Proton Transfer Reaction Mass Spectrometry Explained
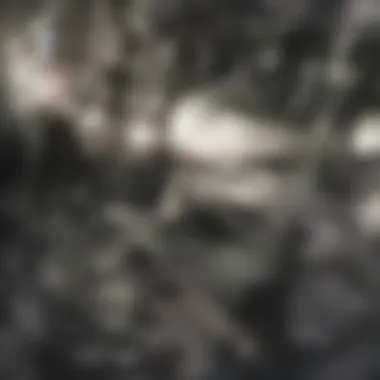
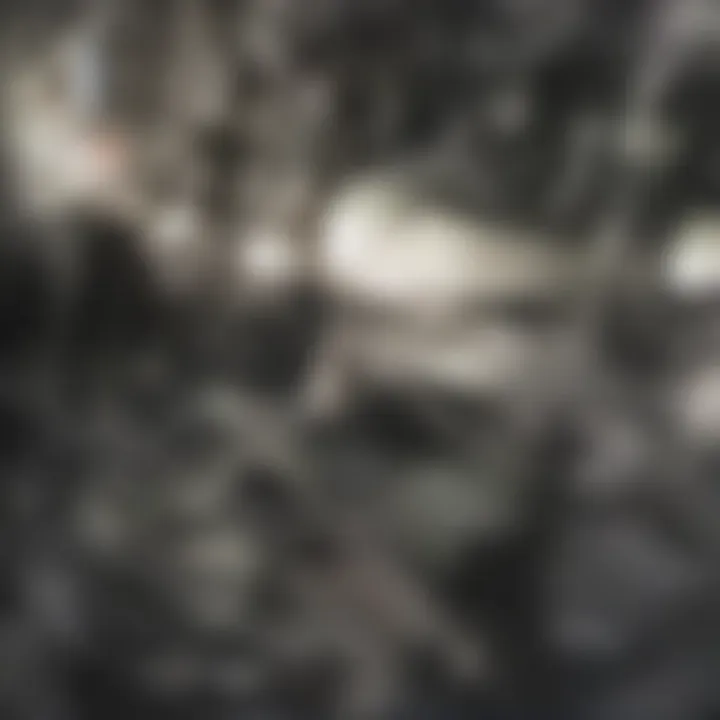
Intro
Proton Transfer Reaction Mass Spectrometry (PTR-MS) is an analytical technique that has gained traction in various fields due to its ability to provide real-time monitoring of volatile organic compounds (VOCs). This method distinguishes itself through high sensitivity and specificity, making it an essential tool in numerous applications, such as environmental monitoring, medical diagnostics, and food quality control. Understanding the key principles of this technique is valuable for students, researchers, educators, and professionals alike, giving insight into its significance and practicality in contemporary scientific research.
Research Methodology
Description of research design and approach
The overarching aim of PTR-MS research is to enhance the detection and analysis of VOCs. This involves a systematic approach to study the fundamentals of proton transfer reactions and develop better instrumentation for mass spectrometry. The research design hinges on both experimental and theoretical frameworks. Practitioners often employ comparison studies involving various samples to validate the accuracy and reliability of PTR-MS.
Materials and methods used in the study
In this context, some materials necessary for PTR-MS analysis include:
- Mass spectrometers: Instruments like the IONICON PTR-QMS or Agilent 7200 provide the backbone for detecting ions.
- Test samples: Volatile organic compounds from environmental sources, biological samples, or food products.
- Calibration standards: Essential for ensuring precision in measurements.
The methodology often includes:
- Sample collection: Extracting VOCs from targeted environments or subjects.
- Ionization process: In the PTR-MS setup, ions from the sample are generated through proton transfer reactions.
- Mass analysis: The produced ions are analyzed using a mass spectrometer to determine their mass-to-charge ratios.
Attention to detail during each stage is critical for achieving accurate results.
Discussion and Interpretation
Interpretation of results in the context of existing literature
Interpreting results from PTR-MS requires a thorough understanding of both the output data and its relevance to existing literature. Researchers frequently compare findings with previous studies to establish trends or discrepancies. For instance, comparisons with chromatographic methods can highlight the advantages of the faster response time of PTR-MS.
Implications for future research or practical applications
The implications of applying PTR-MS are vast. Potential for future research includes:
- Advancing instrumentation: Continued development could lead to more compact and sensitive devices, broadening application scopes.
- In-depth studies on environmental impacts: Monitoring VOCs in various environments may lead to improved strategies for pollution control.
- Expansion into clinical diagnostics: Refinement in detecting specific biomarkers through VOC analysis could revolutionize medical diagnostics.
The versatility and potential applications indicate a strong future for PTR-MS in multiple domains.
Preface to Proton Transfer Reaction Mass Spectrometry
Proton Transfer Reaction Mass Spectrometry (PTR-MS) represents a pivotal advancement in analytical chemistry, enabling the analysis of volatile organic compounds (VOCs) in real time. This technology merges the principles of ion chemistry with mass spectrometry, facilitating fast and reliable detection of various substances. The importance of understanding PTR-MS revolves around its applications across multiple fields such as environmental sciences, medical diagnostics, and food safety. The rise in environmental pollution and the need for quick diagnosis in medical settings underscore the relevance of this technique today.
Historical Background
The development of PTR-MS can be traced back to the 1990s when it was first introduced as a method to measure trace gases. Early applications were primarily in environmental studies to detect atmospheric gases, where traditional methods struggled due to sensitivity and speed limitations. Over the years, enhancements in ionization processes and mass spectrometric technologies have refined PTR-MS, allowing it to overcome the challenges faced by earlier methodologies. The evolution of PTR-MS reflects a broader trend in analytical chemistry: the shift toward real-time analysis and the increasing demand for precise, on-the-spot measurements.
Significance of PTR-MS
The significance of PTR-MS lies in its ability to deliver rapid results without extensive sample preparation. This is crucial in scenarios where time is of the essence, such as monitoring air quality in urban environments or detecting diseases through breath analysis. Due to its high sensitivity and specificity, PTR-MS offers an accurate portrayal of VOC concentrations, aiding researchers and practitioners in making informed decisions. Furthermore, the versatility of PTR-MS extends to areas like food quality control, where it helps to ensure safety and compliance with health regulations.
"Proton Transfer Reaction Mass Spectrometry has transformed how we analyze volatile substances, enabling real-time decisions that were previously unfeasible."
In summary, understanding the foundational aspects of PTR-MS enhances one’s appreciation of its widespread applications and the impact it has in making significant strides in scientific research and public health.
Fundamental Principles of PTR-MS
The fundamental principles of Proton Transfer Reaction Mass Spectrometry (PTR-MS) are crucial to understanding how this technique operates. It coalesces various physical and chemical processes. By recognizing these principles, researchers can harness the full potential of PTR-MS for real-time analysis of volatile organic compounds (VOCs). This section articulates the mechanics behind proton transfer reactions, the ionization processes involved, and the mass spectrometry framework that supports the method.
Basics of Proton Transfer Reactions
Proton transfer reactions serve as the cornerstone of PTR-MS technology. In essence, these reactions involve the transfer of a proton from a reagent ion to a neutral molecule. This interaction is highly efficient for many types of VOCs. The atmospheric pressure ionization ensures fast reactions, allowing immediate detection. The ability to vary the reagent ions provides flexibility for analyzing different compounds.
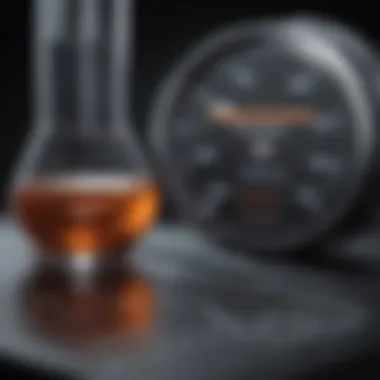
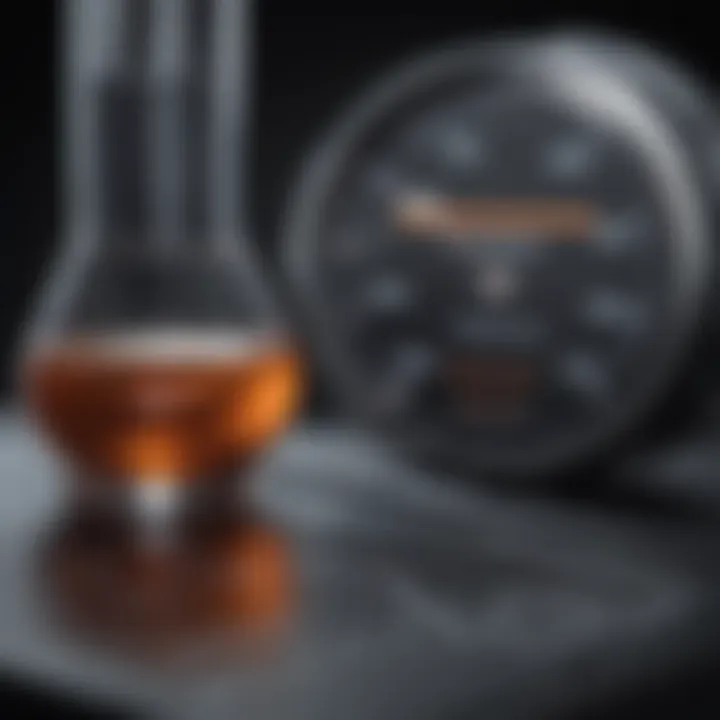
Various factors, such as the acidity of the analyte and the reaction conditions, influence efficiency. Higher acidity in the analyte typically results in enhanced proton transfer rates. Understanding these dynamics is essential for optimizing experimental conditions.
Its relevancy in environmental monitoring and medical diagnostics cannot be overstated. By implementing these reactions, PTR-MS can achieve high sensitivity and selectivity in detecting trace amounts of VOCs in complex mixtures.
Ionization Process in PTR-MS
The ionization process in PTR-MS is fundamentally a two-step affair. First, a reagent ion, usually hydrated protons (O+), interacts with the target VOC. Upon successfully transferring a proton, the target molecule becomes positively charged. This ionization is predominantly soft, minimizing fragmentation of the analyte.
Soft ionization is vital because it preserves intact molecular information, essential for accurate identification. Moreover, the mechanism of ionization aids in achieving low detection limits. Researchers must be aware of the ionization efficiency, as it varies with structure, composition, and the presence of other molecules.
By setting appropriate parameters in the PTR-MS setup, the ionization process can be made more efficient. This allows for more comprehensive analysis of complex samples from different domains, including environmental studies and health assessments.
Mass Spectrometry Overview
Mass spectrometry (MS) is the analytical method responsible for detecting and quantifying ions produced during the ionization process. In the context of PTR-MS, it is crucial for providing the final output: a mass spectrum depicting the presence of various ions and their respective abundances. This process generally involves three main stages: ionization, analysis, and detection.
The mass analyzer, a key component, separates ions based on their mass-to-charge ratios (m/z). Common types of mass analyzers used in PTR-MS include quadrupole and time-of-flight (TOF) spectrometers. The choice of mass analyzer directly affects resolution and sensitivity, thereby influencing the accuracy of the measurements.
Given the rapid advancements in mass spectrometry technology, PTR-MS benefits from improved instrument design. Enhanced resolution and speed facilitate more detailed analysis, allowing researchers to identify minute concentrations of VOCs with greater confidence.
The unique attributes of PTR-MS, stemming from its principles, position it as a powerful tool in various fields of scientific inquiry, advocating its importance in contemporary research.
Instrumentation in PTR-MS
The instrumentation in Proton Transfer Reaction Mass Spectrometry (PTR-MS) is critical for its effectiveness and functionality. Understanding the specific components and their roles provides key insights into how this sophisticated analytical technique operates. Proper instrumentation ensures accurate measurement of volatile organic compounds, making it indispensable for various applications, including environmental monitoring, medical diagnostics, and food quality control. Each component in the PTR-MS system must be carefully selected and integrated to enhance overall performance.
Key Components of PTR-MS Systems
Reaction Chamber
The reaction chamber is a central feature in PTR-MS systems. It offers a controlled environment where proton transfer reactions occur. This chamber is designed to optimize the interaction between the ions and the analytes. A key characteristic of the reaction chamber is its ability to maintain a specific pressure and temperature, which is crucial for reproducibility and sensitivity of the measurements. Its closed structure enables better control over the sample atmosphere.
One unique feature of the reaction chamber is its ability to support various ionization modes. This flexibility enables researchers to adapt the system for diverse analytical needs. The advantage of this adaptability is clear; it makes the PTR-MS applicable in different scientific fields. However, care must be taken regarding the chamber’s material, as some materials may react with volatile compounds, potentially impacting results.
Ion Source
The ion source is another essential component of PTR-MS systems. It generates primary ions that will interact with the sample’s volatile compounds. One notable aspect of the ion source is its efficiency in producing stable ions, which is vital for accurate measurements.
A common choice for the ion source in PTR-MS is the chemical ionization method. This approach is beneficial because it allows for soft ionization, meaning that it produces ions without fragmenting them significantly. A unique feature of these ion sources is their compatibility with a wide range of VOCs. This versatility allows analysts to detect and quantify various organic compounds effectively. The potential downside, however, is that some ion sources may have limitations in ionization efficiency for specific compounds, which can affect sensitivity.
Mass Analyzer
The mass analyzer serves a vital role in separating ions based on their mass-to-charge ratio after ionization. It is crucial in determining the identity and quantity of the volatile compounds present in the sample. A key characteristic of mass analyzers in PTR-MS is their high resolution and speed, which allows for real-time data acquisition.
Quadrupole mass filters are among the most widely used mass analyzers in PTR-MS. Their popularity stems from their robustness and reliability. A significant advantage of quadrupole analyzers is their ability to filter ions selectively, providing clarity in data interpretation. However, these mass analyzers can be limited in their ability to analyze complex mixtures, as overlapping signals can complicate results.
Advancements in Instrumentation
The field of PTR-MS is evolving rapidly, primarily through advancements in instrumentation. New technologies are enhancing the sensitivity and reliability of measurements. Innovations include miniaturized devices, which allow for portable and field-based applications. Enhanced software algorithms improve data interpretation, making analysis more efficient. These ongoing advancements further widen the scope and practicality of PTR-MS in various research and industrial contexts.
In summary, the instrumentation discussed in this section demonstrates the delicate balance between efficiency, selectivity, and adaptability in PTR-MS systems. Each component plays a vital role in achieving precise and accurate analysis of VOCs, which is crucial for the technique's broad applications.
Methodologies Employed in PTR-MS
Understanding the methodologies employed in Proton Transfer Reaction Mass Spectrometry (PTR-MS) is essential for comprehending how this technology functions in various applications. These methodologies influence the effectiveness, sensitivity, and specificity of the measurements that PTR-MS can achieve. In particular, sampling techniques, calibration procedures, and data acquisition and analysis methods play a significant role in the overall performance and reliability of PTR-MS. This section delves into these components, highlighting their importance and offering insights into their intricate workings.
Sampling Techniques
Direct Sampling
Direct sampling is a foundational technique in PTR-MS where gas samples are taken directly from the ambient environment without any modification. This method allows for immediate analysis and quick response, crucial for real-time monitoring of volatile organic compounds (VOCs).
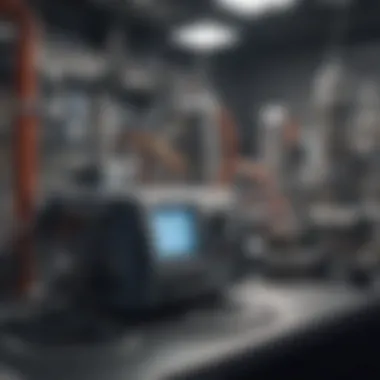
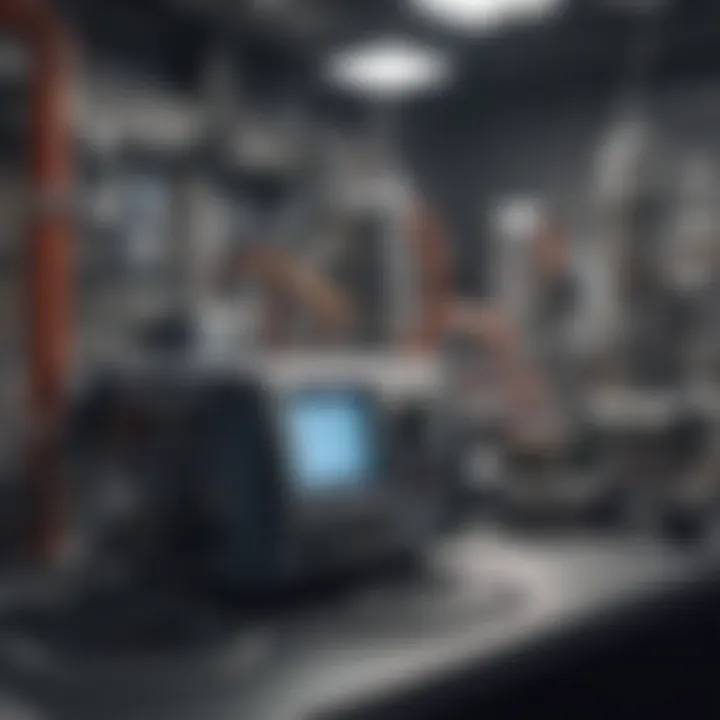
A key characteristic of direct sampling is its simplicity. It enables researchers to capture the sample without complex preparations or intermediary steps, ensuring that the results closely represent the true state of the environment. One benefit of this method is its time efficiency, facilitating rapid data collection, which is invaluable for applications in environmental monitoring.
However, direct sampling also comes with its unique challenges. For instance, it can be subject to variations in atmospheric pressure and temperature, which might influence the concentration of analytes. Ensuring accurate measurements in variable environmental conditions can be problematic.
Dynamic Headspace Sampling
Dynamic headspace sampling serves as an alternative to direct sampling, particularly beneficial in specific applications where analyte concentrations are low. This method involves collecting vapor from a sample into which a carrier gas is introduced, creating a flowing stream that allows for the concentration of target compounds.
The key characteristic of dynamic headspace sampling is its ability to enhance sensitivity. By enriching the sample, it allows for the detection of lower concentrations of VOCs, which can be critical in applications like medical diagnostics where precise detection is essential. This technique is often favored in scenarios where direct sampling may miss trace levels of compounds.
Nonetheless, dynamic headspace sampling is not without its drawbacks. The process can be more complex, necessitating carefully controlled conditions to prevent dilution or loss of volatile compounds during transfer. This may introduce challenges in reproducibility and reliability.
Calibration Procedures
Calibration is a vital aspect of ensuring the accuracy of PTR-MS results. The calibration process establishes a relationship between the instrument's response and the known concentrations of specific target compounds. This method allows for quantification and enhances the reliability of the analysis.
Calibration procedures can vary based on the application. Often, researchers utilize calibration gases with known concentrations to calibrate the instrument. Regular calibration is important to account for factors like instrument drift and variations in response over time.
Data Acquisition and Analysis
Data acquisition and analysis in PTR-MS encompass the methods used to collect and interpret the mass spectral data obtained from the instrument. A significant advantage of PTR-MS is its ability to capture real-time data, which allows for immediate assessment and action when necessary.
Data analysis involves interpreting the mass spectra generated by the instrument to identify and quantify the compounds present in the sample. Advanced software tools aid in this process, allowing for complex data interpretation that can unveil insights into the sample composition. The integration of robust data analysis methods ensures accurate decision-making in various fields, including environmental science and healthcare.
Applications of Proton Transfer Reaction Mass Spectrometry
Proton Transfer Reaction Mass Spectrometry, or PTR-MS, stands out in analytical chemistry due to its versatility and efficiency. The ability to monitor volatile organic compounds (VOCs) in real-time makes it invaluable in various sectors. Understanding its applications is crucial in appreciating its role in scientific research and practical uses.
Environmental Monitoring
Environmental monitoring is one of the key areas where PTR-MS has made significant impacts. It enables the quantification of gases in the atmosphere, aiding in the detection of pollutants, such as nitrogen oxides and sulfur compounds. This technique enhances air quality assessments through its rapid response times. As environmental regulations become stricter, real-time monitoring is essential for compliance and public health. This method allows for continuous sampling, ultimately leading to immediate data availability for analysis and decision-making.
- Key Benefits:
- High sensitivity to low concentrations of VOCs.
- Continuous monitoring capabilities.
- Facilitates rapid identification of pollution sources.
Given the growing concerns related to climate change, the urgency of precise environmental monitoring continues to increase. By implementing PTR-MS, researchers and agencies can gather essential data to support environmental policies.
Medical Diagnostics
In the sphere of medical diagnostics, PTR-MS offers innovative advantages. The ability to detect specific biomarkers in breath samples provides a non-invasive and rapid diagnostic approach. For conditions such as lung cancer or metabolic disorders, early detection can significantly alter treatment outcomes.
Moreover, the specificity of PTR-MS allows for distinguishing between healthy and diseased states through metabolic profiling. This capability can lead to personalized medicine where treatments are tailored to individual metabolic responses.
- Advantages:
- Non-invasive sampling of breath.
- Potential for rapid screening of diseases.
As healthcare evolves towards more precise methodologies, PTR-MS is positioned to play a central role in improving diagnostic frameworks.
Food Quality Control
The food industry benefits from PTR-MS through the enhancement of quality control processes. The detection of volatile compounds helps assess freshness, spoilage, and contamination in food products. For instance, analyzing the aroma of fruits can determine ripeness, while assessing the levels of certain compounds can indicate spoilage.
This method's ability to analyze multiple samples quickly ensures that quality standards are maintained. The direct analysis of food matrices without extensive sample preparation streamlines processes and increases efficiency.
- Notable Points:
- Rapid assessment of food quality.
- Enhanced safety through contamination detection.
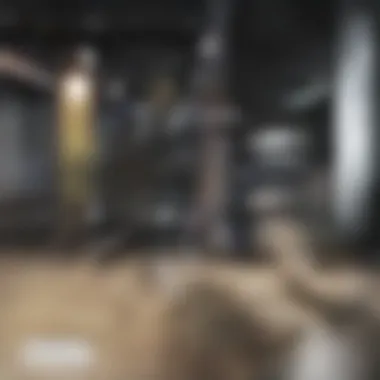
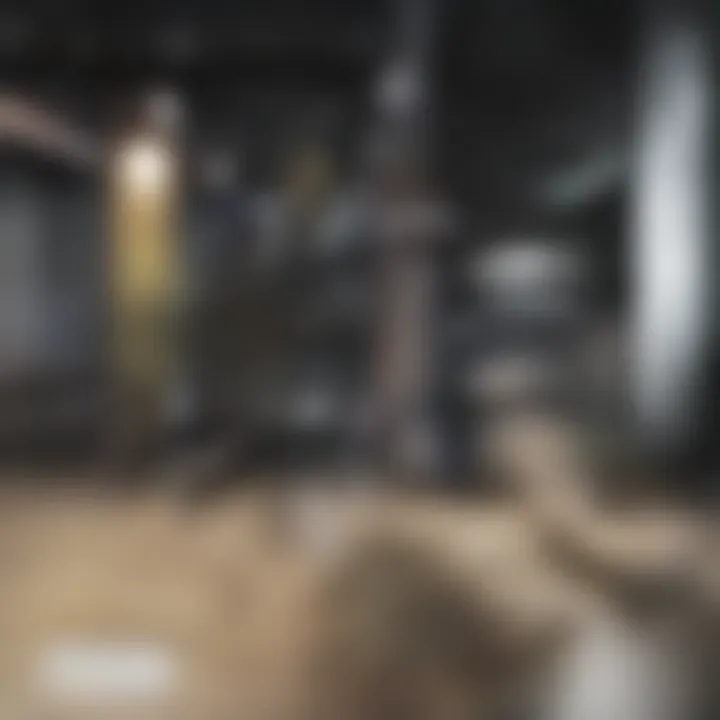
The integration of PTR-MS in food quality control reflects a growing trend towards using advanced analytical techniques to ensure consumer safety.
Industrial Applications
In industrial settings, PTR-MS is utilized across various domains such as petrochemical monitoring and process control. It can track emissions during chemical manufacturing, ensuring that processes run efficiently and comply with environmental standards. The ability to monitor VOCs in real-time supports companies in optimizing operations and minimizing waste.
Furthermore, in the area of product quality assurance, the method can evaluate the formulation of products, from perfumes to paints, ensuring consistent quality in production.
- Importance:
- Effective emissions monitoring in manufacturing.
- Quality assurance of industrial products.
The industrial applications of PTR-MS highlight its efficiency in enhancing both environmental compliance and product quality, reinforcing its value in modern production environments.
Challenges and Limitations of PTR-MS
Proton Transfer Reaction Mass Spectrometry (PTR-MS) offers innovative advantages in the detection of volatile organic compounds (VOCs). Nonetheless, this technique is not without its challenges and limitations. Comprehending these obstacles is crucial for students, researchers, and industry professionals who aim to leverage PTR-MS in their work. This section addresses the sensitivity and detection limits of PTR-MS and the interference issues that can affect accuracy.
Sensitivity and Detection Limits
Sensitivity in PTR-MS is a pivotal characteristic that determines the capability of the method to detect low concentrations of target analytes. While PTR-MS is known for its capability to achieve high sensitivity, there are inherent limitations that should be acknowledged. The detection limits can vary widely depending on several factors such as:
- Ionization Efficiency: The efficiency with which a compound is ionized plays a crucial role in the sensitivity. Certain compounds may have significantly lower ionization efficiency, resulting in higher detection limits.
- Matrix Effects: The presence of various chemicals in a sample can hinder the ionization of target VOCs, thereby affecting sensitivity and leading to ambiguous results.
- Instrument Calibration: Without proper calibration, the sensitivity of the measurements can decrease. Calibration standards must represent the actual conditions to ensure reliable detection limits.
Overall, the ability to detect and quantify VOCs at low concentrations is essential, especially in fields such as environmental science and health diagnostics.
Interference Issues
Interference presents another noteworthy challenge in PTR-MS. This occurs when compounds other than the target VOCs affect the measurement. A few key points regarding interference include:
- Chemical Similarity: Compounds that are chemically similar to the target analytes can ionize similarly, making it difficult to distinguish between them. This can lead to false positives.
- Background Contaminants: The ambient environment where sampling occurs may contain various VOCs that can interfere with the analysis. It is essential to minimize background contamination in order to produce accurate results.
- Reaction Chemistry: The behavior of certain VOCs in the reaction chamber can lead to unwanted side reactions. Such reactions can generate additional ions that may complicate the mass spectra, ultimately skewing the interpretation of data.
Addressing these interference issues is critical for improving the reliability of PTR-MS results. Researchers must implement strategies for mitigating these challenges to enhance accuracy in their analyses.
In summary, while PTR-MS provides significant advantages for real-time VOC monitoring, it is imperative to recognize and address both its sensitivity and interference-related limitations. Identifying these challenges can lead to improved methodologies and enhance the overall effectiveness of this analytical tool.
Future Directions in PTR-MS Research
The field of Proton Transfer Reaction Mass Spectrometry (PTR-MS) is continuously evolving. Researchers are actively seeking to enhance the method's capabilities and broaden its application scope. This section will discuss emerging techniques and the integration of PTR-MS with other analytical methods, which are essential for future advancements in the field.
Emerging Techniques
Emerging techniques in PTR-MS are paving the way for more precise, efficient, and versatile applications. Some notable developments include:
- Advanced Ionization Techniques: New ionization approaches, such as electrospray ionization or atmospheric pressure chemical ionization, can improve sensitivity. These techniques allow for the analysis of a wider range of compounds, including less volatile species.
- Miniaturization: Smaller, portable PTR-MS instruments are being designed. These devices facilitate real-time field measurements, making them valuable for environmental studies and medical diagnostics.
- High-Resolution Mass Spectrometry: Integrating high-resolution mass analyzers enhances the discrimination of isomers. This means researchers can differentiate compounds that have very similar mass-to-charge ratios, thus increasing the reliability of data.
- Online Data Processing: Real-time data processing techniques are becoming more prevalent. The use of advanced algorithms can improve the analysis speed, allowing for quicker decision-making in various applications.
The adoption of these emerging techniques can significantly enhance the role of PTR-MS in current research and industry.
Integration with Other Analytical Methods
The integration of PTR-MS with other analytical methods can increase its utility and applicability across various domains. Some considerations of this integration include:
- Gas Chromatography (GC): Coupling PTR-MS with gas chromatography allows for the separation of complex mixtures before mass spectrometric analysis. This combination benefits applications where sample matrices are intricate or contain numerous overlapping signals.
- High-Performance Liquid Chromatography (HPLC): Similarly, integrating HPLC with PTR-MS can facilitate the analysis of non-volatile and thermally unstable compounds. This approach can enhance detection limits for specific analytes of interest, increasing the overall analytical capability.
- Nuclear Magnetic Resonance (NMR): NMR can complement PTR-MS analysis by providing structural information about compounds that have been identified using PTR-MS. Together, these techniques offer a more comprehensive data set.
- Data Fusion Techniques: Combining PTR-MS data with results from other analytical instruments can create a more holistic view of the sample being studied. Data fusion approaches can lead to improved accuracy and robustness in analytical interpretations.
Combining PTR-MS with other methods not only expands its application range but also enhances the overall analytic workflow and outcome.
Ending
The conclusion of this article encapsulates the essential findings and insights regarding Proton Transfer Reaction Mass Spectrometry (PTR-MS). This analytical technique is crucial for its ability to monitor volatile organic compounds (VOCs) with real-time analysis and precision. In various fields, such as environmental monitoring, medical diagnostics, and food quality assurance, PTR-MS has established itself as a key tool.
The significance of PTR-MS lies in several factors:
- High Sensitivity and Specificity
PTR-MS allows for the detection of compounds at low concentrations, enhancing its effectiveness in research and practical applications. - Real-Time Analysis
Its capability for immediate results means that decisions can be made swiftly, which is crucial in environments where time-sensitive reactions occur. - Versatility Across Disciplines
The wide range of applications makes PTR-MS relevant in many sectors, thus attracting attention from diverse scientific communities. - Ongoing Advancements
Continuous improvements in instrumentation and methodology are expanding the potential uses of PTR-MS further, indicating a promising future for this technology.
Overall, this article highlights that understanding the underlying principles, methodologies, and applications of PTR-MS is vital for professionals in analytical chemistry and related fields. Its integration into current research practices ensures that PTR-MS will remain at the forefront of analytical techniques for years to come.
"The ongoing evolution of Proton Transfer Reaction Mass Spectrometry reflects the growing need for rapid and reliable analytical methods in the scientific community."