Techniques and Applications of Mouse Embryonic Stem Cell Culture
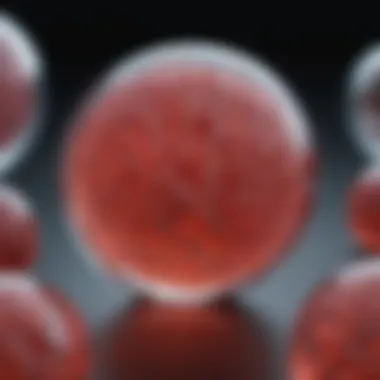
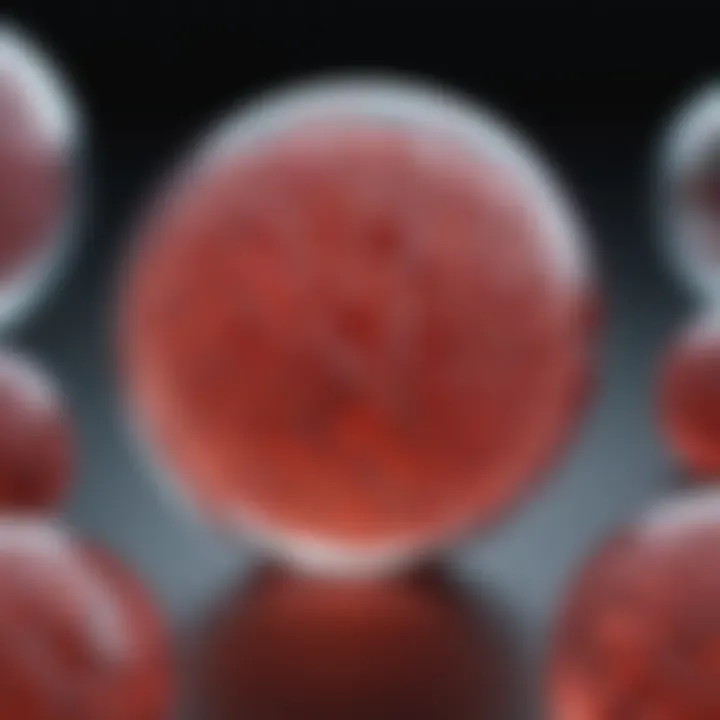
Intro
Mouse embryonic stem cells (mESCs) are a pivotal tool in understanding developmental biology and advancing regenerative medicine. Their unique capacity for self-renewal and pluripotency makes them invaluable for research and therapeutic applications. This article examines the methodologies involved in mouse embryonic stem cell culture, the ethical considerations surrounding their use, and the significance of mESCs in current scientific pursuits. By providing insights into both practical techniques and theoretical frameworks, this narrative aims to enrich the reader's comprehension of this complex discipline.
Research Methodology
In exploring the techniques of mouse embryonic stem cell culture, the research design leans heavily on both empirical data collection and qualitative analysis. The methodology incorporates various approaches tailored to optimize cell isolation, maintenance, and differentiation measurement.
Description of research design and approach
The research utilizes a systematic design focusing on mESCs derived from the inner cell mass of pre-implantation embryos. The design employs both in vitro studies to analyze specific culturing conditions and comparative analyses of different techniques employed in stem cell research. Key factors like growth factors, culture media, and substrate types are analyzed for their impact on cell behavior.
Materials and methods used in the study
The following materials and methods are essential in the process:
- Culture Media: Commonly used media include DMEM (Dulbecco's Modified Eagle Medium) supplemented with FBS (Fetal Bovine Serum) and specific growth factors such as LIF (Leukemia Inhibitory Factor).
- Cell Isolation Techniques: Traditional methods for isolating mESCs involve manual dissection followed by enzymatic dissociation using trypsin or dispase.
- Maintenance Protocols: Cells are routinely passaged every 2 to 3 days to prevent senescence. Careful monitoring of cell morphology is crucial in ensuring optimal growth conditions.
- Differentiation Techniques: Inducing differentiation can be achieved through various approaches such as embryoid body formation or directed differentiation protocols.
Discussion and Interpretation
The study of mESCs reveals significant insights into their role in development and disease modeling. By interpreting findings in the context of existing literature, researchers can contextualize their results within the broader landscape of stem cell research.
Interpretation of results in the context of existing literature
Research shows that variations in culture conditions notably influence cell fate decisions. For instance, studies indicate that mESCs cultured in serum-free conditions exhibit different differentiation characteristics compared to those maintained in serum-supplemented media. This correlates with findings in current literature, emphasizing the importance of precise conditions in culturing methodologies.
Implications for future research or practical applications
Emerging trends suggest a growing interest in using mESCs for modeling human diseases, including cancer and neurodegenerative disorders. The ability to study disease mechanisms using these cells holds promise for future therapeutic interventions. Further, the integration of mESCs into tissue engineering practices could pave the way for innovative treatments, underscoring their relevance in regenerative medicine.
"MESCs offer a window into complexities of development and potential avenues for therapeutic breakthroughs, making them an enduring focus in stem cell research."
Prolusion to Mouse Embryonic Stem Cells
Mouse embryonic stem cells (mESCs) serve as a critical model in biological research, especially for studies concerning development, differentiation, and disease mechanism. These cells are unique in their ability to self-renew and to differentiate into any cell type within the organism, making them invaluable in both basic and applied research.
The significance of mESCs is underscored by their potential applications in regenerative medicine and genetic engineering. As research progresses, understanding the biology of these cells opens doors to innovative therapies for various degenerative diseases. One must also consider the ethical implications surrounding their use, hence establishing robust protocols is essential in research environments.
This section outlines the foundation for exploring the characteristics of mESCs and delineates their pivotal role in modern science. By delving into their definition and importance, we can build a thorough context for the subsequent discussions on techniques and applications.
Definition and Characteristics
Mouse embryonic stem cells are derived from the inner cell mass of blastocyst-stage mouse embryos. They are pluripotent, which signifies that they possess the capability to form all three germ layers: ectoderm, mesoderm, and endoderm. This remarkable attribute positions them at the forefront of developmental biology.
Key characteristics of mESCs include:
- Self-renewal: The ability to divide indefinitely while retaining their undifferentiated state.
- Pluripotency: The capacity to generate any cell type in the organism.
In culture, mESCs require specific media and growth factors that preserve their undifferentiated state. For instance, the addition of leukemia inhibitory factor (LIF) is often essential to maintain pluripotency.
Importance in Research
Research involving mouse embryonic stem cells yields numerous benefits across various scientific fields. Their versatility enables:
- Regenerative medicine: mESCs hold potential for developing cell therapies and tissue engineering applications.
- Modeling diseases: They provide a platform for studying genetic and epigenetic changes during development and disease progression.
- Pharmacological research: mESCs are instrumental in assessing drug responses, toxicity, and efficacy in cell-based assays.
The impact of mESCs on scientific understanding and translational applications cannot be overstated. This research area constantly evolves, with emerging findings driving innovation in both therapeutic strategies and diagnostic tools. Such insights reinforce their role as a cornerstone in fields ranging from developmental biology to oncology.
Isolation of Mouse Embryonic Stem Cells
The isolation of mouse embryonic stem cells is a crucial process in stem cell research. This section elaborates on the significance and methodologies utilized in obtaining these cells. Understanding the techniques of isolation contributes substantially to the overall effectiveness of embryonic stem cell culture. The implications of improper isolation can lead to contamination, reduced cell viability, or compromised pluripotency.
Embryonic stem cells derived from the inner cell mass of early mouse embryos hold tremendous potential. They differentiate into various cell types, making them invaluable in developmental biology and regenerative medicine. The successful isolation of these cells opens the door to numerous applications, including drug testing, disease modeling, and tissue engineering. However, several factors need to be considered to ensure high-quality stem cell lines.
Embryo Collection Techniques
Embryo collection techniques are key in the isolation process. The most common method involves the use of females bred with known genetic backgrounds. Removing the embryos from the uterus typically occurs at the blastocyst stage, around 3.5 days post-fertilization. This stage is when pluripotent cells become accessible.
The collection process requires delicacy and precision to ensure the integrity of the embryos. Surgical procedures may vary but often include:
- Superovulation: Stimulation of female mice with hormones to produce multiple eggs.
- Mating: Co-housing females with males to induce fertilization.
- Dissection and Retrieval: Carefully opening the uterine cavity under sterile conditions, followed by collecting embryos using a fine glass pipette.
When collecting embryos, it's essential to work quickly and maintain a sterile environment to minimize the risk of contamination. The quality of the environment directly impacts the viability of the isolated cells. Moreover, ethical considerations regarding the welfare of the animals also need to be strictly observed.
Cell Extraction Processes
Once the embryos are collected, the next step involves the cell extraction process. This stage is fundamental for obtaining high-quality embryonic stem cells. The extraction process generally entails the following steps:
- Disaggregation of Embryos: The embryos undergo enzymatic treatment to dissociate the cells. Commonly used enzymes include trypsin or collagenase. The choice of enzyme is critical for maintaining cell integrity while ensuring effective dissociation.
- Culturing in Optimal Conditions: After disaggregation, cells are plated on culture dishes pre-coated with feeder layersβsuch as mouse embryonic fibroblast cells. This layer provides crucial signaling factors and nutrients necessary for early stem cell growth and stability.
- Selection of Pluripotent Cells: It is essential to monitor cell colonies for pluripotent characteristics. Morphological assessment, alongside specific marker expression, helps distinguish undifferentiated stem cells from differentiated ones.
- Expansion: Once identified, pluripotent embryonic stem cells can be expanded for further research or therapeutic applications.
Successful isolation of quality cells depends on meticulous techniques and adaptations tailored to specific research goals.
The extraction process not only impacts the quality of harvested cells but also emphasizes the need for consistent methodology. Variability in procedures can lead to different outcomes in stem cell characteristics and functionality. Thus, streamlined protocols should be established and regularly updated per the latest research advancements.
Cell Culture Techniques
Cell culture techniques serve as the backbone of research involving mouse embryonic stem cells. Without these methodologies, the study of these cells would be challenging and the applications in various fields would be limited. These techniques ensure that the cells remain viable, healthy, and undifferentiated, providing a suitable environment for research. A deeper understanding of these techniques can greatly enhance the success of experiments and the reliability of results.
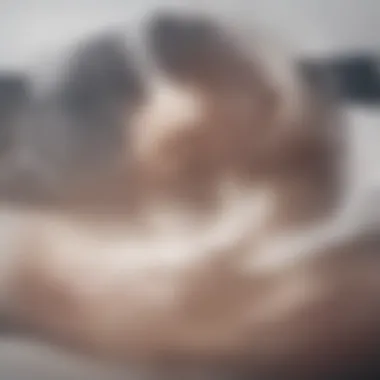
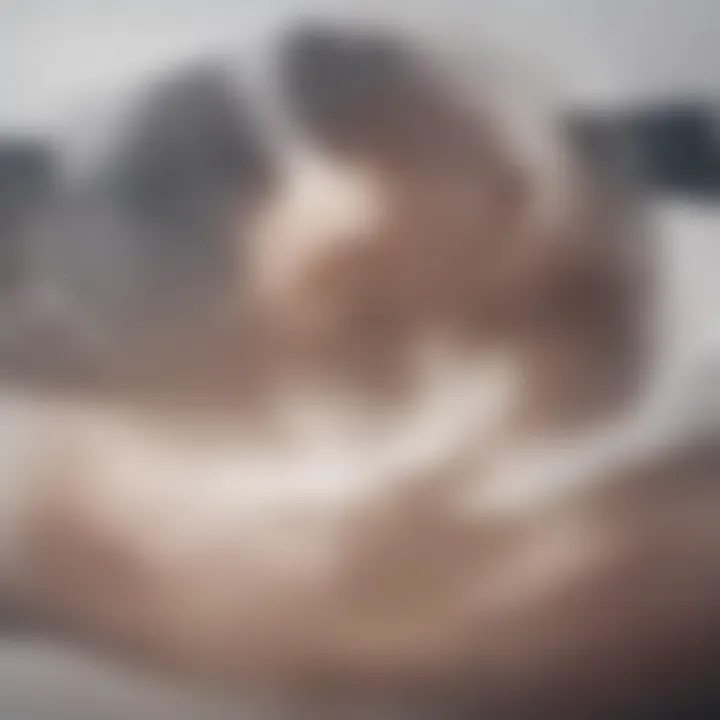
Media Preparation
Preparing culture media is a foundational aspect of cell culture. The media must contain a precise mixture of nutrients, ions, and other components crucial for cell growth and maintenance. Common constituents include amino acids, vitamins, glucose, and salts. Furthermore, some media formulations require supplements, such as fetal bovine serum, to promote cell proliferation.
The preparation process must be executed under sterile conditions to prevent contamination. Maintaining a specific pH and osmolarity is crucial for optimal cell function. It is essential to monitor and adjust these parameters regularly to support the health of the cells.
Use of Growth Factors
Growth factors play a pivotal role in sustaining mouse embryonic stem cells in culture. These are proteins that stimulate cell proliferation, differentiation, and survival. Common growth factors used in stem cell culture include leukemia inhibitory factor and basic fibroblast growth factor. They help maintain the pluripotency of the stem cells by signalling them to remain undifferentiated.
When using growth factors, it is important to adhere to the correct concentrations and timing of administration. Overexposure or underexposure can lead to undesired differentiation, affecting the validity of subsequent experiments.
Maintenance Protocols
Maintaining mouse embryonic stem cells in vitro is not a one-time procedure. It requires continuous monitoring and management of the cell conditions. Regular subculturing is essential. This involves detaching and transferring the cells to a new plate with fresh media. The frequency of subculturing may depend on the growth rate of the cells, usually occurring every few days.
Monitoring cell morphology is also critical. Healthy stem cells should appear round and clustered. Any signs of differentiation or morphological changes may indicate adverse conditions, such as contamination or nutrient depletion.
"The key to effective stem cell research lies not just in the initial isolation, but also in the meticulous culture techniques employed thereafter."
Differentiation of Embryonic Stem Cells
Differentiation of embryonic stem cells is a crucial topic in the study of mouse embryonic stem cells. This process involves the transformation of pluripotent stem cells into specialized cell types. Understanding how to effectively direct this differentiation is vital for applying stem cells in both research and therapeutic contexts. The differentiation of embryonic stem cells is not just about changing one type of cell into another; it embodies the potential for creating tissues that could be used for regenerative medicine, disease modeling, and drug testing.
The differentiation process can be influenced by various factors including growth factors, extracellular matrix components, and environmental conditions. These elements help to guide the stem cells towards desired lineages. Successful differentiation can ultimately lead to the generation of functional tissues, providing invaluable resources for studying disease mechanisms, testing new treatments, or even addressing organ shortage in transplantation.
In Vitro Differentiation
In vitro differentiation is a widely utilized technique that enables researchers to convert mouse embryonic stem cells into specific cell types under controlled laboratory conditions. This method typically involves culturing the stem cells in specific media supplemented with various growth factors that promote the desired lineage specification. The control over the environment allows for a reproducible approach to generating cell types that can mimic physiological conditions.
Protocols for in vitro differentiation vary significantly depending on the target cell type. For example, to differentiate embryonic stem cells into neurons, a combination of retinoic acid and neurotrophic factors is often used. Similarly, to guide them towards a cardiac lineage, factors such as activin A and BMP4 are commonly incorporated into the culture medium. The versatility of in vitro differentiation makes it an essential tool for developmental biology, allowing scientists to dissect the signaling pathways and gene expression patterns involved in cell fate decisions.
Considerations for in vitro differentiation include:
- Optimization of growth factor concentrations: Too much or too little can hinder the process.
- Duration of exposure: Timing is important and varies depending on the specific cell types.
- Monitoring of cell morphology and function: Regular assessments can confirm progress in differentiation.
Lineage Specification
Lineage specification refers to the process of directing embryonic stem cells into more defined progenitor cells or fully differentiated cell types. This aspect of differentiation is critical for studying specific biological pathways and understanding how various cell types arise during development. Researchers aim to identify key signaling molecules and transcription factors that are involved in lineage specification.
A detailed knowledge of lineage specification allows for more refined approaches in regenerative medicine. For example, if a researcher can reliably produce pancreatic beta cells from mouse embryonic stem cells, this can have profound implications for diabetes treatments.
Key elements in lineage specification include:
- Extrinsic factors: These are signals from the surrounding environment that influence stem cell fate.
- Intrinsic factors: This involves the genetic and epigenetic landscape of the cells themselves, influencing how they respond to external cues.
Differentiation and lineage specification are fundamental processes that bridge the gap between basic research and clinical applications. They serve as vital components in advancing our understanding of developmental biology and therapeutic interventions derived from stem cell research.
Quality Control Measures
Quality control measures are critical in mouse embryonic stem cell culture. They ensure that the cells maintained for research or therapeutic applications meet the required standards for viability, functionality, and sterility. These measures mitigate the risk of contamination and inconsistencies that can lead to defective or non-reproducible results. Following stringent quality control protocols enhances the reliability of experimental outcomes, which is particularly important in advanced studies like regenerative medicine and cancer research.
Cell Viability Assessments
Cell viability assessments are fundamental to determining the health and quality of embryonic stem cells. Testing viability helps researchers understand whether the cells are living, functional, and capable of differentiating as expected. Common techniques for assessing cell viability include:
- Trypan Blue Exclusion Test: This classic method stains dead cells while live cells remain colorless, allowing for an easy visual count under a microscope.
- MTT Assay: This colorimetric assay measures metabolic activity. Viable cells convert MTT into formazan crystals, which can be quantified spectrophotometrically.
- Flow Cytometry: This advanced method can quantify viable versus non-viable cells with greater accuracy by utilizing fluorescent dyes that distinguish between live and dead cells.
Regular cell viability assessments are necessary to maintain a high quality of embryonic stem cell lines. Keeping track of viability not only informs researchers about the culture's status but also influences experimental decisions, such as when to passage or differentiate cells.
Contamination Checks
Contamination can compromise the integrity of mouse embryonic stem cell cultures. Therefore, implementing robust contamination checks is essential. The sources of contamination often stem from bacteria, fungi, or mycoplasma. Regular monitoring can look at several aspects:
- Visual Inspections: Routine checks for turbidity or unusual color changes in the culture medium can hint at microbial growth.
- Microbial Culture Tests: Transfer samples of culture media to agar plates to detect bacterial or fungal contamination.
- PCR Testing for Mycoplasma: This sensitive method can detect mycoplasma contamination, which is often unnoticed but can significantly alter cell behavior.
By instituting regular contamination checks, researchers can help ensure the purity of their stem cell cultures. This not only extends the life of the cell lines but also enhances the validity of subsequent experiments.
"Quality control is not just a regulatory function but a pathway to scientific integrity and reproducibility in research."
Incorporating strict quality control measures will ultimately lead to increased reproducibility and reliability in mouse embryonic stem cell research.
Ethical Considerations
The topic of ethical considerations in mouse embryonic stem cell research is paramount. This area of study holds both immense potential and complex moral dilemmas. Ethical considerations guide researchers in aligning their work with societal values. They ensure respect for life forms and promote responsible science. Researchers must be aware of legal regulations, informed consent, and broader implications of their work.
Legal Regulations
Legal frameworks governing embryonic stem cell research vary globally. In the United States, the National Institutes of Health (NIH) establishes guidelines for federally funded research. The NIH mandates compliance with regulations regarding stem cell lines derived from embryos. The guidelines specify that only certain cell lines, created prior to August 2001, may receive federal funding.
In Europe, guidelines are more stringent in many countries. The European Union's legislation often prohibits the creation of embryos for research purposes. Countries like Germany and Italy have strict laws against the use of embryonic stem cells, reflecting broader societal concerns.
The ethical landscape is continually changing, influenced by scientific advancements and public opinion.
This legal environment requires researchers to stay informed and ensure their work complies with current regulations. Ignoring legal guidelines can lead to severe penalties and lost funding opportunities. Researchers must document compliance and frequently reassess their practices.
Best Practices for Ethical Research
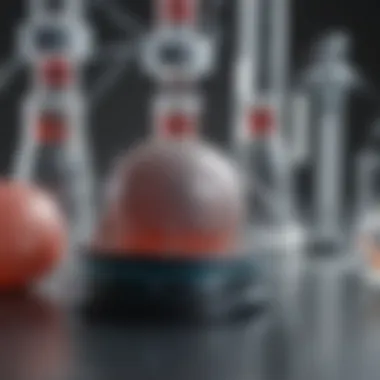
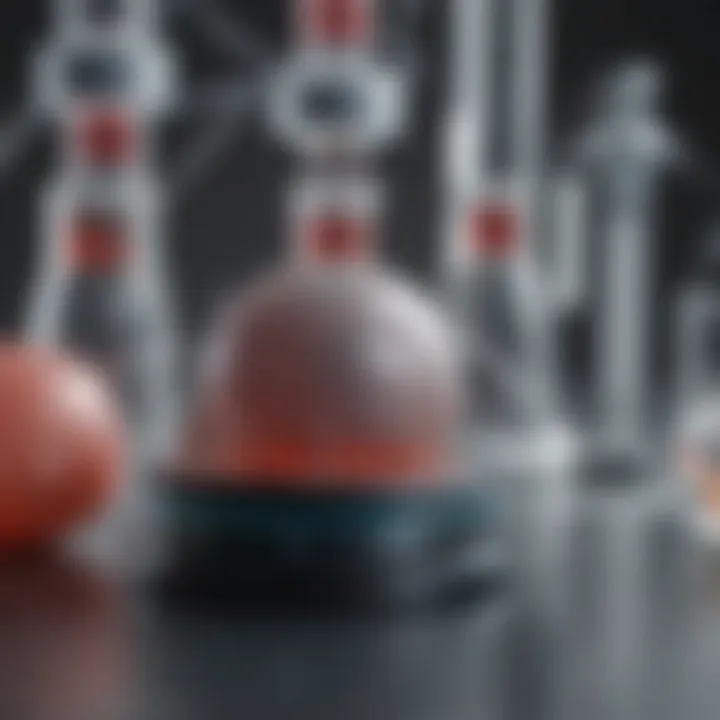
Adhering to best practices is essential for maintaining ethical standards in stem cell research. Key practices include:
- Informed Consent: All donors must be fully informed about the use of their embryos. Clarity on the purpose, risks, and outcomes is crucial. Informed consent transcends legal validity and establishes trust.
- Transparency: Researchers should communicate their methods clearly to the scientific community and the public. Openness strengthens credibility and fosters collaborative efforts.
- Oversight Committees: Establishing internal review boards helps guide ethical compliance. These committees evaluate research proposals, ensuring they meet ethical standards before approval.
- Public Engagement: Engaging with stakeholders, including the public, is significant. Researchers should consider public concerns and insights into ethical dimensions. Public engagement can influence the acceptance and direction of research.
Practicing these guidelines not only enhances the validity of research but also supports the integrity of the scientific community. By navigating the complexities of ethical considerations, researchers contribute to the advancement of knowledge while respecting moral imperatives.
Applications in Regenerative Medicine
Mouse embryonic stem cells (mESCs) represent a significant advancement in regenerative medicine. Their unique properties and capabilities allow for various applications that hold promise for future medical treatments. The importance of mESCs lies in their pluripotency, meaning they can differentiate into any cell type in the body. This capability enables researchers to explore new frontiers in restoring function to damaged tissues and organs.
One primary consideration in using mESCs for regenerative medicine is the potential for generating specific cell types needed for therapy. For example, scientists are actively investigating how mESCs can produce cardiac, neuronal, and pancreatic cells. Each of these applications addresses critical health issues such as heart disease, neurodegenerative disorders, and diabetes. Thus, mESCs provide a pathway for creating specialized cells that can be used in transplantation, overcoming donor organ shortages.
Moreover, the development of mESC-derived tissue engineering strategies can lead to innovative therapeutic solutions. The potential for creating three-dimensional tissues mimics real biological structures and functions more effectively than two-dimensional cultures. These engineered tissues can eventually be used for drug testing, disease modeling, and regenerative therapies.
"The promise of regenerative medicine lies in our ability to harness the unique properties of stem cells to repair and regenerate damaged tissues and organs."
Potential for Tissue Engineering
Tissue engineering often combines cells, scaffolds, and growth factors to develop functional tissues. mESCs play a crucial role in these processes due to their pluripotent nature. They can be guided to become various cell types that can populate a scaffold.
In mESC-derived tissue engineering, several factors contribute to successful outcomes:
- Scaffold Materials: It is essential to choose biocompatible material for scaffolds. Natural materials like collagen and synthetic materials such as polylactic acid are often used. These materials need to support cell adhesion and growth.
- Cell Source: Using cells from mESCs is a strategy that allows for the creation of tissue-specific cells in a controlled manner. This reduces the risk of immune rejection in transplantation scenarios.
- Growth Factors: Adding specific growth factors guides the differentiation of mESCs into desired cell types and promotes tissue growth.
Through these collaborative efforts, mESCs can potentially create cardiac patches for heart repair or neural constructs for repairing nerve damage. The versatility and adaptability of mESCs make them invaluable in tissue engineering, addressing many challenges in regenerative medicine.
Cell Therapy Approaches
Cell therapy is a direct application of using mESCs to treat diseases. This approach involves transplanting cells into a patientβs body to repair damaged tissues or replace lost function.
Some advantages of using mESCs in cell therapy include:
- Targeted Treatment: Therapeutic cells derived from mESCs can be directed towards specific tissues, making interventions more effective.
- Reduced Immune Response: If the mESCs are derived from the patient or genetically matched donors, there is a lower risk of rejection by the immune system.
- Clonal Expansion: mESCs can be expanded in culture, ensuring an adequate supply of therapeutic cells. This scalability is key for clinical applications.
Current research focuses on several diseases that could benefit from mESC-based therapies:
- Cardiovascular Diseases: mESC-derived cardiac cells show potential in repairing heart tissues after myocardial infarction.
- Diabetes: Generation of insulin-producing pancreatic cells from mESCs could offer new treatment options for diabetic patients.
- Neurological Disorders: There is significant interest in using mESCs to generate neural cells that can potentially restore lost function in neurodegenerative diseases.
Role in Cancer Research
The study of mouse embryonic stem cells holds profound implications for cancer research. These cells provide an invaluable model for understanding tumor biology and evaluating potential therapeutic strategies. By harnessing the unique properties of stem cells, researchers can probe into the mechanisms of cancer, identify new drug targets, and improve the efficacy of existing treatments. This section discusses two critical aspects: modeling tumor biology and studying drug responses.
Modeling Tumor Biology
Mouse embryonic stem cells can differentiate into various cell types, including those resembling tumor cells. This capability allows researchers to investigate how normal cells transform into cancerous cells. It offers insights into essential processes such as cellular signaling, genetic mutations, and microenvironment interactions.
Using these models, scientists can manipulate gene expression, facilitating the understanding of oncogenes and tumor suppressor genes. For instance, through targeted gene editing techniques like CRISPR, one can induce mutations that mimic those found in human cancers. This approach opens new pathways for discovering therapeutic agents that can act on specific pathways involved in tumor progression.
The ability to model human cancer conditions in mice enables more accurate testing of therapeutic interventions, bridging the gap between bench research and clinical application.
Studying Drug Responses
In cancer treatment, understanding the response of tumor cells to drugs is pivotal. Mouse embryonic stem cells enable researchers to investigate how different cell types respond to various chemotherapeutic agents. This can lead to the identification of specific markers of sensitivity or resistance to treatment.
By exposing embryonic stem cell-derived tumor models to drugs, scientists can:
- Assess efficacy and toxicity in a controlled environment.
- Investigate mechanisms of resistance underlying treatment failures.
- Screen potential novel drug compounds for effectiveness.
Moreover, studying drug responses in these models can inform clinical decisions, leading to personalized medicine approaches. By selecting drugs based on specific genetic profiles, oncologists may enhance patient outcomes significantly.
In summary, the role of mouse embryonic stem cells in cancer research is crucial. Their ability to model tumor biology and facilitate studies on drug responses lays the groundwork for innovative cancer therapies. These research efforts could eventually translate to improved treatments for patients, highlighting the relevance of stem cell research in oncology.
Genetic Studies and Engineering
Genetic studies and engineering have become critical components in the field of embryonic stem cell research. Understanding the genetic makeup and the manipulation of genes in mouse embryonic stem cells facilitate advancements in various biological domains. These studies reveal insights into gene functions and their interactions, providing pathways to understand diseases, develop new therapies, and innovate methodologies in regenerative medicine.
CRISPR Applications
The CRISPR-Cas9 technology represents a revolutionary approach in genetic engineering. It allows for precise modifications to be made in the genome of mouse embryonic stem cells. This tool has made it feasible to create gene knockouts or introduce specific mutations that can model human diseases accurately.
Some notable benefits of CRISPR applications include:
- Targeted Gene Modification: Scientists can selectively alter genes, which helps in studying gene functions.
- Ease of Use: Compared to traditional gene-editing methods, CRISPR is more manageable and efficient.
- Cost-Effective: This technology lowers the financial barrier for carrying out sophisticated genetic engineering projects.
Furthermore, the impact of CRISPR technology on research is profound. It enhances the feasibility of designing mouse models that mirror human conditions more closely than ever before, thus moving translational research forward.
Transgenic Models
Transgenic models are another significant aspect of genetic engineering in this context. These models involve the introduction of foreign DNA into the mouse embryonic stem cells, leading to the expression of new traits. They serve important functions in various areas such as:
- Studying Gene Function: By observing the effects of introduced genes, researchers can elucidate their roles in biological processes.
- Disease Modeling: Transgenic models can replicate certain diseases, allowing researchers to test drugs and observe disease progression in a controlled environment.
- Therapeutic Research: Understanding genetic diseases can lead to novel treatment strategies. Transgenic models aid in identifying potential therapeutic targets.
Overall, genetic studies and engineering, specifically through CRISPR applications and transgenic models, broaden the horizons of mouse embryonic stem cell research. These methodologies provide powerful platforms for advancing our knowledge in genetics, disease mechanisms, and therapeutic developments. The implications of these techniques resonate within regenerative medicine and beyond, paving the way for future discoveries.
Emerging Technologies in Cell Culture
Emerging technologies in cell culture have become a crucial area of focus in recent years. They enhance the capabilities of researchers in manipulating and studying mouse embryonic stem cells. These advancements not only improve the efficiency of research but also offer significant benefits in terms of reproducibility, scalability, and precision. Therefore, understanding these technologies is essential for anyone involved in stem cell research or applications.
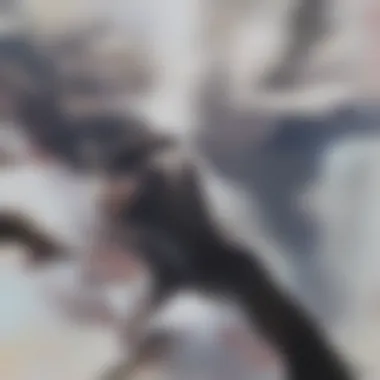
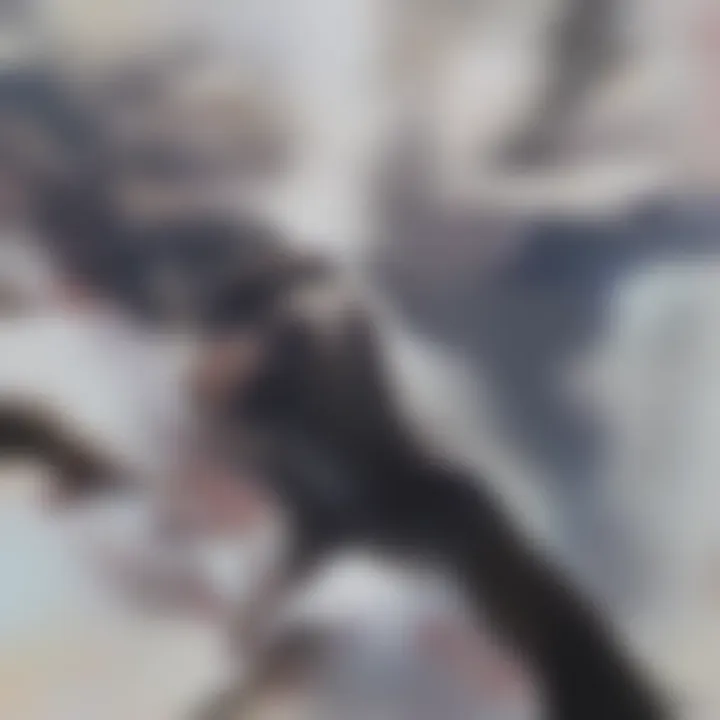
Bioprinting Innovations
Bioprinting is one of the most promising technologies in the field of cell culture. It involves the layer-by-layer deposition of cells and biomaterials, creating three-dimensional structures that mimic natural tissues. This technique allows for precise control over cell placement, which is vital in creating functional tissue constructs.
Some key aspects of bioprinting innovations include:
- Customization of Cell Structures: Researchers can design specific tissue shapes and architectures, catering to various biological needs.
- Material Versatility: A wide range of biomaterials can be used in conjunction with living cells, enabling the creation of complex environments that support cell growth and differentiation.
- Scalability: Bioprinting facilitates the production of large numbers of tissues, which can be invaluable for drug testing and regenerative medicine.
Bioprinting represents a shift towards personalized medicine, potentially enabling custom tissue grafts for patients.
Despite its potential, challenges remain. These include ensuring cell viability post-printing and optimizing the printing process itself to avoid damaging sensitive cells. Researchers are actively working to overcome these hurdles to realize the full promise of this technology.
Automation and Robotics in Culture
Automation and robotics play an increasingly important role in the process of cell culture, including mouse embryonic stem cells. By integrating automated systems in laboratories, researchers can streamline workflows, reduce human error, and increase reproducibility in experiments. This is particularly relevant in high-throughput screening and large-scale cell production.
The significance of automation in cell culture includes:
- Enhanced Consistency: Automated systems provide uniformity in cell handling and treatment, which is essential for reliable results.
- Increased Throughput: Laboratories can process more samples in less time, leading to faster discoveries and advancements in research.
- Data Integration: Advanced robotic systems often include software that collects and analyzes data in real-time, aiding researchers in making informed decisions throughout their experiments.
Automation is not without its challenges. Capital investment can be significant, and the learning curve associated with new technologies can be steep for some researchers. However, the long-term benefits often outweigh these initial obstacles, particularly in terms of resource allocation and research quality.
In summary, emerging technologies such as bioprinting and automation in cell culture are transforming the landscape of mouse embryonic stem cell research. By facilitating innovative approaches and improving existing methods, these technologies are paving the way for breakthroughs in regenerative medicine, cancer research, and beyond.
Challenges in Mouse Embryonic Stem Cell Research
Research in mouse embryonic stem cells presents significant challenges that affect advancement in the field. Addressing these challenges is crucial for maximizing the potential of embryonic stem cells in various applications, including regenerative medicine and genetic engineering. Understanding technical obstacles and reproducibility issues help in developing more efficient protocols and methodologies.
Technical Obstacles
Technical obstacles in mouse embryonic stem cell research can arise from various aspects of the culture process. These can hinder the progress in achieving reliable results. Key points include:
- Cell Line Variability: Different mouse strains may yield embryonic stem cells with distinct behaviors. This variability can lead to inconsistent results across studies.
- Culture Environment: The conditions under which stem cells are cultured, such as the composition of media and the presence of growth factors, can significantly impact cell viability and differentiation potential.
- Handling Techniques: Procedure-based issues can occur during the isolation and transfer of embryonic stem cells. Such technical errors may compromise the integrity and functionality of the cells.
These factors must be carefully controlled to ensure reproducibility and reliability of experimental results.
Reproducibility Issues
Reproducibility is a cornerstone of scientific research yet poses significant challenges in mouse embryonic stem cell studies. The following elements can contribute to reproducibility issues:
- Protocol Variation: Even slight differences in the methodologies applied can lead to distinct biological outcomes. Therefore, standardized protocols become vital to enhance reproducibility across different laboratories.
- Biological Variability: Genetic differences among mouse models and environmental factors can further complicate reproducibility. Results obtained from one embryonic stem cell line may not translate effectively to another.
- Documentation Practices: Inadequate documentation of experimental procedures makes it challenging for others to replicate the research. Clear, detailed records are essential for ensuring transparency and reproducibility in findings.
As the field progresses, addressing these challenges will be important to foster trust and reliability in the applications of mouse embryonic stem cells.
Future Directions in Research
The field of mouse embryonic stem cell research is at a pivotal point. Understanding and implementing innovative strategies can lead to significant breakthroughs in various domains of biological science. Considering the complexity of biological systems, interdisciplinary approaches and next-generation techniques are essential to advance our knowledge and capabilities.
Interdisciplinary Approaches
Interdisciplinary collaboration becomes increasingly valuable as research expands its frontier. This involves merging expertise from various fields such as molecular biology, bioinformatics, engineering, and even ethics. For instance, integrating bioinformatics can aid in analyzing large datasets generated during stem cell research. This analysis can enhance our understanding of gene expression and cellular behavior in different environments.
Additionally, engineers can contribute to the design of better culture systems. This includes bioreactors that can precisely control environmental conditions, such as nutrient supply and waste removal. Such innovations lead to improved cell yields and quality, further supporting downstream applications.
The inclusion of ethical considerations is also critical. Collaborations with ethicists can ensure that research aligns with societal values and regulations. As research impacts public health and policy, focusing on ethical standards will promote trust and acceptance of stem cell technologies.
This approach has multiple benefits:
- Enhanced innovation through diverse perspectives.
- More comprehensive understanding of complex biological systems.
- Improved methodologies that consider technical, social, and ethical dimensions.
Next Generation Techniques
Next-generation techniques are becoming crucial in the landscape of mouse embryonic stem cell research. As technology evolves, researchers are continually adapting new tools to enhance efficiency and effectiveness in culture practices.
One of the forefront techniques includes CRISPR-Cas9 gene editing. This method allows for precise modifications of the stem cell genome, facilitating the study of gene functions and the development of disease models. With high efficiency and specificity, CRISPR has transformed experimental designs and expanded the scope of research possibilities.
Moreover, advances in single-cell sequencing technologies are enabling researchers to analyze gene expression at a single-cell resolution. This method illuminates heterogeneity within stem cell populations, offering insights into differentiation processes and stem cell identity. By understanding these cellular dynamics, the information can lead to targeted therapies and improved regenerative techniques.
Emerging bioprinting technologies also show promise for creating complex tissue structures from stem cells. This innovation holds potential for modeling organ systems and investigating disease mechanisms in more physiological contexts, leading to potential advancements in drug testing and therapeutic interventions.
To summarize the significance of next-generation techniques:
- They provide precise control over genomic and epigenomic changes.
- They analyze cellular behavior with unprecedented detail.
- They enable the biofabrication of tissues mimicking native environments.
As the landscape of mouse embryonic stem cell research evolves, these future directions hold immense potential for transformative discoveries that could reshape our understanding of biology and its applications.
Culmination
In summarizing the extensive exploration of mouse embryonic stem cell culture, it becomes clear that understanding this field is vital for multiple scientific disciplines. The techniques outlined in the article pave the way for advancements in regenerative medicine, cancer research, and genetic studies. Moreover, the ethical considerations associated with using embryonic stem cells cannot be understated; they play a crucial role in shaping how research is conducted and perceived.
The benefits of mastering these techniques go beyond basic knowledge. Researchers are better equipped to innovate in areas such as tissue engineering and therapeutic applications. By implementing stringent quality control measures, the reliability and integrity of experimental outcomes can be ensured. This ultimately fosters a promising future for stem cell research, influencing therapies and treatments for various diseases.
"Understanding mouse embryonic stem cell culture is not just fundamental; it is foundational to the future of biology and medicine."
Summary of Key Points
- The significance of mouse embryonic stem cells in research spans across regenerative medicine, cancer studies, and genetic engineering.
- Techniques such as media preparation, growth factor utilization, and differentiation protocols play a significant role in effective cell culture.
- Quality control measures are essential to maintain cell viability and prevent contamination.
- Ethical considerations must be at the forefront of research involving embryonic stem cells, guiding best practices and legal compliance.
- Emerging technologies, like bioprinting and automation, are reshaping the future of stem cell culture.
Implications for Future Research
The future research landscape in mouse embryonic stem cell culture presents numerous opportunities. Interdisciplinary approaches can lead to groundbreaking discoveries that enhance our understanding of cell biology and human health. The integration of next-generation techniques will likely refine existing methods and introduce novel applications.
As researchers continue to explore this area, they will need to address the challenges such as reproducibility and technical obstacles. Collaborative efforts can facilitate the sharing of protocols and findings, ultimately advancing the field. The potential to develop therapies tailored for individual patients makes these avenues of research crucial not just for science, but for society as a whole.