Exploring Radiation Resistance in Cancer Treatment

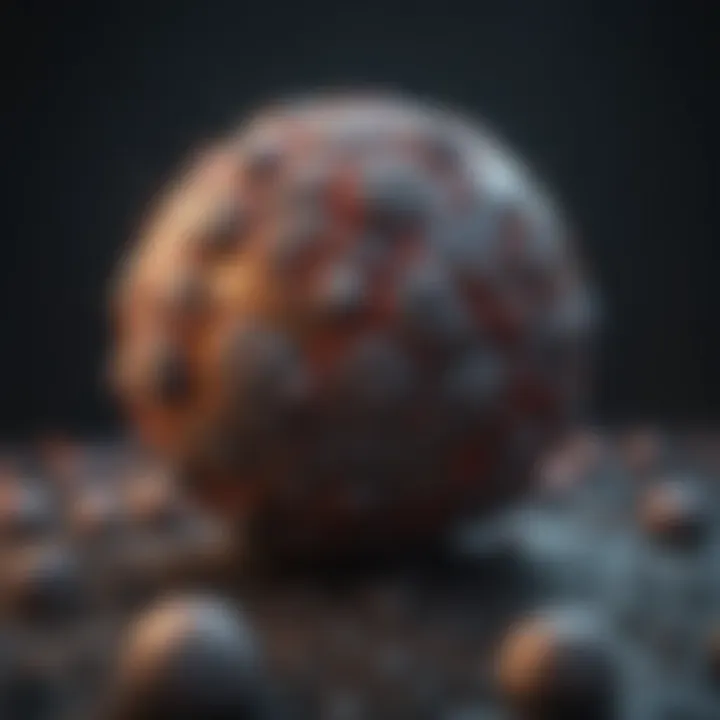
Intro
Radiation therapy continues to be a cornerstone in the fight against cancer. However, a recurring challenge in treatment outcomes is the phenomenon of radiation resistance. Understanding the mechanics behind this resistance is crucial for improving therapeutic efficacy. This section will set the stage for a detailed exploration of the various factors leading to radiation resistance.
At the very foundation of this discussion lies the complexity of cancer biology. Tumors are not merely collections of malignant cells; they embody dynamic ecosystems shaped by genetic mutations, epigenetic modifications, and microenvironmental influences. By investigating these dimensions, we can better appreciate the implications of radiation resistance and explore potential interventions that may overcome it.
The strain on conventional therapies necessitates an evolving discourse among researchers and clinicians. As the landscape of cancer treatment shifts, unearthing insights on radiation resistance can guide future methodologies and therapeutic strategies. This article aims to be a valuable resource for students, researchers, educators, and professionals seeking to deepen their understanding of this pressing issue.
Research Methodology
In order to thoroughly assess the various mechanisms of radiation resistance, a multifaceted research design has been employed. This approach incorporates both experimental and observational methodologies, combining laboratory studies with real-world clinical observations.
Description of Research Design and Approach
The research is grounded in a systematic review of existing literature and experimental data. This review method allows for the synthesis of various studies, thereby painting a comprehensive picture of radiation resistance. Control experiments were conducted using a selection of cancer cell lines that exhibit known varying levels of radiation sensitivity.
Materials and Methods Used in the Study
- Cell Lines: Various cancer cell lines were chosen, including:
- Radiation Exposure: Cells received controlled doses of ionizing radiation using a linear accelerator.
- Assessment Techniques: A combination of techniques was employed:
- HeLa (cervical cancer)
- A549 (lung cancer)
- MCF-7 (breast cancer)
- Clonogenic Assays: To gauge cell survival post-radiation.
- Gene Expression Analysis: To evaluate changes in key genetic markers associated with resistance.
- Microenvironmental Studies: Utilizing 3D cultures to replicate tumor environments.
These methodologies allow researchers not only to identify the underlying mechanisms leading to resistance but also to derive plausible strategies for overcoming the challenges posed by radiation therapy.
Discussion and Interpretation
The findings reveal a diverse array of factors contributing to radiation resistance, including:
- Genetic mutations that alter DNA repair pathways.
- Epigenetic changes that modify gene expression without altering the DNA sequence.
- The tumor microenvironment that can shield cells from effective radiation exposure.
"Understanding these elements is key for future advancements in overcoming radiation resistance."
As we interpret the results, they must be framed within the context of existing literature. Previous studies have established foundational concepts in the realm of tumor biology, which this research builds upon. The alignment or divergence from established findings provides avenues for further investigation.
Implications for Future Research or Practical Applications
The implications of these findings extend into clinical practice. Understanding the mechanisms of radiation resistance may lead to enhanced treatment protocols and personalized medicine strategies tailored to individual tumor profiles. Future research should focus on:
- Development of targeted therapies that can be combined with radiation.
- Exploration of microenvironmental modifications to increase treatment efficacy.
By addressing radiation resistance comprehensively, we can potentially reshape how we approach cancer treatment in the years to come.
Preface to Radiation Resistance
Understanding radiation resistance is critical in cancer biology and treatment. The phenomenon plays a significant role in the efficacy of radiotherapy, which is a key approach in managing various cancer types. When resistance occurs, this can lead to treatment failure, necessitating a deeper investigation into the underlying mechanisms.
Definition and Importance
Radiation resistance refers to the ability of cancer cells to withstand exposure to ionizing radiation. This resistance affects how these cells respond to treatment, impacting the overall success of therapy. Identifying the factors that contribute to this resistance is vital. This knowledge can lead to the development of new strategies to increase the effectiveness of radiotherapy.
Radiation resistance is important for both clinical outcomes and research. Clinically, it informs treatment planning and patient management strategies. In research, it drives the investigation into potential therapeutic targets. Improved understanding of this trait could also aid in personalizing treatments, ensuring that every patient receives the best care possible.
Overview of Radiotherapy in Cancer Treatment
Radiotherapy uses ionizing radiation to eliminate cancer cells. It is typically used in various ways, such as primary treatment, postoperative adjuvant therapy, and for palliative care. The precision of modern radiation techniques has improved patient outcomes considerably. However, not all tumors respond effectively to radiation, with some exhibiting significant radiation resistance.
The impact of radiotherapy on tumors hinges on several factors. Tumor cell characteristics, surrounding microenvironment, and treatment protocols all contribute to the outcome. Understanding these elements helps refine treatment approaches. For instance, combining radiotherapy with chemotherapy or immunotherapy may enhance efficacy, especially in resistant cancers. This combination can help overcome some mechanisms of resistance, ultimately leading to better patient prognosis.
The Biological Basis of Radiation Resistance
The understanding of radiation resistance in cancer therapy hinges significantly on its biological underpinnings. This section explores how cells have developed mechanisms that enable them to withstand the damaging effects of radiation. By comprehending these mechanisms, researchers can identify the challenges they present in treatment.
Radiation therapy, a cornerstone of cancer care, often encounters the problem of tumor cells that resist its effects. This resistance can stem from various cellular processes and molecular factors. Analyzing these topics contributes to developing more effective treatment strategies and improved patient outcomes.
Cellular Mechanisms of Resistance
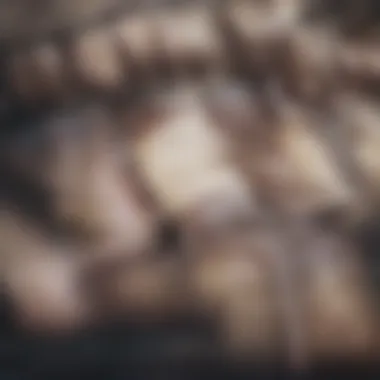
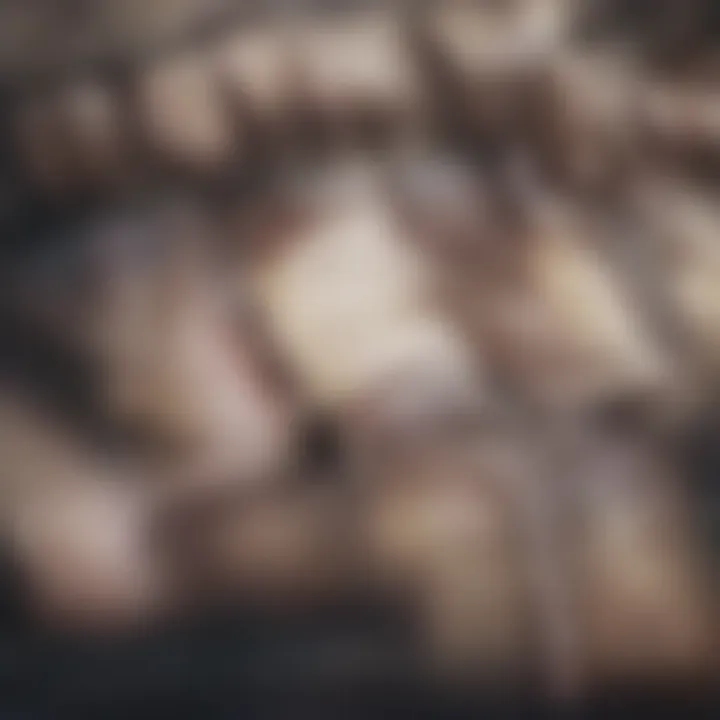
DNA Damage Repair Pathways
DNA damage repair pathways are vital in determining a cell's fate after radiation exposure. When radiation induces damage to DNA, the cell attempts to fix it through complex repair mechanisms. The efficiency and accuracy of these pathways are crucial; if errors occur during repair, it can lead to additional mutations.
One key characteristic of these pathways is their redundancy. Cells possess multiple mechanisms to repair DNA damage, including homologous recombination and non-homologous end joining. This feature makes it challenging to eliminate resistant tumor cells effectively. Understanding how these pathways function can help identify bottlenecks that might be targeted with therapies, enhancing radiation sensitivity.
However, a potential disadvantage lies in the ability of cancerous cells to upregulate these pathways upon exposure to radiation. This adaptation allows them not just to survive treatment but to proliferate even more vigorously, complicating the overall treatment process.
Cell Cycle Regulation
Cell cycle regulation plays a crucial role in how tumor cells respond to radiation. The cell cycle consists of phases during which the cell grows, prepares for division, and divides. Radiosensitivity varies across these phases, with cells in the G2/M phase generally being the most vulnerable.
Tumor cells often exhibit dysregulated cell cycle checkpoints. This can result in premature entry into the cell cycle or an inability to halt division in response to DNA damage. Such behaviors are significant because they confer radioresistance. By exploring cell cycle dynamics, it may be possible to devise strategies that can sensitize these cells to radiation, making treatment more effective.
However, the adaptation of some tumor cells to alter their cycling patterns upon exposure to radiation is a downside. This adaptive behavior can create subsequent challenges for treatment, requiring a nuanced understanding of cell biology and cycle progression.
Apoptosis Inhibition
Apoptosis, or programmed cell death, is a vital safeguard against cancer. Cellular mechanisms promoting apoptosis usually act as a counterbalance to uncontrolled cell division. When cells encounter radiation, those that sustain significant damage usually undergo apoptosis.
However, many tumor cells develop ways to inhibit this process. The upregulation of survival pathways and the downregulation of pro-apoptotic factors are primary examples of this. As a result, resistant tumor cells can evade death following radiation exposure. This characteristic makes apoptosis inhibition a critical area of study.
Understanding the molecular players involved in this regulation could lead to therapies that restore the sensitivity of cancer cells to induced death, thereby improving treatment efficiency.
Molecular Players Involved
Oncogenes and Tumor Suppressors
The interplay between oncogenes and tumor suppressor genes is fundamental to the development of cancer and its resistance patterns. Oncogenes, when activated, can drive the proliferation of tumor cells, while tumor suppressor genes are responsible for regulating cell growth and maintaining genomic stability.
A crucial aspect of this balance is how oncogenes can promote resilience against radiation through enhanced survival signaling. This becomes significant as these genes often lead to the activation of compensatory pathways in response to DNA damage.
Nevertheless, the challenge remains in targeting these processes effectively, as the loss of tumor suppressor function often accelerates resistance, complicating treatment outcomes.
Signaling Pathways
Signaling pathways facilitate cellular communication and play a critical role in how cancer cells respond to stress, including radiation. Pathways such as the PI3K/Akt and MAPK pathways are frequently implicated in promoting survival and growth in response to radiation.
Recognizing which pathways dominate in specific types of tumors can provide insights into their radioresistance mechanisms. Identifying and targeting these pathways could offer new avenues for treatment, primarily when combined with existing therapies.
However, there is a challenge in the simultaneous targeting of multiple pathways due to interconnected signaling networks. This complexity necessitates a careful approach to ensure that interventions do not inadvertently promote alternative survival strategies.
MicroRNAs
MicroRNAs are small, non-coding RNA molecules that play a role in regulating gene expression. They can affect various cellular processes, including responses to radiation. By modulating the expression of target genes, microRNAs can enhance or impair the sensitivity of cancer cells to radiotherapy.
Their capacity to fine-tune cellular responses positions them as potential biomarkers for predicting radiation resistance.
Yet, the dual role of microRNAs in both oncogenic and tumor-suppressive contexts complicates their application. One microRNA might promote resistance in one context while enhancing sensitivity in another, highlighting the need for thorough investigation into their roles across different tumor types.
This dynamic nature of molecular interactions reaffirms the necessity for continued research into radiation resistance mechanisms in cancer therapy.
The Role of the Tumor Microenvironment
The tumor microenvironment plays a significant role in cancer progression and treatment response. It consists of various cellular components, including immune cells, vascular cells, and stromal cells, all interacting with cancer cells. These interactions are crucial for creating a niche that can influence the behavior of the tumor. In the context of radiation resistance, the tumor microenvironment is essential as it can shield cancer cells from the damaging effects of radiation.
Understanding the components of the tumor microenvironment can help in developing strategies to enhance the effectiveness of radiotherapy. Notably, elements such as hypoxia and stromal interactions have been identified to contribute to the resistance observed in different cancers.
Impact of Hypoxia
Hypoxia, or low oxygen levels, is a frequent characteristic in tumors and significantly influences the efficacy of radiotherapy. Oxygen enhances the effects of radiation by increasing the production of reactive oxygen species that damage cellular components. In a hypoxic environment, cancer cells are more resistant to radiation; they can survive and exhibit altered metabolic processes. This phenomenon is crucial because many solid tumors have regions that are poorly oxygenated.
Recognizing hypoxia’s impact opens avenues for therapeutic interventions. Strategies may focus on reoxygenating tumors or developing hypoxia-activated drugs that selectively target cancer cells in low-oxygen zones.
Stromal Interactions
Stromal interactions within the tumor microenvironment significantly influence radiation responses through various mechanisms.
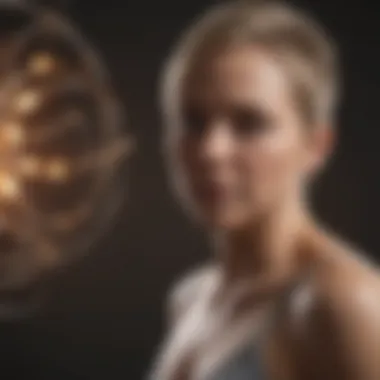
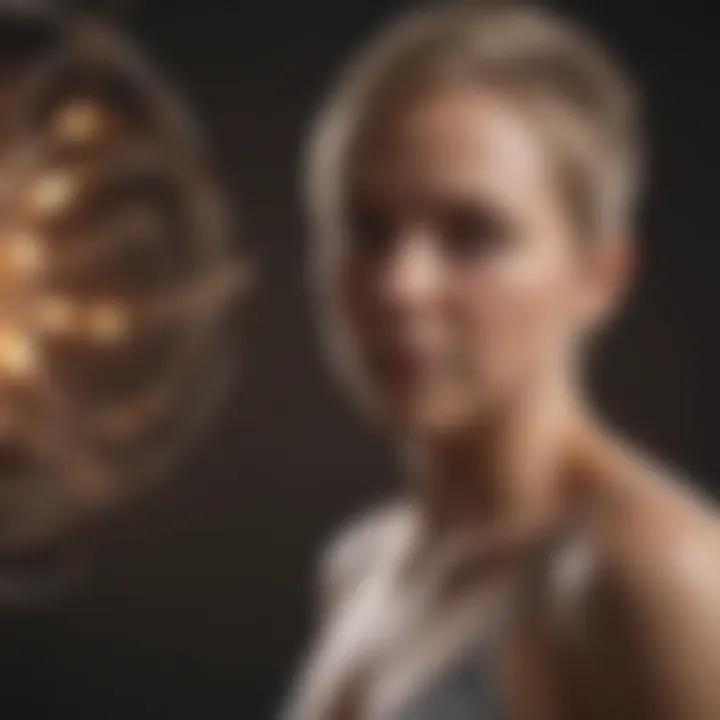
Fibroblasts
Fibroblasts are crucial components of the stroma. They secrete extracellular matrix proteins and growth factors that can enhance tumor survival and promote resistance to treatment. A key characteristic of fibroblasts is their ability to alter the local environment, making it more supportive for cancer cells.
Fibroblasts can create an environment that limits the effectiveness of radiation therapy. They can secrete signals that prompt cancer cells to evade radiation-induced apoptosis, leading to treatment failure. Understanding how fibroblasts function can provide insight into potential targets for intervention, making it a beneficial area of study in overcoming radiation resistance.
Extracellular Matrix Components
The extracellular matrix (ECM) serves as a scaffold in the tumor microenvironment and includes collagen, fibronectin, and other proteins. Its structure and composition can affect cancer cell behavior and response to radiation. One key characteristic of the ECM is its ability to facilitate communication between cells.
This arrangement can either support or hinder the effectiveness of radiotherapy. For instance, a dense ECM may prevent successful radiation penetration and limit the therapeutic dose received by tumor cells. At the same time, understanding ECM’s structure can lead to the development of therapies that effectively modulate the matrix, thereby enhancing radiation efficacy.
In summary, the tumor microenvironment is not merely a passive background but a dynamic entity that directly influences cancer therapy, particularly radiation treatment. Exploring the interactions among its components provides pathways to potentially overcome resistance.
By deepening our understanding of these biological aspects, researchers can devise new strategies that could lead to improved treatment outcomes.
Genetic and Epigenetic Factors
Understanding genetic and epigenetic factors is essential in the examination of radiation resistance in cancer. These elements play a crucial role in determining how cancer cells respond to radiotherapy. Genetic factors involve alterations in DNA that can lead to either increased resistance or heightened sensitivity to radiation. Conversely, epigenetic modifications can influence gene expression without altering the DNA sequence. Both aspects combine to create a complex interplay that significantly impacts treatment outcomes. By analyzing these factors, researchers can identify biomarkers for better prediction of radiotherapy efficacy, aiding in personalized cancer treatment strategies.
Genetic Mutations and Radiation Sensitivity
Genetic mutations are often at the forefront of radiation sensitivity discussions. They refer to permanent alterations in the DNA sequence of an organism. In cancer, specific mutations can enhance the ability of tumor cells to tolerate radiation. For instance, mutations in genes responsible for DNA repair, such as BRCA1 and BRCA2, can lead to persistent DNA damage after radiation, increasing the likelihood of resistance. Understanding these mutations is vital as they serve as potential targets for therapies aimed at increasing radiation efficacy.
Moreover, certain oncogenes can promote tumor survival after radiation exposure, leading to treatment failure. For example, mutations in the TP53 gene, which is involved in the regulation of the cell cycle and apoptosis, can result in robust tumor cell survival mechanisms. This connection emphasizes the necessity of advanced cancer genetic profiling to predict sensitivity and resistance more accurately.
Epigenetic Modifications in Cancer Cells
Epigenetic modifications represent another layer in the complexity of radiation resistance. These modifications do not change the DNA itself but alter the way genes are expressed. Mechanisms such as DNA methylation, histone modification, and non-coding RNA involvement are crucial in cancer biology. For example, hypermethylation of tumor suppressor genes can silence their expression, allowing cancer cells to evade the effects of radiation.
Additionally, alterations in histones can lead to chromatin remodeling, which may induce a state of chromatin compaction that inhibits DNA repair mechanisms. This limitation can enhance tumor cells' ability to survive while also avoiding apoptosis. Thus, targeting these epigenetic changes with specific drugs can potentially restore sensitivity to radiation therapy.
Resistance in Specific Cancer Types
Understanding radiation resistance in specific cancer types is crucial for enhancing the efficacy of radiotherapy. Not all cancers respond equally to radiation; hence it is necessary to identify the unique resistance mechanisms for each subtype. This consideration allows researchers and clinicians to tailor treatment plans more effectively, using targeted therapies that may enhance the response to radiation. Furthermore, knowing the resistance profiles can inform the development of better predictive biomarkers, leading to more personalized cancer treatment strategies.
Breast Cancer
Breast cancer exhibits several resistance mechanisms that can vary based on the tumor subtype. Triple-negative breast cancer, in particular, is known for its high levels of intrinsic radiation resistance. Mechanisms such as enhanced DNA repair capabilities and alterations in signaling pathways play significant roles in this resistance. For instance, the overactivity of homologous recombination repair systems allows these cells to better manage DNA double-strand breaks caused by radiation.
Moreover, the tumor microenvironment greatly influences radiation sensitivity in breast cancer. Tumor-associated macrophages and fibroblasts can secrete factors that promote cellular survival and resistance. Efforts to counteract these effects have led to strategies combining radiotherapy with agents that target these supportive cells, such as immune-checkpoint inhibitors or anti-inflammatory agents.
Lung Cancer
Lung cancer is another area where radiation resistance presents substantial challenges. Both non-small cell lung cancer and small cell lung cancer have demonstrated the ability to resist radiation treatment. A primary mechanism in these cancer types is related to the activation of specific signaling pathways like the PI3K/Akt/mTOR pathway. This pathway supports cell survival and proliferation, even in the presence of DNA damage induced by radiation.
Hypoxia, a common condition within tumors, further complicates radiation therapy in lung cancer. Low oxygen levels can reduce the efficacy of radiation, making tumors less responsive. Strategies focusing on reducing hypoxia, such as the use of hypoxic cell sensitizers, are being explored to improve treatment outcomes. Furthermore, the integration of different treatment modalities, including chemotherapy and targeted therapies, aims to dismantle resistance and enhance overall response rates.
Head and Neck Cancers
Head and neck cancers also demonstrate distinct patterns of radiation resistance, significantly influenced by their biological and molecular characteristics. The presence of mutations in genes such as TP53 can impair the ability of cancer cells to undergo apoptosis, leading to resistance. Additionally, many head and neck tumors show a unique interaction with their microenvironments, where stromal cells release factors that promote tumor survival.
There is also a growing recognition of the importance of strategies such as targeting the tumor stroma. These strategies aim to disrupt the supportive environment that bolsters cancer cell survival, thereby increasing their susceptibility to radiation. Recent research is focused on exploring combination therapies that include conventional radiotherapy and novel agents aimed at tackling the mechanisms of resistance found specifically within head and neck cancers.
Understanding the nuances of radiation resistance in specific cancer types is essential for the development of more effective treatment approaches.
By focusing on breast cancer, lung cancer, and head and neck cancers, it is clear that tailored strategies have the potential to significantly improve treatment outcomes and manage resistance more effectively.
Therapeutic Strategies to Overcome Radiation Resistance
Understanding how to combat radiation resistance is crucial in improving cancer treatment outcomes. This section evaluates various strategies that focus on enhancing the effectiveness of radiotherapy in resistant tumors. Successful therapeutic interventions can increase cell death rates and improve survival odds. Multiple strategies can tackle the different mechanisms of resistance, ultimately refining the overall treatment landscape.
Combination Therapies
Chemotherapy and Radiotherapy
Chemotherapy and radiotherapy are often used in tandem to maximize cancer treatment efficacy. Chemotherapy can sensitize resistant cancer cells to radiation. It targets rapidly dividing cells and can diminish tumor burden before irradiation. This reduces the volume of cancer cells, thereby enhancing the effect of radiotherapy.
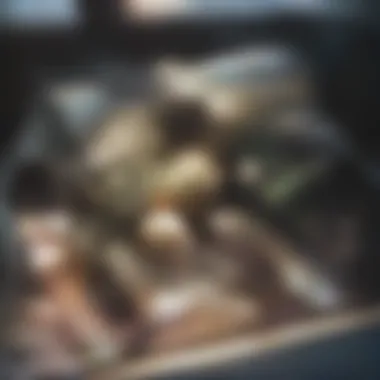
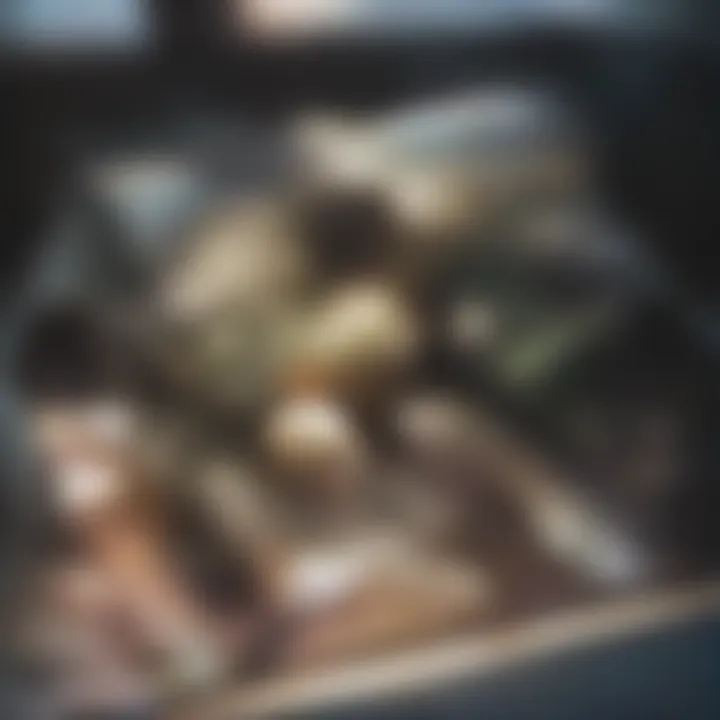
A key characteristic of this approach is its ability to exploit the timing of drug administration. The synchronization between chemotherapy and radiotherapy can prevent cell repair mechanisms from activating before radiation exposure. This characteristic makes combined modalities a popular choice among oncologists.
However, there are disadvantages. Patients may experience heightened side effects due to the combination treatment. Moreover, not all cancers respond uniformly to this therapy, which necessitates personalized treatment plans for effective management.
Immunotherapy Approaches
Immunotherapy is another innovative approach to surmount radiation resistance. By harnessing the body’s immune system, this technique can help identify and destroy cancer cells that evade traditional therapies. It has shown the ability to enhance the effects of radiotherapy, potentially leading to better outcomes in resistant cancers.
A major highlight is the induction of an immune memory response. This unique feature allows the immune system to recognize and attack residual cancer cells after radiation therapy. Given its adaptive nature, immunotherapy becomes a valuable addition to the oncological arsenal.
Nonetheless, immunotherapy is not without challenges. It can lead to autoimmune reactions, making it sometimes risky for patients. Different cancer types also exhibit varying responses to immunotherapy, complicating its implementation as a one-size-fits-all solution.
Targeting Specific Pathways
PARP Inhibition
PARP inhibition is a targeted strategy focusing specifically on cancer cells with defects in DNA repair mechanisms. This approach exploits the reliance of these cancer cells on PARP (Poly ADP-ribose polymerase) for DNA damage repair. By obstructing PARP, cancer cells struggle to recover from radiation-induced damage.
The critical aspect of PARP inhibition is its selectivity. It specifically targets tumor cells with homologous recombination deficiencies, a common trait in certain breast and ovarian cancers. This precision makes it a favorable choice for individualized treatment regimens.
However, while promising, PARP inhibitors may not be universal. Not all patients demonstrate significant efficacy from this targeting, necessitating further exploration to identify appropriate biomarkers.
Other Molecular Targeted Approaches
Other molecular targeted approaches encompass various strategies aiming at specific pathways involved in tumor survival and growth. These can include drugs targeting cell cycle checkpoints or signaling molecules critical for tumor resilience. The significance lies in its ability to disrupt the pathways that cancer cells exploit to resist treatment.
A key characteristic of these approaches is their adaptability. They can be tailored to target specific tumor biology, aligning treatment with individual patient profiles. This adaptability could lead to more effective personalized therapies.
However, the complexity of cancer biology presents challenges. Resistance mechanisms evolve, often rendering initially effective therapies less responsive over time. Continuous research is essential to identify emerging resistance pathways, ensuring these methods remain effective in countering radiation resistance.
"Employing these therapeutic strategies is vital for overcoming the persistent challenge of radiation resistance in cancer treatment. Continuous research and innovation in therapies can significantly impact patient outcomes."
Future Directions in Research
The exploration of radiation resistance in cancer is paramount for understanding how to enhance therapeutic efficacy. As cancer treatment evolves, the need to address resistance mechanisms becomes increasingly critical. Future research must leverage both technical advancements and biological insights. This will facilitate the development of tailored strategies to overcome resistance, leading to improved patient outcomes.
Emerging Predictive Biomarkers
Identifying predictive biomarkers is at the core of future cancer treatment strategies. Biomarkers can indicate how a patient will respond to radiotherapy, helping to personalize treatment plans. This is crucial for optimizing therapeutic regimens to enhance sensitivity and mitigate resistance.
Some potential biomarkers include:
- p53 mutations, which have shown varying impacts on response to radiation
- EGFR alterations in specific tumors that influence treatment outcomes
- MicroRNAs, which can modulate gene expression related to radiation sensitivity
Research into these biomarkers can clarify their roles in predicting treatment efficacy. Understanding their mechanisms of action can provide insights into how to modify treatment plans effectively.
Personalized Cancer Treatment Strategies
Tailoring treatment to individual patient profiles represents a significant advancement in oncology. This involves assessing the specific biological characteristics of tumors and their microenvironments. Personalized strategies may include:
- Utilizing genomic profiling to customize radiotherapy
- Integrating immunotherapy with personalized radiotherapy approaches
- Employing advanced imaging techniques to monitor tumor response
By focusing on personalized treatment solutions, clinicians can better navigate the challenges posed by radiation resistance. Identifying patients who are more likely to benefit from combination therapies can improve overall outcomes.
"Personalized treatment approaches have the potential to transform oncology by addressing the unique complexities of each patient's cancer."
Ending
The exploration of radiation resistance in cancer provides critical insights into how treatment efficacy can be compromised. This section is essential because it synthesizes the information discussed throughout the article and highlights the key points that need emphasis. Understanding the complexities of radiation resistance not only contributes to the field of oncology but also informs clinical practice in significant ways.
Summary of Key Findings
In reviewing the contents of this article, it is clear that there are multiple layers to radiation resistance:
- Biological Mechanisms: The cellular mechanisms like DNA damage repair pathways and signaling pathways play a main role in how cancer cells evade the effects of radiation.
- Tumor Microenvironment: Factors such as hypoxia and stromal interactions can further complicate the effectiveness of radiotherapy, leading to increased resistance.
- Genetic and Epigenetic Influences: Genetic mutations and epigenetic modifications contribute significantly to the variable responses seen in different cancer types.
- Therapeutic Strategies: Approaches like combination therapies and targeting specific pathways are essential for overcoming resistance, showcasing ongoing developments in treatment strategies.
- Emerging Research: Continuous investigation into predictive biomarkers and personalized treatments could shape the landscape of cancer care in the future.
Importance of Continued Research
The need for ongoing research in the realm of radiation resistance is paramount. Cancer is not static; the mechanisms that contribute to resistance evolve, so researchers must stay ahead of these changes. Investing in research can lead to:
- Development of Effective Therapies: By understanding the intricacies of how resistance occurs, researchers can develop targeted therapies that counteract these mechanisms.
- Personalized Treatment Plans: Greater insight into genetic and epigenetic factors may help tailor treatment plans more effectively to individual patients, improving outcomes.
- Improved Predictive Biomarkers: Identifying and validating biomarkers can help predict which patients will respond to radiotherapy and those who might require alternative strategies.
As the field progresses, it becomes increasingly important to integrate discoveries from basic science with clinical applications. Collaboration across disciplines will amplify the efforts in combating radiation resistance.
By continuing to delve deeper into these aspects, the medical community can better navigate the complexities involved and ultimately improve the survival rates and quality of life for cancer patients.