Exploring Membrane Biofilm Reactors: Principles & Applications
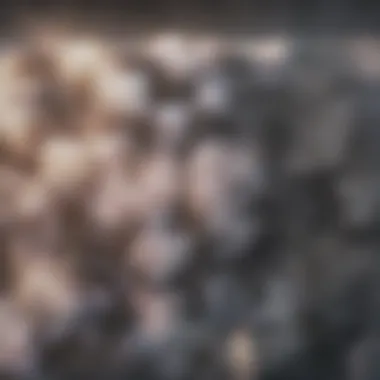
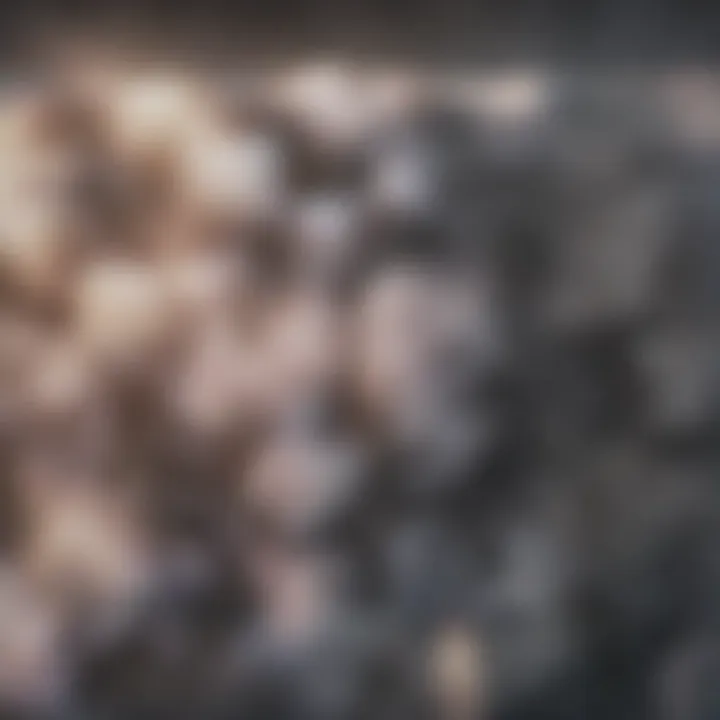
Intro
Membrane biofilm reactors (MBRs) represent a transformative approach in wastewater treatment. These systems integrate biological processes with membrane filtration, allowing them to simultaneously achieve high levels of pollutant removal and produce treated water with superior quality. The appeal of MBRs lies in their ability to handle high organic loads and their flexibility in design and operation.
This article explores the operational principles of MBRs, examining their configurations and the various applications across industries. The discussion delves into the biological mechanisms that facilitate efficient treatment and the physicochemical interactions occurring within the reactors. Additionally, several factors influencing the performance of MBRs are considered, alongside advancements in membrane technology that have enhanced their effectiveness.
As water scarcity and pollution become increasingly pressing global issues, MBRs offer sustainable solutions. By analyzing current challenges and potential future directions, this article provides valuable insights into the role of MBRs in addressing these critical environmental concerns.
Preamble to Membrane Biofilm Reactors
Membrane biofilm reactors (MBRs) represent a significant innovation in wastewater treatment and resource recovery. Their unique integration of biological processes with membrane filtration allows for improved efficiency and effectiveness in treating various forms of wastewater. Understanding MBRs provides insights into their operational principles, configurations, and applications. Such knowledge is essential, especially in the context of global water scarcity and pollution.
Definition and Overview
Membrane biofilm reactors are advanced systems that combine biological treatment methods with membrane technology. They utilize a biofilm, a layer of microorganisms that form on the membrane surface, to break down organic matter. The process increases treatment capacity and reduces the footprint compared to traditional treatment systems. MBRs are capable of producing high-quality effluent, making them suitable for water reuse applications.
The membranes used in MBRs are semi-permeable barriers that allow water and small molecules to pass through while rejecting larger particles, including suspended solids and pathogens. This separation not only enhances effluent quality but also facilitates the concentration and removal of nutrients and other contaminants.
Historical Context
The concept of using membranes for wastewater treatment dates back several decades. However, the integration of biofilm technology into membrane processes has evolved more recently. In the 1970s and 1980s, research focused on membrane filtration alone, but the limitations in treatment performance led to the exploration of biological methods alongside.
By the early 1990s, this research culminated in the development of the first membrane bioreactors that operated with biological aerated filters. Technologies improved, and now MBRs are a relevant topic in both academic and industrial circles. The rise of MBRs correlates with the increasing awareness of the need for sustainable water management practices, amid growing environmental concerns.
Establishing a comprehensive understanding of MBRs provides an essential foundation for evaluating their current application in wastewater treatment and potential future developments.
Operating Principles of MBRs
Membrane biofilm reactors (MBRs) are a blend of biological processes and advanced membrane filtration technologies. This combination allows for efficient wastewater treatment, making it a focal point in many discussions about sustainable water management. Understanding how MBRs function is crucial to appreciating their applications and the potential challenges they may face.
Biological Processes
The biological processes are key to the performance of MBRs. They include the actions of microbial communities, biofilm formation, and nutrient removal strategies. Each element contributes to the overall effectiveness of these reactors in treating wastewater.
Microbial Communities
Microbial communities play a pivotal role in MBRs. They are made up of various microorganisms that work together to degrade organic matter and pollutants. The diversity of these communities is vital for ensuring that a wide range of contaminants can be processed efficiently. One key characteristic is their adaptability to different environmental conditions. This makes them a beneficial choice for MBRs as they can thrive under various operational scenarios. However, the unique feature of these communities is their susceptibility to sudden changes in the influent quality. This disadvantage can lead to instability in treatment performance, highlighting the importance of regular monitoring.
Biofilm Formation
Biofilm formation is another essential aspect of MBRs. It involves microorganisms attaching to each other and to the membrane surface, creating a protective layer. This process enhances the degradation of pollutants as the biofilm retains a higher concentration of active microorganisms. Its key characteristic is that it provides a stable environment for microbial activities. This is beneficial in maintaining consistent treatment efficiencies. A unique feature of biofilms is their resistance to shear forces, allowing them to remain intact even with high flow rates. However, excessive biofilm can lead to fouling, which poses a significant challenge to MBR operation.
Nutrient Removal
Nutrient removal in MBRs targets the reduction of nitrogen and phosphorus compounds from wastewater. This process is crucial for preventing the eutrophication of receiving water bodies. The significant characteristic of nutrient removal is its ability to achieve high removal efficiencies. This capability makes MBRs a popular choice for managing municipal and industrial effluents. The unique feature of this process is its integration with both biological and chemical methods, which enhances overall performance. However, cost implications of nutrient removal strategies can be a disadvantage, necessitating effective cost-management practices.
Membrane Filtration
Membrane filtration is the backbone of MBR technology. It separates treated water from the mixed liquor and plays a vital role in determining the quality of the permeate. Understanding different membrane types, operational modes, and permeate quality is critical for optimizing MBR performance.
Membrane Types
There are various membrane types used in MBRs, including microfiltration and ultrafiltration membranes. Each type has different pore sizes and applications. Microfiltration membranes are effective for larger particulate removal, while ultrafiltration membranes address smaller dissolved molecules. A key characteristic of these membranes is their differing filtration capacities, which directly affect treatment efficiency. This variability makes careful selection essential for MBR design. A unique feature of newer membrane materials is their enhanced fouling resistance, but they may come at a higher cost, presenting a trade-off for operators to consider.
Operation Modes
The operation modes of MBRs can greatly influence their performance. Common modes include batch and continuous flow systems. Each operation mode provides specific advantages based on the treatment goals. For instance, continuous flow operation allows for steady-state conditions, promoting stable treatment performance. Its key characteristic is efficiency in handling varying inflow rates. However, batch operation can offer flexibility for fluctuating wastewater qualities, which may be beneficial in some scenarios. The unique advantage of continuous modes is their simplicity in operation, but they may require more complex control systems.
Permeate Quality
Permeate quality is crucial in determining the overall success of MBRs. High-quality permeate can significantly impact water reuse applications. The key characteristic of this quality assessment is its influence on the acceptability of treated water for various uses. MBRs are known for producing high-quality effluent with low concentrations of suspended solids and pathogens. The unique feature of this process is the ability to meet stringent regulatory requirements. However, monitoring and maintaining permeate quality can be challenging, necessitating robust operational practices.
Overall, the operating principles of MBRs are fundamental to realizing their potential in sustainable wastewater treatment. Understanding each aspect provides insight into how these systems can be effectively managed and improved.
Configurations of Membrane Biofilm Reactors
The configuration of membrane biofilm reactors (MBRs) is a crucial aspect that can significantly influence their performance and application in various areas. Understanding these configurations allows researchers and practitioners to optimize system designs for specific operational objectives. MBR configurations chiefly determine the interactions between the biofilm, the membrane, and the influent, directly impacting efficiency and practicality.
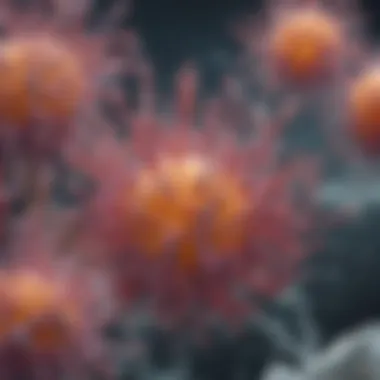
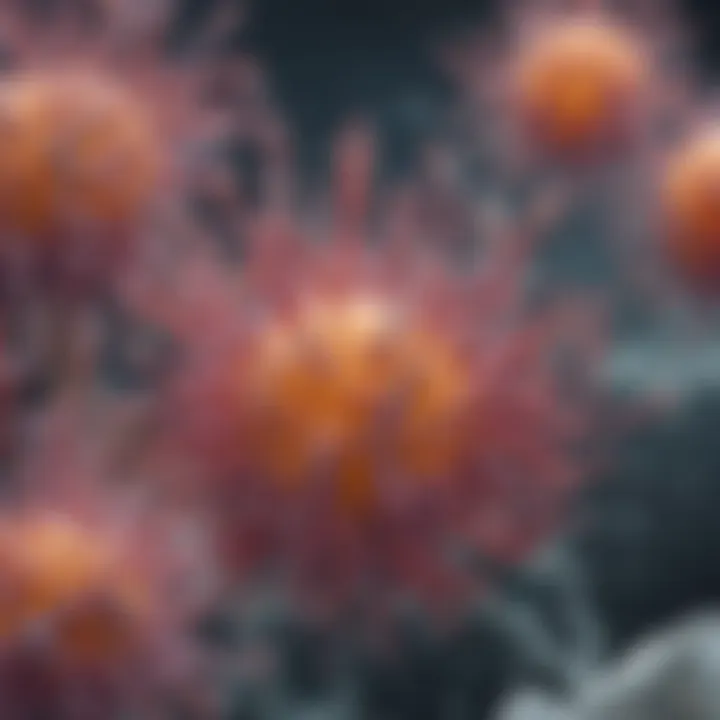
Submerged vs. External MBRs
Submerged membrane biofilm reactors integrate membrane filtration within the reactor itself. In this setup, membranes are submerged in the mixed liquor of the bioreactor. This configuration promotes high biomass retention, which is beneficial for efficient biological treatment. The biofilm grows on the membrane surface, allowing for direct processing of the effluent. One notable advantage of submerged systems is their compact design, which minimizes land use and is often easier to manage when compared to external systems.
However, submerged MBRs face challenges, particularly with membrane fouling. The constant contact between the biomass and the membrane surface can lead to the accumulation of organic and inorganic materials, necessitating regular cleaning and maintenance. This fouling can reduce overall filtration efficiency, posing a challenge for long-term operation.
In contrast, external membrane biofilm reactors position the membrane outside the bioreactor. Here, the mixed liquor passes to an external filtration unit where permeate is drawn through the membrane. This setup often features lower fouling rates because the filtration occurs away from the biomass environment. Consequently, it can simplify cleaning processes and extend the membrane's lifespan.
However, external systems may require additional energy for pumping, and they can occupy more physical space compared to submerged designs. The selection between submerged and external systems thus relies on analyzing the specific operational requirements and constraints of the treatment process.
Hybrid Systems
Hybrid systems represent a combination of different MBR configurations. These may merge the benefits of both submerged and external setups, tailoring the reactor design to specific wastewater characteristics and treatment goals. Hybrid systems frequently employ advanced filtration technologies and may incorporate additional treatment components, such as anaerobic reactors or advanced oxidation processes, to enhance overall performance.
The flexibility of hybrid systems allows for adjustable operational parameters, making them suitable for varied applications from municipal wastewater treatment to specialized industrial effluent management. They can also improve resource recovery, capturing nutrients and biogas more efficiently than traditional approaches.
In essence, hybrid systems can enhance treatment efficiency, but they may involve higher capital and operational costs. As researchers continue to explore innovative configurations, these systems present exciting possibilities for improving MBR technology and addressing water quality challenges.
"Innovative MBR configurations can bring significant operational benefits and open new avenues for wastewater treatment applications."
Understanding the different configurations of MBRs enables stakeholders to make informed decisions regarding system choice and design. By aligning configuration types with specific treatment goals, researchers and practitioners can enhance the sustainability and effectiveness of membrane biofilm reactors in addressing pressing water management issues.
Applications of Membrane Biofilm Reactors
The applications of membrane biofilm reactors (MBRs) are diverse and play a critical role in modern waste management and resource recovery strategies. These systems combine biological treatment with membrane filtration, making them particularly effective in treating polluted water and reclaiming valuable resources. Their capacity to improve effluent quality while addressing environmental concerns makes them a significant focus within this article.
Wastewater Treatment
Municipal Wastewater Management
Municipal wastewater management involves the treatment of sewage and wastewater generated by residential and commercial establishments. MBRs are increasingly recognized in this sector as they provide a more compact solution compared to traditional processes. The key characteristic of municipal wastewater management using MBRs is their ability to produce high-quality effluent that meets stringent discharge standards. This makes them a popular choice for urban settings where space is limited.
One unique feature of municipal wastewater management with MBRs is the simultaneous removal of organic matter and pathogens. This dual role enhances the treatment efficiency, resulting in clean water that can be directly reused for irrigation and other non-potable applications.
Advantages of using MBRs in this context include reduced footprint and increased treatment efficiency. However, the disadvantages may involve higher operational costs and membrane fouling challenges that necessitate regular maintenance and management.
Industrial Effluents
Industrial effluents represent another significant application of MBR technology. These effluents often contain complex and harmful substances that require advanced treatment approaches. MBRs can effectively handle such variations in wastewater, making them a suitable choice for diverse industries, including pharmaceuticals and food processing.
The key characteristic of using MBRs for industrial effluents is their adaptability. They can be designed to manage fluctuating flow rates and varying contaminant loads, which are common in industrial operations.
A unique feature of this application is the capability of MBRs to recover water and valuable by-products, contributing to sustainability goals within industries. Among the advantages are improved removal efficiencies for nutrients and organic pollutants. Yet, the disadvantages might include the necessity for complex pretreatment procedures to tackle high concentrations of specific pollutants.
Resource Recovery
Nutrient Recovery
Nutrient recovery from wastewater is gaining interest as a vital application in MBR technology. This practice aims to reclaim essential nutrients like nitrogen and phosphorus from waste streams. The key characteristic of nutrient recovery through MBRs lies in their ability to concentrate these nutrients, which can then be reused in agriculture as fertilizers.
The unique feature of this process is the dual benefit of reducing environmental pollution while providing a sustainable source for agricultural needs. Advantages include closed-loop nutrient systems that help in minimizing runoff and improving soil health. However, challenges may arise, such as the cost of processing and the technology’s maturity.
Biogas Production
Another compelling aspect of resource recovery in MBRs is biogas production. During the anaerobic treatment of wastewater, methane is generated, serving as a renewable energy source. The key characteristic of this process involves harnessing waste material to generate energy, promoting sustainability.
The unique feature of biogas production within MBRs is the potential for integration with energy generation systems. Advantages include the reduction of greenhouse gas emissions, while disadvantages can include operational complexities and the need for additional operational monitoring to maintain efficiency.
Drinking Water Reuse
Drinking water reuse is an essential application for MBRs, especially in regions facing water scarcity. MBR technology enables the treatment of wastewater so that it meets potable water standards. The importance of this application lies in its role in enhancing water security and providing an alternative water source.
The key characteristic of drinking water reuse with MBRs is the advanced filtration systems that remove pathogens and contaminants from treated effluents. This makes it a beneficial choice for communities seeking to augment their water supply sustainably.
In summary, the applications of membrane biofilm reactors span various sectors, including wastewater treatment and resource recovery. MBRs not only contribute to environmental protection efforts but also advance the circular economy by reclaiming valuable resources from waste. These applications highlight the significance of MBRs in tackling global water challenges.
Factors Influencing MBR Performance
Understanding the factors that influence the performance of membrane biofilm reactors (MBRs) is crucial for optimizing their operation and ensuring efficient treatment processes. MBRs are affected by various elements including fouling, operational conditions, and technological advancements. Addressing these factors can lead to enhanced efficiency and sustainability in wastewater treatment applications.
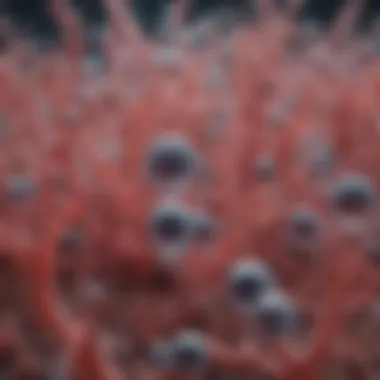
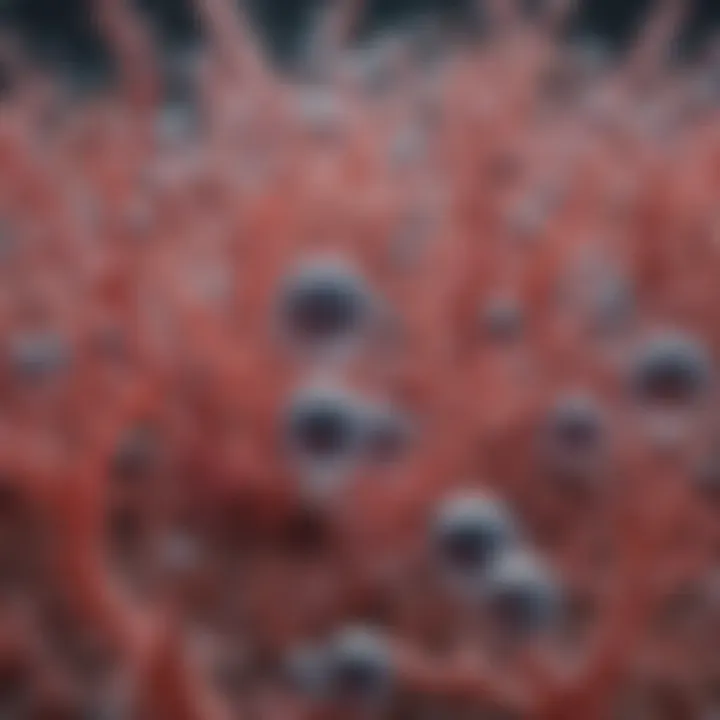
Membrane Fouling
Fouling Mechanisms
Fouling mechanisms refer to the processes that lead to a decline in membrane performance and efficiency. The primary fouling mechanisms in MBRs include:
- Biological fouling: This occurs when microbial cells and extracellular polymeric substances accumulate on the membrane surface. The growth of a biofilm can create a resistant layer that impacts filtration.
- Organic fouling: Organic materials, such as proteins and humics, can adsorb onto the membrane, causing obstruction of pores.
- Inorganic fouling: Inorganic compounds, including salts and minerals, may precipitate and form scales that impede flow.
These mechanisms are significant as they directly affect permeate quality and operational costs, making their understanding essential in this article. Effective management of fouling leads to longer membrane life and reduced maintenance.
Mitigation Strategies
Mitigation strategies focus on reducing or preventing fouling to maintain optimal MBR performance. Key strategies include:
- Physical cleaning: Techniques such as backwashing or air scouring can dislodge fouling materials from the membrane surface.
- Chemical cleaning: Regular chemical cleaning regimens using acids or bases can effectively remove accumulated foulants.
- Optimizing operating conditions: Adjusting flow rates and hydraulic retention times can minimize fouling by promoting better flow dynamics.
Utilizing these strategies can lead to significant performance improvements, resulting in lower operational costs and prolonged membrane lifespan. Each method has its unique advantages and disadvantages, which should be carefully considered based on the specific context of the treatment process.
Operational Conditions
Hydraulic Retention Time
Hydraulic retention time (HRT) determines the duration that wastewater remains in the reactor. Greater HRT facilitates:
- Improved microbial growth and activity
- Enhanced nutrient removal capacity
- Increased biopolymer production, which can help mitigate fouling
An optimal HRT balances sufficient treatment time without unnecessary volumetric excess, which can elevate operating costs. For MBRs, a well-optimized HRT enhances the overall efficiency of treatment processes.
Temperature Effects
Temperature significantly affects biological activity within an MBR. Increased temperatures generally lead to:
- Accelerated microbial metabolism
- Changes in the solubility of various compounds, influencing nutrient dynamics
However, excessive temperatures can also disrupt microbial communities and increase membrane fouling rates. Therefore, understanding the specific temperature-range effects is critical for maximizing MBR performance in various environments.
Technological Advances in MBRs
Technological advances in membrane biofilm reactors (MBRs) play a crucial role in enhancing the overall efficiency and effectiveness of wastewater treatment systems. This section details recent innovations that help optimize MBR operations, improve performance metrics, and expand their applications. By understanding these advancements, researchers and practitioners can make informed decisions that contribute to sustainable water management solutions.
Novel Membrane Materials
Polymeric Innovations
Polymeric membranes have gained attention due to their flexibility and relatively low production costs. These membranes are often composed of materials like polyvinylidene fluoride (PVDF) and polyethersulfone. The key characteristic of polymeric innovations lies in their excellent permeability, allowing for higher flux rates in MBR systems.
Their adaptability to various operating conditions significantly contributes to advancements in MBR technology. One of the unique features of these membranes is their ability to be modified with functional groups. This modification can enhance antifouling properties, which is advantageous in reducing maintenance cycles and improving longevity. However, they often suffer from thermal and chemical stability, which might limit their use under extreme conditions.
Inorganic Membranes
In contrast, inorganic membranes offer exceptional stability and resistance to fouling. Common materials include ceramic and metal oxides. A key characteristic of inorganic membranes is their robustness, which allows them to withstand harsh operational environments such as high temperatures or aggressive chemical conditions. The high structural integrity of these membranes makes them a reliable choice for permanent installations.
A unique feature of inorganic membranes is their enhanced resistance to fouling and clogging. This characteristic can lead to extended operational periods without the need for frequent cleaning. However, the fragility during handling and the higher manufacturing costs can pose significant challenges for scaling up their production and use in widespread applications.
Hybrid Treatment Technologies
Hybrid treatment technologies comprise the integration of MBRs with other treatment processes. This combination can optimize resource recovery while reducing operational costs. The most prominent hybrid configurations include coupling MBRs with biological processes or advanced oxidation processes.
Economic Aspects of MBR Implementation
The economic aspects of membrane biofilm reactors (MBRs) play a vital role in their adoption and longevity in various applications, particularly in wastewater treatment and resource recovery. Understanding the costs involved, alongside available funding and incentives, provides insights into the feasibility and sustainability of implementing MBR systems. Evaluating these economic factors is essential for stakeholders considering investments in this technology.
Cost Analysis
Analyzing the costs associated with MBR systems is imperative for decision-makers. The initial investment in MBR technology can be higher than that of conventional treatment methods. These costs include membrane procurement, installation, and the expenses associated with maintaining operational efficiency. Notably, the absence of a significant land requirement and a smaller footprint can offset some of these costs. Moreover, operational costs involve energy consumption, labor, and maintenance.
Several studies emphasize the long-term cost benefits of MBRs. For instance, reduced sludge production and improved effluent quality can lead to lower downstream processing costs. In some municipalities, the savings achieved through resource recovery have proven considerable. When evaluating costs, stakeholders should consider life-cycle assessments to understand long-term implications better.
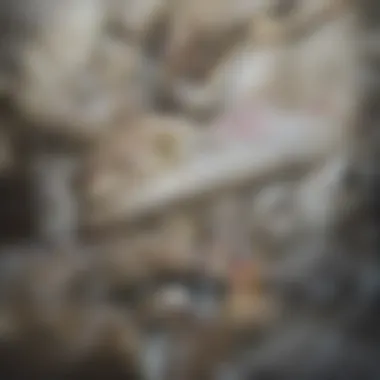
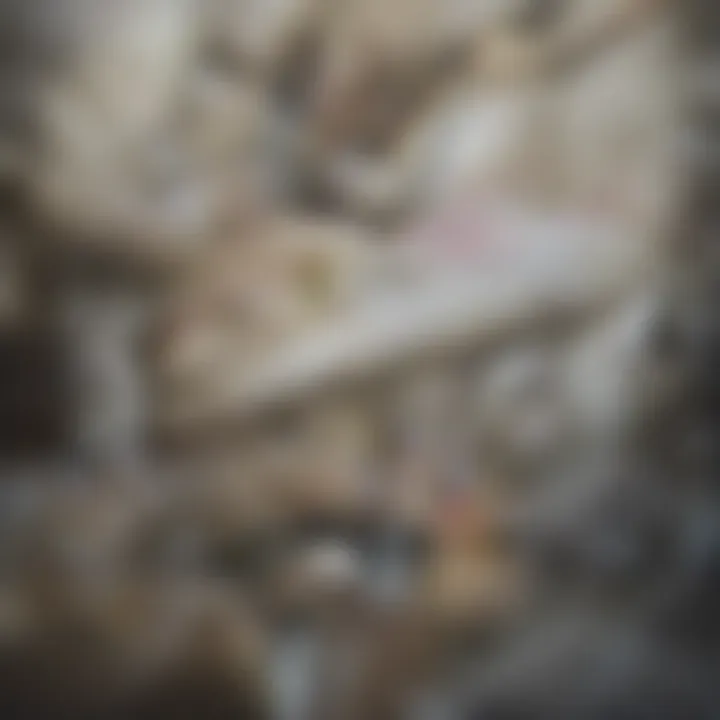
"Investing in MBRs might seem costly at the outset, yet the return on investment becomes clearer with enhanced operational efficiencies and resource recovery capabilities."
Funding and Incentives
Funding opportunities and incentives can significantly enhance the economic viability of MBRs. Various government programs and grants aim to promote sustainable water management and environmental protection initiatives. Engaging with local and national agencies can help identify potential funding sources.
In addition to grants, tax incentives are available in certain regions. These incentives can alleviate some financial burden associated with initial investments. Moreover, public-private partnerships have emerged as a viable way to share resources, expertise, and risks. They encourage private sector involvement in developing and supporting MBR projects.
In summary, the economic aspects of MBR implementation involve careful analysis of costs and potential funding sources. Understanding the cost dynamics and available incentives allows stakeholders to make informed decisions that can lead to successful MBR operations.
Challenges Facing Membrane Biofilm Reactors
Membrane biofilm reactors (MBRs) present a cutting-edge solution for wastewater treatment. However, their implementation is not without significant challenges. Understanding these challenges is crucial to enhancing MBR technology's effectiveness. The core aspects to consider include scalability issues and regulatory considerations, both of which critically shape the adoption and success of MBR systems in various contexts.
Scalability Issues
One major challenge with MBRs is scalability. As a treatment technology, MBRs often have high operational costs and complicated designs that may not easily translate from pilot projects to full-scale operations. It becomes difficult to determine if an MBR system at a smaller scale will be effective when scaled up. This is mainly because equipment performance can vary significantly with changes in size and operational conditions.
In addition, operational parameters such as hydraulic retention time and influent characteristics may vary between small and large systems. This variability introduces uncertainty in predicting how systems will respond under different loads and conditions.
Moreover, there is the concern of maintaining consistent biofilm quality and thickness as the system scales. At larger scales, maintaining uniform membrane fouling mitigation also becomes complex, leading to potential inefficiencies. Without careful management of these factors, MBRs may not perform optimally, which can deter municipalities or industries from investing in this technology.
"The transition from pilot to full-scale MBRs often presents unforeseen challenges that must be addressed for successful implementation."
Regulatory Considerations
Regulatory considerations also play a key role in the challenges faced by MBR technology. Despite their potential for efficient treatment, the regulatory framework in many regions is not fully equipped to handle the unique aspects of MBR systems. For instance, most existing regulations are often designed around traditional wastewater treatment methods, which may not adequately capture the benefits or concerns specific to MBRs.
The establishment of new standards for water quality post-treatment is necessary. MBR systems can produce high-quality permeate, but without clear guidelines, there may be hesitance from regulatory bodies to recognize or endorse these advancements. Furthermore, the complexity of MBR systems may necessitate specialized knowledge among regulators to properly assess their compliance and operational performance.
This regulatory gap may also hinder funding opportunities for MBR projects. If there are uncertainties regarding compliance or efficacy, financial backers may look for less complex technologies to support.
In summary, understanding and addressing scalability and regulatory issues are essential steps towards realizing the full potential of membrane biofilm reactors in the realm of wastewater treatment.
Future Directions in MBR Research
Membrane biofilm reactors (MBRs) represent a crucial innovation in wastewater treatment technologies. As global water issues become more pronounced, the importance of researching the future directions in MBRs cannot be understated. Continued research efforts focus on two primary areas: emerging research and the integration with circular economy concepts.
Emerging Research Areas
Sustainable Practices
Sustainable practices in MBR research focus on optimizing processes while minimizing environmental impacts. The key characteristic of sustainable practices is their ability to enhance efficiency in resource utilization and waste reduction. This is beneficial in the context of MBRs, where achieving high treatment standards while lowering operational costs is vital. Sustainable practices include the use of renewable energy sources and waste-to-energy conversion, which not only support energy efficiency but also contribute to lower carbon emissions.
A unique aspect of these practices is their adaptability to local contexts. This can lead to customized solutions that better suit specific environmental conditions and community needs. The advantages include reducing the overall footprint of treatment facilities, improving public acceptance, and potentially providing economic benefits through energy recovery. However, challenges may arise, such as the initial costs of adopting these green technologies and the complexity of operational modifications.
Advanced Monitoring Techniques
Advanced monitoring techniques are becoming increasingly important in MBR systems. These techniques encompass the use of real-time sensors and data analytics to assess system performance continuously. The key characteristic of advanced monitoring is its precision in detecting changes in operational parameters, allowing for timely interventions.
These techniques are advantageous because they improve the reliability and efficiency of MBRs. For example, they can help to identify issues related to membrane fouling or variations in influent quality before they escalate into larger problems. A unique feature is the integration of artificial intelligence, which allows for predictive maintenance and operational optimization. However, the disadvantages may include the need for substantial investment in technology and training, as well as the potential for data overload, which could complicate decision-making processes.
Integration with Circular Economy Concepts
Integration with circular economy concepts is crucial for the future of MBR research. The circular economy aims to close the loop in resource use, making systems more resource-efficient. In this context, MBRs can act as central players in treating wastewater while recovering valuable resources like nutrients and water.
The emphasis on maintaining and recovering resources means that MBRs need to transition from being solely treatment systems to resource recovery technologies. This shift encourages the design of MBR facilities that not only filter wastewater but also generate usable bioproducts. Understanding the interplay of biological and technical systems within a circular economy framework can lead to more innovative solutions. However, challenges reside in the need for regulatory frameworks that support such integrations and in providing infrastructures that facilitate resource recovery processes.
In summary, advancing MBR research in sustainable practices, monitoring techniques, and circular economy integration is essential. A focus on these aspects promotes more efficient wastewater treatment while addressing environmental challenges, thus paving the way for a more sustainable future.
Continued exploration in these areas will enhance the viability of MBRs as a solution to global water scarcity and pollution issues.
Closure
In this article, we have examined the significance of membrane biofilm reactors (MBRs) in wastewater treatment and resource recovery. This conclusion emphasizes the critical aspects discussed throughout the piece and the implications for future research and application.
MBRs represent a vital technology in the field of waste management. They combine biological and membrane filtration processes, optimizing efficiency in treating wastewater. Hence, understanding their principles and applications informs practitioners and researchers about the most effective ways to integrate them into current systems.
The benefits of MBRs include their ability to achieve high-quality effluent while minimizing footprint. These qualities make them appealing for both municipal and industrial applications. Moreover, MBRs facilitate resource recovery, particularly nutrients and biogas, aligning with global sustainability goals.
Nevertheless, challenges related to scalability, membrane fouling, and regulatory requirements remain. Addressing these concerns is paramount for broader implementation. Specifically, future research must focus on developing more resilient membranes and enhancing operational protocols to mitigate fouling and maintain performance efficiency.
Additionally, the integration of MBRs into circular economy concepts offers a path forward that aligns technology with sustainable practices. By fostering innovative approaches, industries can harness the full potential of MBRs, paving the way for a more sustainable future.
Ultimately, this discourse on membrane biofilm reactors illustrates their importance in combating water scarcity and pollution. As we continue to navigate these global challenges, embracing and advancing MBR technology will be crucial for ensuring a clean and sustainable environment.