Microbial Detection Methods: A Comprehensive Overview
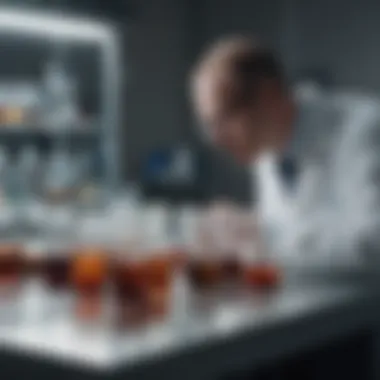
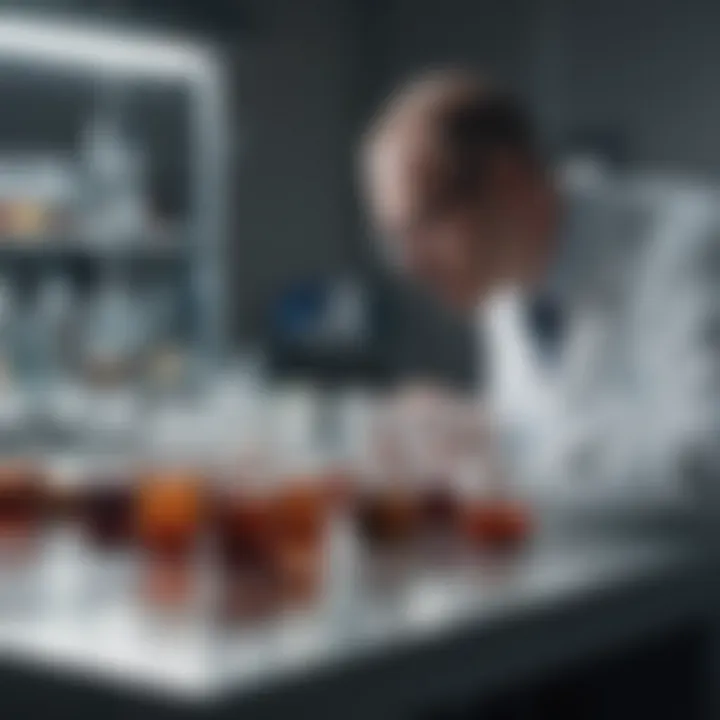
Intro
Microbial detection plays a crucial role in various fields, influencing public health, food safety, and environmental monitoring. These methods help scientists and practitioners identify microorganisms that may pose risks to human health or the ecosystem. The evolution of technologies available for microbial detection significantly enhances our understanding of microbial communities in different environments. It also informs policy-makers and safety regulators on how to manage risks effectively.
In delving into microbial detection methods, we uncover a tapestry of approaches, each with distinct benefits and drawbacks. From traditional culturing techniques to the latest molecular methods, every technique offers unique insights while presenting certain challenges. The landscape is indeed diversified, providing a broad spectrum of options that can be tailored to specific requirements.
This exploration does not only summarize existing methods but also sets the stage for future advancements. It emphasizes the importance of adapted and innovative detection strategies, particularly as new microbial strains emerge and regulatory needs shift.
The discussion will address how these detection methods operate, their applications, and their significance in safeguarding health and the environment. Let’s embark on this comprehensive journey to understand how microbial detection reshapes our interaction with the unseen life forms around us.
Preamble to Microbial Detection
In the complex world of life sciences, microbial detection emerges as a cornerstone for understanding both pathogenic and beneficial microorganisms. This process, which encompasses a collection of methods, allows researchers and professionals to identify and quantify microbial life in a variety of settings. From agriculture to healthcare, the importance of microbial detection cannot be overstated, as it plays a critical role in ensuring public health safety, food quality, and environmental sustainability.
Importance of Microbial Detection
The significance of microbial detection extends far beyond mere identification of microbes. For one, detecting pathogens in clinical samples can help flag potential outbreaks before they spiral out of control. Early and accurate detection can mean the difference between containment and widespread public health crises. In food safety, knowing what types of bacteria or molds are present in food products can guide quality control measures and prevent foodborne illnesses.
Moreover, microbial detection supports biodiversity assessments in ecosystem studies, where understanding microbial community composition can shed light on environmental health. For businesses involved in fermentation or bioprocessing, consistent monitoring can drastically influence product quality and safety. This intricate dance of detection ultimately demands tools that not only are reliable but also efficient and adaptable, providing a comprehensive framework for analysis.
Historical Perspective
Looking back at the journey of microbial detection reveals a fascinating evolution. In the early days, detection hinged on rudimentary methods, often relying solely on observable traits of microorganisms, like colony morphology or staining properties. For example, the use of Koch’s postulates laid the groundwork for associating specific microbes with diseases. As the field advanced, techniques like microscopy provided a clearer view into the microbial world, illuminating details that would have otherwise remained hidden.
The 20th century brought on the rise of culturing methods, which paved the way for isolating and studying individual microbial species more thoroughly. Enrichment cultures allowed researchers to coax out even the most elusive organisms from complex mixtures. This collective knowledge was essential in developing immunological techniques such as serology, where antibodies are employed to detect specific pathogens in samples.
Today, advances in molecular techniques have revolutionized the field, allowing for rapid detection and a deeper understanding of microbial genetics and interactions. It’s interesting to note how what started with simple observations has morphed into a multi-faceted discipline, continuously adapting to meet the challenges of modern science.
"The history of microbial detection is a story of innovation, skillfully blending art with science to peel back the layers of the microscopic kingdom."
The exploration of microbial detection methods reveals not just the methods themselves, but a deeper understanding of our world, making it a vital area of study for students, researchers, and professionals alike. The pace of change suggests that each new breakthrough will add another layer to this already intricate tapestry.
Traditional Microbial Detection Methods
Traditional microbial detection methods hold a crucial place in the broader landscape of identifying and analyzing microorganisms. These methods offer fundamental insights into microbial presence and behavior, which is essential in various fields like medicine, food safety, and environmental science. Their significance lies in their accessibility and the foundational understanding they provide, paving the way for later, more advanced techniques. Moreover, many of these methods have been honed over decades, making them reliable and well-understood in terms of their benefits and limitations.
Culturing Techniques
Culturing techniques are at the core of traditional microbial detection methods. They rely on growing microorganisms in controlled environments to study their characteristics, behaviors, and interactions.
Agar Plate Techniques
Agar plate techniques are commonly used for isolating and identifying microorganisms. This method involves spreading a sample across a solid medium, allowing colonies to form. A notable strength of agar plate techniques is their capacity to support a wide variety of microbial species with minimal resources. This approach is especially beneficial for its simplicity and low cost, making it accessible to laboratories worldwide.
One unique feature of agar plates is the ability to incorporate specific nutrients and indicators directly into the medium. These modifications can help distinguish between various microorganisms based on their growth patterns and biochemical properties. However, these techniques also display notable constraints; for instance, not all microorganisms grow in culture, which may lead to underestimating microbial diversity. Moreover, the process can be time-consuming as it typically takes several days to observe growth.
Liquid Cultures
Liquid cultures present another dimension of culturing techniques. They involve growing microorganisms in a broth, allowing for the evaluation of growth rates and metabolic activity over time. The key characteristic of liquid cultures is their ability to provide a more uniform growth environment compared to solid media, which aids in the assessment of fast-growing organisms.
Moreover, liquid culture methods can be easily scaled up or scaled down depending on the needs of a study, making them quite versatile. A significant advantage is that they can be used for quantitative assays, allowing for precise measurements of microbial concentration.
However, like agar plate techniques, liquid cultures have disadvantages. They may not support the growth of certain anaerobic microorganisms without specific conditions in place. Furthermore, the potential for contamination is higher in liquid cultures, and it can sometimes be challenging to isolate individual colonies for further study.
Microscopic Examination
Microscopic examination techniques are vital for visualizing microorganisms and confirming their characteristics, often serving as a complementary method to culturing.
Light Microscopy
Light microscopy is one of the most employed techniques for examining microorganisms. Its importance in microbial detection can't be overstated; it enables researchers to visualize morphology, arrangement, and sometimes even motility of microbes directly. A key benefit is that it allows for real-time observation of living organisms.
The unique feature of light microscopy is its simplicity and ease of use; many laboratories can employ this method without needing specialized training. However, it has limitations in terms of resolution, which may restrict the ability to visualize smaller or less pigmented microorganisms clearly.
Fluorescence Microscopy
Fluorescence microscopy provides an enhanced perspective compared to traditional light microscopy. This technique utilizes fluorescent dyes to stain specific cellular components, allowing for detailed insights into cellular functions and interactions. It's increasingly popular due to its capacity for specific targeting, which can provide high contrast images of cells in various environments.
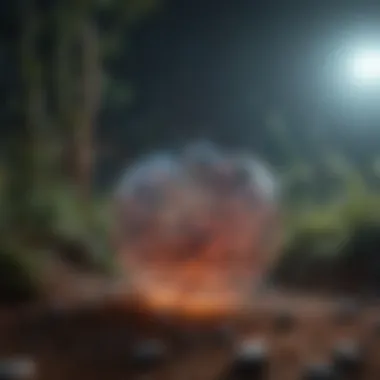
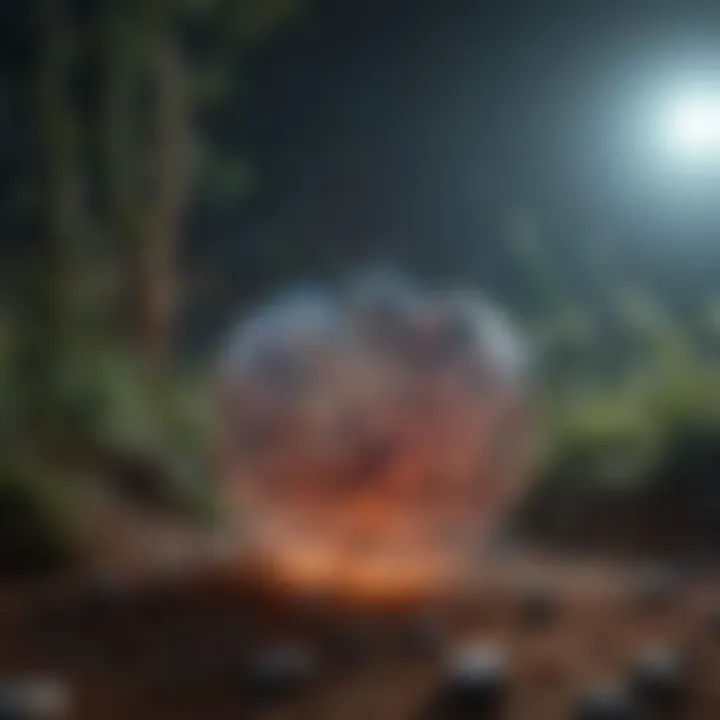
One of the major advantages of fluorescence microscopy is its ability to identify and locate specific pathogens in a complex mixture, crucial in clinical diagnostics. However, limitations include the requirement for specialized equipment and potentially complex staining protocols that can introduce variability in results.
Chemical Detection Methods
Chemical detection methods play a significant role in microbial detection, allowing for the identification of microbial organisms through their specific biochemical reactions. These methods often serve in situations where culturing techniques might fall short, especially in detecting fastidious organisms that are difficult to grow in a laboratory setting. By leveraging chemical reactions, these methods offer faster turnaround times and more specific identification.
Biochemical Tests
Enzymatic Assays
Enzymatic assays, a staple in the toolkit of microbial detection, exploit the unique enzymatic activity of various microorganisms. By measuring the activity of specific enzymes, researchers can identify particular species or strains with precision. This method shines due to its ability to provide quick results, often less than a day, which can be essential for timely decision-making in clinical and environmental settings.
A key characteristic of enzymatic assays is their specificity; they can differentiate closely related microbes, which is crucial in fields like public health where accurate diagnosis is paramount. One unique aspect that sets enzymatic assays apart is their reliance on substrate conversion, leading to detectable changes such as color shifts or fluorescence.
Advantages include ease of use and scalability, as assays can often be performed in multiplex formats, allowing multiple targets to be assessed simultaneously. However, on the flip side, they can sometimes lack sensitivity and might miss low-abundance pathogens. Thus, balancing these aspects is vital when employing enzymatic assays in practice.
Metabolic Profiling
Metabolic profiling takes a broader approach by analyzing the metabolites produced by microorganisms. Through this method, researchers can gain insights into the metabolic activities of an organism, which can be reflective of its biochemical identity. This is particularly beneficial in research applications, providing a holistic view of microbial behavior and interactions within their environments.
One notable characteristic of metabolic profiling is its ability to generate a comprehensive metabolic fingerprint of a microorganism, helping distinguish between closely related species. This means that when quicker, definitive answers are needed, metabolic profiling can be invaluable.
A unique feature of this method is its adaptability; it can be used across various samples, including soil, biofilms, and clinical specimens. The main disadvantage here lies in its complexity, requiring intricate analytics and interpretation of the data gathered. Thus, it often requires specialized knowledge, which may limit its accessibility to some researchers.
Immunological Techniques
ELISA
Enzyme-Linked Immunosorbent Assay (ELISA) is a popular immunological method utilized for detecting proteins, such as antibodies, and specific microbial antigens. It relies on the interaction between an antigen and an antibody to generate a measurable signal, making it a highly sensitive technique.
A standout feature of ELISA lies in its versatility, being applicable in a variety of fields from clinical diagnostics to food safety inspections. What makes it even more appealing is its capacity for high-throughput testing, allowing for the simultaneous analysis of many samples, thus saving time and resources.
However, one must be cautious; while ELISA is dependable and widely recognized, it does require careful calibration and validation to ensure precise results. Limitations can arise when detecting certain antigens, necessitating secondary methods for confirmation.
Western Blotting
Western Blotting is another widely employed immunological technique analyzed protein samples to identify specific proteins. It combines gel electrophoresis with antibody-based detection, allowing researchers to confirm the presence of a particular protein associated with a microbe of interest.
The hallmark of Western blotting is its specificity, as the technique relies on high-affinity antibodies that bind to the desired antigen. This can make it immensely useful in confirming the results obtained from other techniques. Furthermore, it can also provide information about the protein's size, adding another layer to the detection capabilities.
However, this method can be labor-intensive and time-consuming, often requiring multiple steps and controls to achieve credible results. Additionally, it may not be suitable for real-time detection or high-throughput screening. Balancing accuracy with practicality is essential when considering its application in microbial detection.
Molecular Microbial Detection Methods
Molecular microbial detection methods represent a cornerstone in modern microbiology, providing high specificity and sensitivity in identifying microorganisms. These techniques utilize the principles of molecular biology to detect microbial genetic material, allowing researchers to not only pinpoint the presence of pathogens but also assess their abundance and diversity in various environments. This is particularly crucial in fields like clinical diagnostics, where the swift identification of infectious agents can significantly impact treatment outcomes and public health initiatives.
The ability to analyze even minute amounts of DNA or RNA means that these methods are capable of detecting pathogens that traditional culturing techniques might miss. This enhanced capability positions molecular detection as an indispensable tool in the face of emerging microbial threats that challenge existing public health infrastructures.
Polymerase Chain Reaction (PCR)
Real-Time PCR
Real-Time PCR, often called quantitative PCR, is a powerful advancement in PCR technology that allows for the monitoring of the amplification of DNA in real-time. This method stands out due to its speed and precision in quantifying DNA, making it invaluable in many scenarios, including clinical diagnostics and environmental monitoring. Real-Time PCR employs fluorescent dyes that bind to the DNA strand during amplification, giving immediate feedback about the quantity of genetic material present.
One of the key advantages of Real-Time PCR is its ability to provide quantitative data, which is markedly useful when assessing the load of a specific microorganism in a sample. Real-Time PCR is highly favored in research and clinical settings for its efficiency and accuracy. However, it requires calibration and optimization, which can be a stumbling block for some laboratories.
"Real-time PCR has transformed microbial detection by making it faster and more accurate than ever before."
Nested PCR
Nested PCR is another variation of traditional PCR that enhances specificity by conducting two rounds of amplification. The first round uses outer primers that flank the target region, and the second round employs inner primers that further amplify the target. This dual-stage process significantly reduces non-specific amplification, making it especially beneficial when dealing with complex samples laden with genetic material from numerous organisms.
A notable characteristic of Nested PCR is its heightened sensitivity, which enables the detection of low-abundance targets. This makes it popular in research exploring rare pathogens or genetic variants. However, the process can be labor-intensive and is more prone to contamination between amplification rounds. Increased oversight and sterile conditions are critical to ensuring reliable results.
Next-Generation Sequencing (NGS)
Metagenomics
Metagenomics refers to the study of genetic material recovered directly from environmental samples, allowing researchers to analyze the collective DNA of whole communities of microorganisms without isolating individual species. This method has been pivotal in uncovering microbial diversity and community structures in environments ranging from human gut microbiomes to environmental samples such as soil and water.
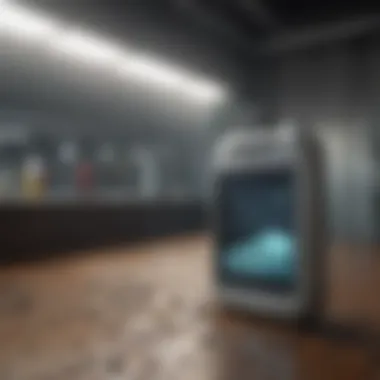
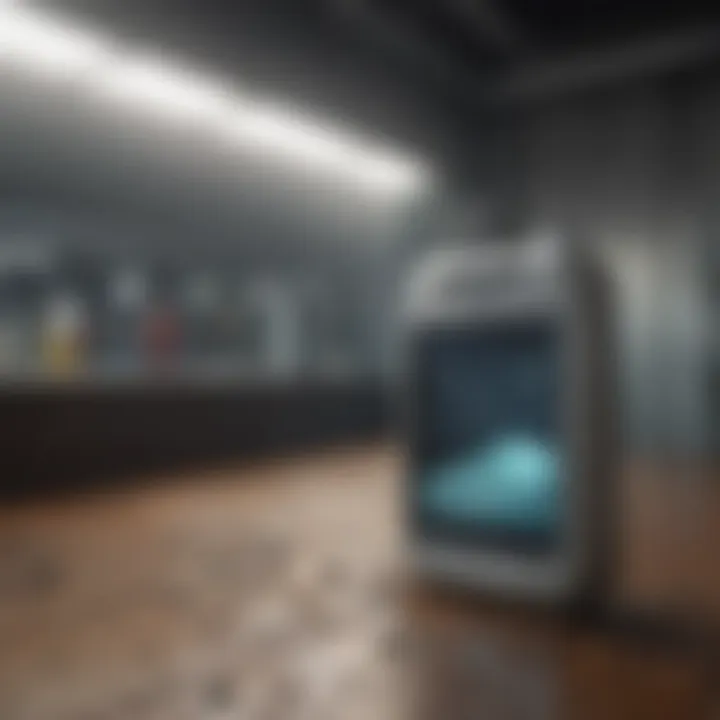
One of the critical aspects of metagenomics is its capability to provide insights into the functional potential of microbial communities. This insight can be vital for understanding their roles in ecosystems, their interactions with hosts, and their contributions to biogeochemical cycles. However, metagenomics can be data-heavy, often requiring advanced bioinformatics tools for analysis, which can be a hurdle for smaller labs.
Whole Genome Sequencing
Whole Genome Sequencing (WGS) analyses the complete DNA sequence of an organism, offering the most comprehensive view of a microbial genome. This method provides invaluable information regarding genetic variations, resistance mechanisms, and phylogenetic relationships among microorganisms. The detailed insights gained from WGS help determine the epidemiology of infectious diseases, trace outbreaks, and understand the evolution of pathogens.
The ability of WGS to deliver thorough data about a microbe’s genetic makeup makes it an exceptional choice for addressing public health concerns. The downside, however, is that WGS can be time-consuming and cost-prohibitive, especially for smaller entities or those just starting with sequencing technologies.
In summary, molecular microbial detection methods embody the advances in microbial analysis that not only enhance our understanding of microbial life but also empower us to address various challenges in health, safety, and environmental sustainability.
Emerging Techniques in Microbial Detection
Emerging techniques in microbial detection are revolutionizing the landscape of how we identify and analyze microorganisms. With advancements in technology, these methods offer unprecedented speed, accuracy, and versatility. Understanding these innovations not only enhances our approach to microbial analysis but also affirms their significance in various fields such as public health, environmental science, and food safety, making it vital for professionals and researchers alike to stay abreast of these developments.
CRISPR-Based Methods
CRISPR technology has made quite the splash in recent years, not just for gene editing but also in microbial detection. By harnessing the precise targeting capabilities of CRISPR, scientists can now detect specific bacterial DNA sequences with remarkable accuracy. This approach capitalizes on the natural defense mechanism of bacteria against viruses, providing a unique and innovative means for microbial identification.
The major benefit of CRISPR-based methods is their specificity. This characteristic allows for the detection of even the faintest traces of harmful microorganisms, making them crucial in a public health context. Besides, the adaptability of CRISPR technology means that once a particular detection system is developed, it can be fine-tuned to target an array of pathogens. However, it's essential to also consider the challenges involved, like the need for sophisticated equipment and expertise.
Biosensors
Integrating biological components with electronic sensing devices, biosensors have opened a new frontier in microbial detection. These tools can provide real-time results, transforming our ability to assess microbial presence and activity. Two main types of biosensors stand out: electrochemical biosensors and optical biosensors.
Electrochemical Biosensors
Electrochemical biosensors leverage the electrical changes that occur during biochemical reactions. This aspect makes them a particularly powerful asset in microbial detection, as they deliver quick and sensitive responses to microbial presence. The key characteristic of these biosensors is their simplicity and low cost, allowing for widespread use in various fields.
One unique feature of electrochemical biosensors is their ability to perform continuous monitoring, a boon for environments that require constant surveillance, like hospitals or food processing plants. However, their specificity can sometimes be questioned; cross-reactivity with similar substances can lead to false positives, thus warranting careful calibration and validation to ensure reliability.
Optical Biosensors
On the other hand, optical biosensors utilize light to detect microorganisms. They work by measuring changes in light properties when a microbe interacts with a sensing element. This method is gaining traction due to its high sensitivity and the non-invasive nature of the detection process.
One key characteristic of optical biosensors is their ability to offer multiplex detection capabilities. This means that multiple pathogens can be detected simultaneously, significantly enhancing throughput in analysis. Nevertheless, while they are highly functional, their cost and complexity can serve as barriers for some applications, making them less accessible compared to simpler methods.
Integrating methods like CRISPR and biosensing technologies addresses the urgent need for innovative microbial detection solutions in a rapidly changing world.
As we move forward, these emerging techniques promise to enhance not just our understanding of microbial populations but also inform public health initiatives and improve safety across various industries.
Applications of Microbial Detection Methods
The applications of microbial detection methods span across various fields, reflecting their centrality in addressing key issues in health, food safety, and the environment. In today's world, where microbial threats are ubiquitous, understanding how to identify and mitigate these risks is crucial. This segment will delve into specific applications that showcase the breadth and depth of microbial detection's relevance in real-world problems.
Public Health
In the realm of public health, microbial detection serves as a frontline defense against pathogens that can cause outbreaks and epidemics. By employing accurate detection methods, healthcare professionals can trace the source of infections, assess the effectiveness of control measures, and evaluate the prevalence of certain diseases in different populations.
- Epidemiological Tracking: Rapid identification of microorganisms can drastically reduce the spread of infectious diseases. For instance, during the COVID-19 pandemic, timely detection of the SARS-CoV-2 virus became critical in containment efforts.
- Hospital-Acquired Infections: Regular microbial surveillance in hospitals helps detect resistant strains of bacteria, which is vital for infection control programs. This proactive approach can minimize the risk for vulnerable patients.
"Microbial detection isn't just about knowing what’s there; it's about preventing what could happen next."
Food Safety
Food safety is another domain where microbial detection methods are indispensable. The consequences of foodborne illnesses can be dire, leading to severe health complications and even death. Understanding which microbes contaminate food products is essential in maintaining public health.
- Pathogen Detection: Regular monitoring for pathogens like Salmonella or E. coli in food products allows companies to recall contaminated items swiftly, reducing risk for consumers.
- Quality Control: In food production, microbiological testing ensures that processes are followed correctly; it helps maintain product consistency and safety. Companies often utilize techniques such as PCR or immunoassays to verify that products meet safety standards before reaching the market.
Environmental Monitoring
Environmental monitoring provides insights into the presence of harmful microbes in various ecosystems, from water sources to soil. By utilizing microbial detection methods, environmental scientists can assess the impact of human activity on ecosystems.
- Water Quality Testing: Regular testing of water sources for microbial contaminants is essential, particularly in areas where industrial waste is a concern. Standards set by organizations like the Environmental Protection Agency help guide testing protocols.
- Soil Analysis: Assessing microbial populations in soil can indicate its health. Studies show that microbial diversity is essential for robust ecosystems, impacting everything from nutrient cycling to plant health.
Challenges in Microbial Detection
The field of microbial detection is fraught with complexities that can throw a wrench into effective analysis. Understanding these challenges is crucial for both researchers and practitioners who rely on accurate microbial detection methods in various applications, from healthcare to environmental monitoring.
Admittedly, microbial detection can often feel like finding a needle in a haystack. The nature of microorganisms means that identifying them accurately takes precision and reliability. This section will delve into two primary challenges: specificity and sensitivity, as well as the pressing need for rapid detection methods. By highlighting these issues, we aim to shed light on critical considerations that impact the efficacy of microbial detection.
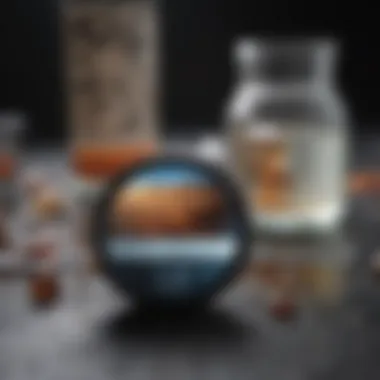
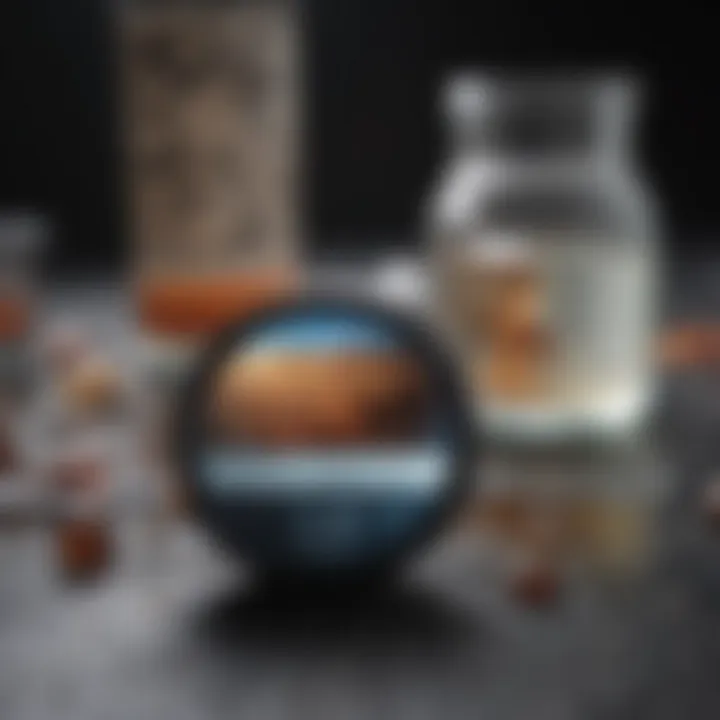
Specificity and Sensitivity
When we talk about specificity and sensitivity in microbial detection, we are addressing fundamental questions concerning the accuracy of detection methods.
Specificity refers to a method's ability to correctly identify a target microorganism while avoiding false positives. In a world where cross-reactivity can cause major disruptions, specificity is paramount. For instance, imagine employing a diagnostic test designed to detect Salmonella but inadvertently cross-reacting with other non-pathogenic bacteria. This not only muddles diagnosis but could lead to inappropriate treatments or interventions.
On the flip side, sensitivity indicates how well a method can detect microorganisms at low concentrations. For instance, in contaminated food samples, finding low levels of pathogens is crucial. A highly sensitive test would help discover these minute levels, potentially preventing outbreaks. However, there’s a delicate balancing act; increasing sensitivity might also lead to false positives, complicating interpretations.
To put it figuratively, consider the case of using a metal detector to find a gold ring in the sand. A device with high specificity would ignore coins and other metals, while high sensitivity would pick up even the tiniest metallic shards, but you’d risk digging up false alarms.
Rapid Detection Needs
In today’s fast-paced world, the importance of rapid microbial detection cannot be overstated. Traditional methods such as culturing bacteria can take days, or even weeks, to yield results. In contrast, many scenarios demand immediate answers — think food safety testing during a supply chain disruption or quick identification of pathogens in a clinical setting.
The need for speed is further emphasized during outbreaks of infectious diseases. In such cases, rapid detection methods can lead to timely public health responses, potentially saving lives. Consider, for example, an emerging viral outbreak. The sooner we identify the causative agent, the quicker healthcare systems can implement containment strategies.
Moreover, industries that rely on microbial presence monitoring, such as breweries, also seek rapid results. An unanticipated bloom in pathogenic microbes could spell disaster for production quality, making rapid detection vital for operational integrity.
"Time lost in detection can translate to lives affected. Rapid microbial detection isn’t just a matter of efficiency; it’s a matter of public health and safety."
The drive for rapid detection is shaping innovations in the field, leading to the development of point-of-care devices and automated systems. These advancements enable quicker turnaround times, allowing stakeholders to respond effectively to microbial threats.
In summary, as we navigate the intricate landscape of microbial detection, addressing the challenges of specificity, sensitivity, and rapid detection becomes ever more critical. Awareness of these intricacies empowers stakeholders to make informed decisions, ultimately enhancing the overall efficacy of microbial detection methodologies.
Future Directions in Microbial Detection
As we look toward the horizon of microbial detection, there’s a substantial shift in how we perceive and utilize these methods. The growing intersection between technology and biology indicates there's much more to explore in this field. Innovations hold the potential to revolutionize the way we detect microbes, and this is particularly relevant in an era where speed and precision are paramount. Key elements like integration of technologies and personalized medicine applications are at the forefront of these future directions, promising significant advances in laboratory practices and healthcare.
Integration of Technologies
The melding of various technologies is set to enhance microbial detection like never before. Imagine a world where smartphone applications collaborate seamlessly with advanced biosensors and molecular methods. This convergence could lead to rapid field-testing capabilities, which are particularly valuable in outbreak scenarios. For example, combining CRISPR technology with smartphone-based analysis can potentially allow anyone, in real-time, to identify microbial threats simply by sampling environmental factors.
- Advantages include:
- Improved accuracy of microbial identification.
- Reduction in time spent on analysis.
- Greater accessibility for non-specialists, broadening the scope of detection initiatives in remote areas.
However, integrating these technologies comes with its own set of challenges. Data management, interoperability of devices, and ensuring that rapid tests maintain high specificity are all key considerations going forward. Collaboration among scientists, engineers, and regulatory bodies will be crucial to forge a path that results in reliable tools for broader use.
Personalized Medicine Applications
Personalized medicine is changing the landscape of healthcare, and microbial detection plays a significant role in this shift. By understanding an individual’s microbial profile, clinicians can tailor treatments to the specific pathogens present in a patient. This goes beyond just fighting infections; it extends into areas like antibiotic stewardship, whereby precise detection reduces the over-prescription of antibiotics.
- Key Benefits:
- Enhanced treatment efficacy through targeted therapies.
- Improved patient outcomes as a result of personalized approaches.
- Reduction in global antibiotic resistance fueled by more responsible usage practices.
"Incorporating microbial detection into personalized medicine not only aids doctors in crafting more effective treatments but also helps mitigate the pressing threat of antimicrobial resistance."
The future isn't just about advancing technologies; it is about creating an interconnected system where microbial detection complements various aspects of health management. The more insights we gain into microbial behaviors and their interactions with human health, the better equipped we’ll be to combat infections in a more informed manner. As this area of research evolves, it will pave the way for greater innovations and applications that touch every corner of health spectrum from hospitals to homes.
By keeping an eye on these futuristic directions, we not only prepare for smarter health interventions but also invite a whole new level of understanding regarding microbes and their robust impact on our world.
Ending
The conclusion serves as a critical element in this exploration of microbial detection methods, weaving together the significant threads laid out throughout the article. By summarizing the key points, it not only reinforces the importance of understanding these detection methods but also emphasizes their far-reaching implications across various fields such as public health, food safety, and environmental monitoring.
Microbial detection methods are the backbone of many significant disciplines. They help in identifying pathogens in health settings, verifying food safety in production lines, and monitoring ecological integrity in our environments. The importance of tailoring methods to fit specific needs cannot be understated, ensuring that they have the sensitivity and specificity required to accurately detect microbial life in diverse contexts.
Moreover, as we have discussed, the integration of traditional and modern techniques creates a more robust approach to microbial detection. Innovations like CRISPR-based methods and biosensors are revolutionizing our ability to respond to microbial threats in real-time, thus enhancing the efficiency and speed at which we manage health outbreaks or food contamination.
"In the rapidly evolving world of microbial analysis, understanding the existing landscape is crucial for the advancement of science and public welfare."
This conclusion serves to remind us that the journey does not end here; rather, it opens the door to future explorations and improvements in our methodologies. Let’s take a look at the summarizing key points regarding this rich topic.
Summarizing Key Points
- Diversity of Methods: Various techniques are employed for microbial detection from traditional culturing to advanced molecular methods, providing flexibility in addressing different scenarios.
- Relevance: Each method has its unique application and limits, influencing their utility across sectors like healthcare, food safety, and environmental health.
- Innovation: Emerging techniques are continuously redefining existing methods, paving the way for faster and more accurate detection.
- Need for Integration: Combining traditional practices with cutting-edge technologies enhances the effectiveness of microbial detection strategies.
Looking Ahead
Looking forward, several promising avenues emerge in the realm of microbial detection:
- Technological Integration: The fusion of different methods, such as PCR with biosensors, is expected to yield more comprehensive detection systems that are both sensitive and rapid.
- Personalized Medicine: Advances in microbial detection could usher in a new era of tailored treatment, especially for infections that have become resistant to conventional methods.
- Continuous Learning: As digital health grows, so will our need to train practitioners and researchers in the latest microbial detection technologies, ensuring that knowledge evolves along with the tools at their disposal.
- Global Collaboration: Cross-border partnerships are essential in addressing global health challenges, particularly in rapid outbreak response strategies.
Embracing these directions could greatly enhance our capability to manage microbial threats more effectively. The journey into the microbial landscape is ongoing, and its significance only seems to grow in our immensely interconnected world.