compounds and particulate matter.
- Soil Analysis: Identifying contaminants from agricultural practices.
The analytical capabilities of mass spectrometry enhance compliance with environmental regulations and contribute to the development of better environmental policies. This technology allows researchers to gain insights into pollution sources, effects on ecosystems, and effectiveness of remediation efforts.
Pharmaceutical Development
The pharmaceutical industry benefits significantly from mass spectrometry during drug development. It supports various stages, from discovery to production, ensuring that drug formulations are effective and safe for consumer use. Mass spectrometry provides the following benefits:
- Drug Discovery: Identifying active pharmaceutical ingredients and metabolites in drug candidates.
- Quality Control: Ensuring purity and consistency in drug manufacturing.
- Stability Testing: Assessing drug stability over time and under different environmental conditions.
Additionally, mass spectrometry assists in bioanalytical testing, determining drug levels in biological matrices, which is crucial for dose optimization and pharmacological studies.
Proteomics and Metabolomics
Proteomics and metabolomics are rapidly growing fields where mass spectrometry plays a central role. Proteomics focuses on the large-scale study of proteins, while metabolomics examines metabolites within cells, biofluids, tissues, or organisms.
Mass spectrometry allows for:
- Protein Characterization: Identifying and quantifying proteins within complex mixtures.
- Post-Translational Modifications (PTMs): Analyzing modifications that affect protein function and activity.
- Metabolite Profiling: Understanding metabolic changes in disease versus healthy states.
These applications extend into personalized medicine, where tailored treatments are developed based on individual biomolecular profiles.
Mass spectrometry is not just a tool; it is a gateway to understanding complex biological systems and environmental interactions.
Advancements in Mass Spectrometry Technology
The field of mass spectrometry is in a constant state of growth. Advancements in technology are crucial as they enhance the performance, sensitivity, and applicability of mass spectrometry in various fields. Understanding these advancements is key to grasping the future of analytical science. A closer look will reveal specific elements, benefits, and considerations around these advancements.
Innovations in Ionization Techniques
Recent innovations in ionization techniques have addressed limitations in sensitivity and versatility. Traditional ionization methods like Electrospray Ionization (ESI) and Matrix-Assisted Laser Desorption/Ionization (MALDI) provided foundational capabilities. However, new techniques such as Ambient Ionization have emerged. This allows analysis of samples in their natural states, bypassing the need for extensive sample preparation. Further enhancement is seen in techniques like Desorption Electrospray Ionization (DESI) that offers on-the-fly analysis and reduces contamination issues. These advancements push the boundaries of mass spectrometry while making it more accessible to various disciplines.
Improvements in Detector Sensitivity
High detector sensitivity remains a significant focus in mass spectrometry development. Enhanced sensitivity facilitates the detection of trace amounts of compounds that traditional instrumentation may miss. For instance, the arrival of more refined detectors like the Orbitrap and Ion Trap has increased the capacity to resolve complex mixtures. This improvement enables researchers to detect low-abundance analytes, crucial for fields like proteomics and metabolomics. Such innovations not only augment the capability of existing methods but also allow for more comprehensive analysis of samples.
Integration with Chromatography
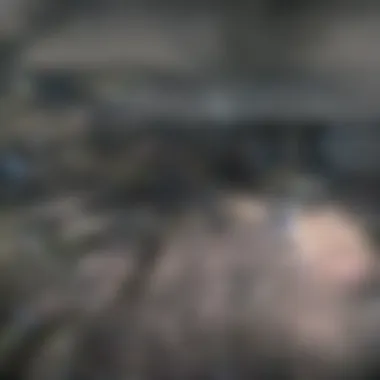
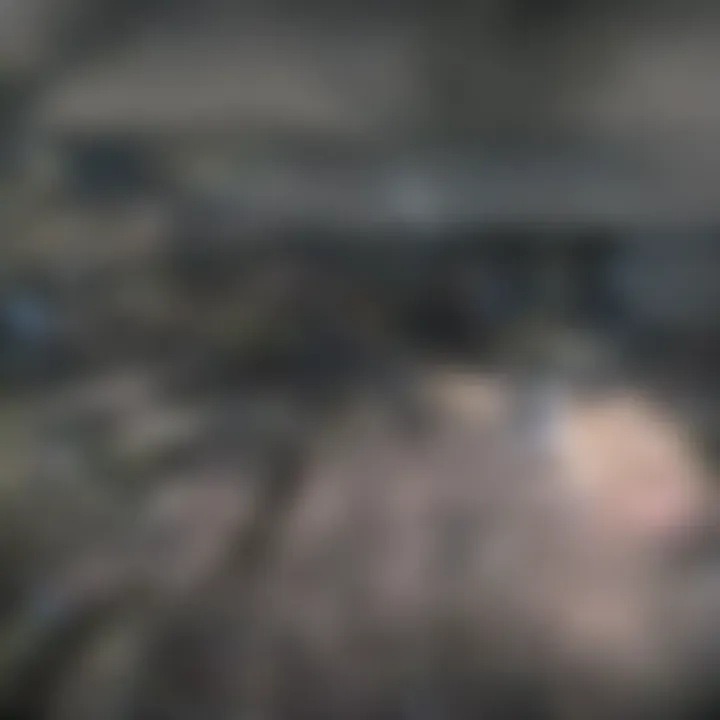
The integration of mass spectrometry with chromatography techniques marks a significant development in ensuring accurate and reliable results. Techniques such as Gas Chromatography-Mass Spectrometry (GC-MS) and Liquid Chromatography-Mass Spectrometry (LC-MS) become vital in separating chemical mixtures before analysis. This integration enhances the resolution and quantification of compounds. For instance, LC-MS plays a critical role in pharmaceutical development, identifying active components in drug formulations and ensuring quality control. As both fields continue to evolve, their collaboration makes mass spectrometry a powerful tool in both research and applied sciences.
"Innovations in mass spectrometry technology are reshaping scientific methodologies, making previously impossible analyses achievable."
Challenges Facing Mass Spectrometry
Mass spectrometry, despite its wide-ranging applications, faces several challenges that impact its effectiveness and accessibility. These challenges can hinder the accuracy of results and require researchers to develop solutions that ensure the reliability of analyses conducted using this technique. Understanding these obstacles is crucial for both new and experienced professionals in the field. Among the primary issues are data interpretation, high instrumentation costs, and the complexity of samples.
Data Interpretation Issues
Data interpretation is a major hurdle in mass spectrometry. The raw data generated from mass spectrometers may be dense and complex, making precise analysis a challenging endeavor. Each spectrum contains peaks that need to be correctly identified and quantified. Misinterpretation can lead to erroneous conclusions in research, which can have significant consequences, especially in fields such as clinical diagnostics or drug development.
Researchers must be trained to understand the nuances of the data. Furthermore, specialized software tools are often required to assist in data analysis. These tools use algorithms to process and interpret the results, but they are not foolproof. Many times, different programs may yield varying interpretations from the same data set, leading to inconsistency.
"One of the most significant challenges in mass spectrometry is ensuring accurate data interpretation, as errors can have far-reaching impacts."
Improving training and standardizing software tools could help alleviate some of these issues. Continuous development in data analysis methods remains essential.
Instrumentation Costs
The costs associated with mass spectrometry instruments can be a significant barrier to entry for many laboratories. High-end mass spectrometers, such as those from Agilent Technologies or Thermo Fisher Scientific, are often priced beyond the budgets of smaller laboratories or educational institutions. This can lead to a disparity in research capabilities, where only well-funded facilities have access to the necessary technology.
In addition to the initial investment in equipment, there are ongoing costs related to maintenance and operation. These costs include consumables, parts replacement, and staff training. The financial burden can deter potential users and slow down advancements in various scientific fields. For many, the price tag is a decisive factor when considering the adoption of mass spectrometry for their research needs.
Sample Complexity
Sample complexity presents another significant challenge in mass spectrometry. As scientific research evolves, samples are becoming increasingly complex, containing a wide variety of components. For instance, biological samples such as blood or tissue can have numerous metabolites, proteins, or lipids that can interfere with the analysis.
Dealing with this complexity often requires thorough sample preparation techniques, which can be time-consuming and demanding. Additionally, when certain components dominate the sample, it can hinder the detection of other, potentially more relevant, species. This necessitates the development of effective separation methods prior to mass spectrometry analysis.
To minimize issues arising from sample complexity, researchers must refine their methodologies and continue to optimize sample preparation techniques. Innovative extraction and purification processes can enhance the reliability of mass spectrometry outputs and ultimately lead to more accurate scientific conclusions.
Future Directions in Mass Spectrometry Research
The field of mass spectrometry is continuously evolving. Understanding future directions in this area is important for several reasons. First, the ongoing advancements drive improvements in analytical capabilities. New technologies and methodologies can enhance precision, sensitivity, and speed for researchers across multiple disciplines. Therefore, staying informed about emerging trends is crucial for anyone engaged in research, development, or education in the sciences.
Emerging Applications
Mass spectrometry has opened doors to numerous innovative applications. As the technology advances, its use in various fields is becoming more prevalent. Applications in proteomics and metabolomics allow for detailed insights into biological systems, which can lead to breakthroughs in biomedical research. The integration of mass spectrometry in clinical diagnostics is its new frontier. It can enable rapid analysis of metabolites and biomarkers for disease detection. Furthermore, food safety testing using mass spectrometry aids in identifying contaminants and ensuring public health.
Other emerging applications include advancements in material science research. Mass spectrometry assists in understanding the composition and properties of new materials. In environmental science, the technique is being used for monitoring pollutants. It has the potential to detect trace levels of hazardous substances in various samples, contributing to more effective environmental protection measures.
Trends in Automation
Automation is becoming a significant trend in mass spectrometry research. With increasing sample volumes, the demand for efficient workflows has also grown. Automated systems can reduce manual intervention, thus minimizing errors and increasing throughput. This trend emphasizes the integration of automated sample preparation techniques with mass spectrometry.
Recent developments in robotic systems allow for high-throughput analysis of samples. Such innovations promise to increase productivity. Moreover, software advancements are enabling better control over mass spectrometry operations. These tools can analyze data more quickly, aiding researchers in making decisions faster. Automating routine tasks creates more time for scientists to focus on complex data interpretation and innovative research projects.
Ethical Considerations
Venturing into future directions of mass spectrometry requires consideration of the ethical implications. The widespread use of mass spectrometry, especially in medical and environmental applications, raises serious ethical questions. Issues related to data privacy, especially in clinical settings, must be addressed. Ensuring the confidentiality of patient information during metabolomic studies is paramount.
Additionally, the environmental impact of chemicals analyzed by mass spectrometry should be considered. There must be strict regulations regarding the use of hazardous materials in sample processing. Researchers must adopt practices that prioritize sustainability and public safety. Moreover, the accessibility of mass spectrometry technology is essential. An equitable distribution of resources and training opportunities is crucial for advancing research without creating disparities in scientific progress.
"With the potential of mass spectrometry expanding, it is vital to approach its development with cautious consideration of ethical implications."
In summary, the future of mass spectrometry is promising. Emerging applications are leading to significant breakthroughs. Ongoing trends in automation are enhancing efficiency, while ethical considerations ensure responsible research practices. Keeping an eye on these directions will aid researchers and professionals in making informed decisions.
Culmination
The conclusion serves as a critical element in understanding the vast impact of mass spectrometry in contemporary research. It encapsulates the insights gained throughout the article, underlining the foundational principles, diverse applications, technological advancements, and the challenges addressed. Notably, it reiterates the significance of mass spectrometry as a pivotal analytical tool across various fields such as biology, chemistry, and environmental science, fostering a deeper appreciation of its role in scientific discovery.
Furthermore, this section provides an opportunity to synthesize the information discussed, allowing readers to grasp the overarching themes and key takeaways. Readers can reflect on how mass spectrometry not only contributes to existing knowledge but also sparks new questions and avenues for exploration.
Summary of Key Points
In summary, the article outlines essential aspects of mass spectrometry, including:
- Definitions and Importance: Mass spectrometry is defined as a sophisticated analytical technique crucial for identifying and quantifying substances.
- Core Principles: Detailed explanations of ionization methods, mass analyzers, and detection mechanisms that illustrate how mass spectrometers function.
- Types of Mass Spectrometry: Discussion on various techniques such as Quadrupole and Time-of-Flight, each with unique characteristics and applications.
- Sample Preparation: Methods like liquid extraction and solid-phase extraction are examined, emphasizing their role in obtaining accurate results.
- Applications: Diverse applications in biological analysis, environmental monitoring, and pharmaceutical development highlight the versatility of mass spectrometry.
- Technological Advancements: Cutting-edge developments in ionization and detector sensitivity that enhance the performance of mass spectrometry.
- Challenges: Considerations surrounding data interpretation, costs, and sample complexity that researchers must navigate.
- Future Directions: Emerging applications and trends, including automation and ethical aspects, hint at the evolving landscape of mass spectrometry.
Implications for Future Research
The implications for future research in mass spectrometry are profound. As scientific inquiries become more complex, the need for advanced analytical techniques will grow. Researchers are encouraged to:
- Explore Emerging Applications: The realm of mass spectrometry is ripe for discovery, particularly in untapped areas such as personalized medicine or environmental analysis.
- Embrace Automation: With the rise of automation, integrating modern technologies with mass spectrometry could streamline processes, improve efficiency, and reduce human error.
- Address Ethical Considerations: As mass spectrometry pushes boundaries, researchers must remain vigilant regarding ethical dilemmas associated with data use and privacy in biological analysis.
Ultimately, the future of mass spectrometry appears promising, fostering innovation and contributing to scientific knowledge on multiple fronts.