Real-Time qPCR: Techniques and Innovations in Biology
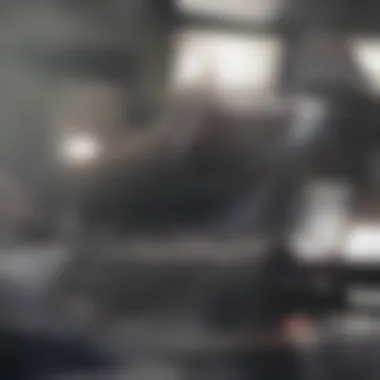
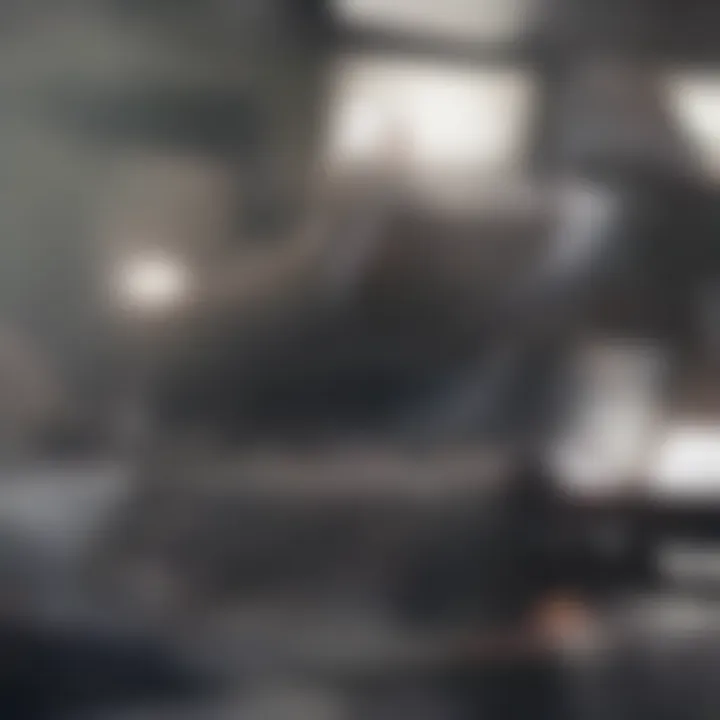
Intro
Real-Time Quantitative Polymerase Chain Reaction (qPCR) stands as a cornerstone technique in molecular biology. It allows for the detection and quantification of nucleic acids with precision and speed. The method has evolved significantly since its inception, adapting to the ever-changing landscape of molecular research and diagnostics. This article aims to dissect the intricacies of qPCR, providing a detailed exposition that highlights its techniques, applications, and recent innovations.
The qPCR method hinges on the amplification of DNA sequences, enabling researchers to measure specific nucleic acid targets in real-time. Unlike traditional PCR, where results are only visible at the end of the process, real-time qPCR allows for continuous monitoring of the reaction through fluorescent signals. This capability has transformed how quantitative analysis of gene expression and pathogen detection is performed.
With the goal of elucidating the multi-faceted aspects of this technology, we will explore the technical underpinnings of qPCR, the methodologies involved in executing experiments, and the various contexts in which this technique proves beneficial. The implications of recent advancements will also be addressed, keeping in mind the relevance of these developments to the future of molecular research.
Research Methodology
Description of Research Design and Approach
The methodology surrounding real-time qPCR is typically structured around well-defined protocols. The design often starts with the selection of target sequences, which may involve gene of interest or pathogens in clinical samples. Researchers must, therefore, consider factors such as specificity, efficiency, and reproducibility in their design.
Key components of the research approach include:
- Target Selection: The identification of specific genomic sequences that are relevant for the study.
- Assay Development: Designing primers and probes specific to the target sequences. This step is critical, as it directly influences the sensitivity and accuracy of qPCR results.
- Validation: Testing the developed assays to ensure their precision and reliability before conducting extensive experiments.
Materials and Methods Used in the Study
The materials employed in real-time qPCR vary but typically include:
- DNA or RNA samples: Isolated from biological specimens or generated through cell cultures.
- Primers: Short sequences that initiate the polymerase activity at the target site.
- Probes: Often labeled with fluorescent dyes that allow for the quantification of the amplified product during the reaction.
- Thermal cycler: A specialized instrument that facilitates the necessary temperature changes for DNA denaturation, annealing, and extension.
The methodology often entails the following steps:
- Sample Preparation: Extracting nucleic acids from the samples using kits or other methods.
- Reaction Setup: Combining the samples with primers, probes, and PCR reagents in a reaction vessel.
- Cycling Conditions: Programming the thermal cycler for appropriate denaturation, annealing, and extension cycles.
- Data Acquisition: Monitoring fluorescence during each cycle to quantify the nucleic acid.
By adhering to these methodologies, researchers can ensure that their qPCR results are both valid and replicable, reinforcing the reliability of their conclusions in the context of molecular biology.
"Understanding the methodology of real-time qPCR can significantly enhance the quality of molecular research and diagnostics."
Discussion and Interpretation
Interpretation of Results in the Context of Existing Literature
Once data collection is complete, interpreting results within the framework of existing literature becomes paramount. Comparing findings against previous studies can validate results or reveal discrepancies that warrant further investigation. The accuracy of quantification is critical; thus, understanding variability within assays can illuminate potential improvements in technique.
Implications for Future Research or Practical Applications
Future research may explore various avenues to enhance the efficiency of qPCR. Newer technologies could potentially reduce time and costs, making the method more accessible in various fields, from clinical diagnostics to environmental monitoring. There is also a growing interest in multiplexing capabilities—simultaneously analyzing multiple targets—which can drastically increase the throughput of relevant data.
In summary, a thorough understanding of real-time qPCR offers great potential for enhancing research outcomes across a myriad of applications.
Understanding Real-Time qPCR
Real-Time Quantitative Polymerase Chain Reaction, commonly known as real-time qPCR, stands as a cornerstone in molecular biology today. Its significance lies in the ability to quantify nucleic acids in real-time using fluorescent dyes. This offers a distinct advantage over traditional PCR methods that provide results at the end of the amplification process. The immediacy of data collection aids in real-time monitoring, which makes it invaluable for diagnostics, research, and various applications in biotechnology.
In this section, we delve deep into understanding the foundational aspects of real-time qPCR. By comprehending its basics, users can appreciate its nuances and applications better. The core components of the process reveal why it is often favored over older methodologies.
Basics of Polymerase Chain Reaction
The Polymerase Chain Reaction (PCR) is a technique allowing amplification of specific DNA sequences. It utilizes cycles of heating and cooling to denature DNA strands, anneal primers, and elongate new strands with a DNA polymerase enzyme. This cyclical process can result in exponential amplification of target sequences, enabling detection in minute quantities. Without the advancements inherent to real-time qPCR, fields such as genetics and forensics would face limitations in their analyses and diagnostics.
Mechanism of Real-Time qPCR
Real-time qPCR modifies traditional PCR by integrating a method for measuring fluorescent signals produced during DNA amplification. The mechanism involves three key processes:
Fluorescent dyes and probes
Fluorescent dyes or probes are critical for the visualization of the amplification process. Commonly used dyes include SYBR Green and TaqMan probes. These compounds emit fluorescence upon binding to double-stranded DNA. They enable quantitative measurement as the reaction progresses, providing a direct correlation between fluorescence and the amount of DNA present. Their non-specific binding, however, can lead to background signals, affecting accuracy.
Amplification and detection process
The amplification process in real-time qPCR is simultaneous with detection. Each cycle of qPCR amplifies the DNA and allows for the collection of fluorescence data. This real-time approach makes it possible to track the amount of DNA as it increases over time. The efficiency of this dual process caters to a wide range of applications from clinical diagnostics to environmental monitoring. Nonetheless, variability in efficiency may arise due to template quality or reaction conditions.
Cycle threshold (Ct) value determination
Cycle threshold (Ct) value reflects the cycle number at which the fluorescent signal exceeds background levels, indicating the presence of the target DNA. The lower the Ct value, the higher the initial amount of DNA in the sample. This quantification method is pivotal for comparing samples and determining relative expressions of genes. However, its interpretation necessitates careful consideration of reaction conditions, as they can differ widely across various settings.
"Real-time qPCR has virtually transformed molecular biology, making it possible to quantify genetic material with unprecedented speed and accuracy."
The analysis in this section illustrates how each component of real-time qPCR contributes to its functionality. Understanding its mechanisms not only aids in practical applications but also informs improvements in methodologies as innovations continue to emerge.
Essential Components of qPCR
In the realm of Real-Time quantitative Polymerase Chain Reaction (qPCR), the essential components play a critical role. Each element contributes uniquely to the accuracy, sensitivity, and reliability of the amplification process. Understanding these components allows researchers to optimize experiments and achieve precise results.
DNA or RNA Templates
The templates serve as the foundational material in any qPCR experiment. DNA or RNA samples derived from various sources, such as clinical specimens or cell cultures, are used for amplification. The selection of high-quality templates is vital, as degraded or contaminated samples can lead to unreliable data. It is important to quantify and assess the quality of the nucleic acids before proceeding with the experiment. Properly prepared templates improve reproducibility and enhance the reliability of the results.
Primers and Probes
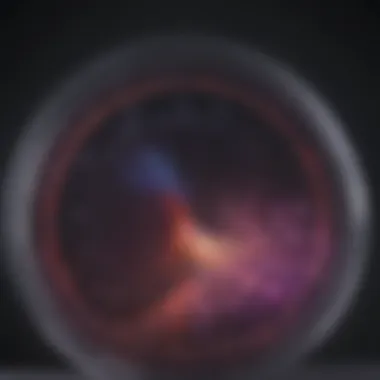
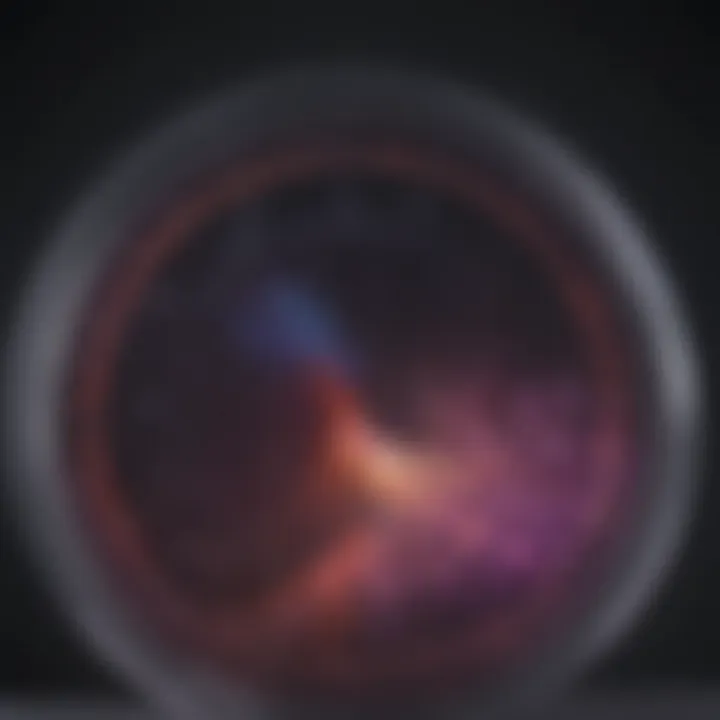
Primers and probes are crucial for the specificity and efficiency of qPCR. Their design and optimization influence the success of the technique significantly.
Design and optimization
Designing primers involves selecting sequences that ensure specificity for the target gene. The optimization process is vital because it allows adjustments in temperature and concentration, which can enhance the yield and efficiency of the reaction. Good primer design reduces the chance of non-specific amplifications, leading to cleaner data. The key characteristic of this process is its focus on achieving the best possible reaction conditions. It is beneficial primarily because optimized conditions can lead to improved yield and lower Ct values.
Types of primers
There are various types of primers, including forward, reverse, and more advanced formats like locked nucleic acids (LNA) or peptide nucleic acids (PNA). The choice of primers impacts the dynamics of the amplification. For example, LNA-based primers offer higher binding affinities, which can increase specificity. Each type of primer has its advantages and can be chosen based on the unique requirements of the experiment.
Specificity and efficiency
The specificity and efficiency of primers determine the accuracy of qPCR results. Specific primers amplify only the desired target sequences while minimalizing non-specific products. Efficient primers ensure optimal yield and minimize the formation of undesirable by-products. This makes specificity and efficiency beneficial for obtaining reliable quantification results in research and diagnostics. Overall, the balanced performance of specificity and efficiency is fundamental to the success of qPCR.
Reagents and Enzymes
Reagents and enzymes are the chemical backbone of qPCR. They ensure that the amplification process occurs efficiently and effectively.
Taq polymerase and alternatives
Taq polymerase is widely recognized for its robustness and high activity under a variety of conditions. However, there are several alternatives available, such as Pfu polymerase, that offer different fidelity and elongation rates. Choosing the right enzyme type can depend on the specific needs of the experiment. Each enzyme has its strengths; for example, high-fidelity polymerases are essential in applications requiring precise amplification without errors.
Buffer systems
Buffer systems provide the necessary environment for optimal enzyme activity. They help stabilize the pH and ionic strength, influencing the overall performance of the amplification process. Effective buffer systems, like the ones containing Tris and KCl, help improve enzyme performance and yield. Moreover, different buffers can suit different enzyme systems, enabling researchers to tailor experimental conditions for specific applications.
Dyes and fluorescent reporters
Fluorescent dyes, such as SYBR Green or TaqMan probes, are pivotal for quantifying the amplified products during qPCR. These dyes allow for real-time monitoring, making it possible to track the progress and output of the reaction. The key characteristic of these reporters is their ability to signal the presence of the target sequences by emitting fluorescence. While they greatly enhance sensitivity, each type has its own set of advantages and are chosen based on desired specificity and dynamic range in quantification.
The components of qPCR interact in complex ways to enable highly sensitive and specific detection of nucleic acids, making it a cornerstone technique in molecular biology today.
In summary, understanding the essential components of qPCR is vital for success in molecular biology research and diagnostics. Each element, from templates to reagents, plays a role that affects the overall performance of the technique.
Protocols and Methodologies
Protocols and methodologies in Real-Time qPCR are essential for achieving accurate and reproducible results. This section outlines different approaches used in the technique, shedding light on their significance and diverse applications. Codifying methodologies ensures standardization across experiments, thereby improving reliability. A well-established protocol can help in troubleshooting, enabling researchers to discern and address any anomalies observed during amplification.
Effective methodologies are pivotal when conducting experiments. They dictate the experimental design, from the choice of reagents to the final analysis of data. The development of standardized protocols enhances the comparability of results across studies, proving to be invaluable in fields from clinical diagnostics to agricultural biotechnology.
Additionally, specific protocols allow for the adaptability of the technique. They provide a foundation that can be tailored to individual research needs, balancing optimization and efficiency. Understanding these methodologies is vital for researchers aiming to leverage Real-Time qPCR in innovative ways.
Standard qPCR Protocol
The standard qPCR protocol serves as a cornerstone for many quantitative experiments. It involves several types of key steps:
- Preparation of Reagents: Reagents must be prepared carefully to ensure optimal reactions. This includes the setup of the Master Mix containing polymerase, primers, and other essential components.
- Template Preparation: The quality and quantity of the DNA or RNA template significantly affect qPCR results. It is crucial to quantify the nucleic acid accurately and to avoid degradation.
- Thermal Cycling: This step involves the repeated cycles of denaturation, annealing, and extension. Each cycle doubles the target DNA, with real-time detection of the amplification occurring at each cycle.
This standard protocol provides reproducibility and efficiency. It allows scientists to detect specific sequences with a high degree of sensitivity. Each researcher may adapt certain elements to fit specific experiments, but maintaining core principles is critical for success.
Reverse Transcription qPCR
Reverse Transcription qPCR (RT-qPCR) is crucial for studying gene expression. This method extends standard qPCR by incorporating a reverse transcription step that converts RNA into complementary DNA (cDNA). This addition enables researchers to analyze RNA transcripts effectively, providing insights into gene regulation and overall cellular activity.
- Isolation of RNA: High-quality RNA is essential. Researchers must ensure that the RNA is not degraded, as this affects the integrity of cDNA synthesis.
- cDNA Synthesis: The use of reverse transcriptase enzymes to synthesize cDNA from RNA is a critical step. Conditions must be optimized to ensure high efficiency and yield.
- Subsequent qPCR Amplification: After obtaining cDNA, standard qPCR protocols are followed. The cycle threshold values are measured to analyze gene expression quantitatively.
RT-qPCR provides detailed data that are indispensable for understanding biological processes at a transcriptional level. As such, it has become a mainstay in molecular research.
Digital PCR Techniques
Digital PCR represents a significant advancement in the realm of quantitative PCR technologies. Unlike traditional qPCR, digital PCR provides absolute quantification without the need for standard curves. This method partitions the sample into many separate reactions, allowing for accurate quantification of target nucleic acids.
- Partitioning the Sample: The sample can be divided into numerous small reaction chambers or droplets. This reduces the chance of cross-contamination and allows for individual reaction monitoring.
- Amplification and Detection: Each partition undergoes the amplification process independently. This method enables highly sensitive detection of low-abundance targets.
- Data Analysis: The end result indicates how many partitions contain the target sequence, producing a precise quantification of the original sample.
Digital PCR enhances sensitivity and offers greater precision. This technique is particularly beneficial in applications such as rare mutation detection and assessing viral loads in clinical samples.
"Understanding the methodologies behind Real-Time PCR is essential for advancing research and diagnostics in molecular biology. The choice of protocol can greatly influence the reliability of results."
Data Analysis and Interpretation
Data analysis and interpretation are fundamental components in the utilization of Real-Time qPCR. The accuracy of qPCR results highly depends on the proper analysis of the data collected during experiments. Data analysis not only ensures the validity of the experimental findings but also enhances the reproducibility of results by providing a clear framework for interpretation. Researchers must engage with various quantification methods, use appropriate software tools, and apply proper statistical analysis to draw reliable conclusions from their data.
Quantification Methods
Absolute vs Relative Quantification
Absolute quantification provides a specific number of DNA copies present in a sample, which is crucial for experiments requiring quantifiable data. This method involves a standard curve generated from known concentrations of a template. The strength of absolute quantification is that it permits a direct comparison among samples. However, it requires strict adherence to protocol, as discrepancies in standards can skew results significantly.
On the other hand, relative quantification compares the amount of target nucleic acid in a sample to a reference or control. This approach is often used to assess gene expression levels. The primary advantage of relative quantification is its simplicity and efficiency, making it a popular choice in many molecular biology laboratories. It can be performed with less expense compared to absolute quantification, which typically demands higher precision with an established standard.
Standard Curve Analysis
Standard curve analysis is an essential technique that helps quantify nucleic acids based on the PCR cycle threshold values. A standard curve refers to a graphical representation of the relationship between the concentration of DNA and the corresponding Ct values. This method is widely adopted because it assists in determining the initial amount of target sequence in unknown samples based on their Ct values.
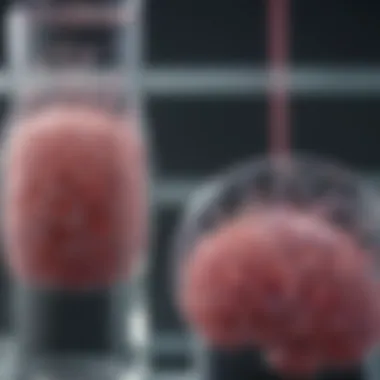
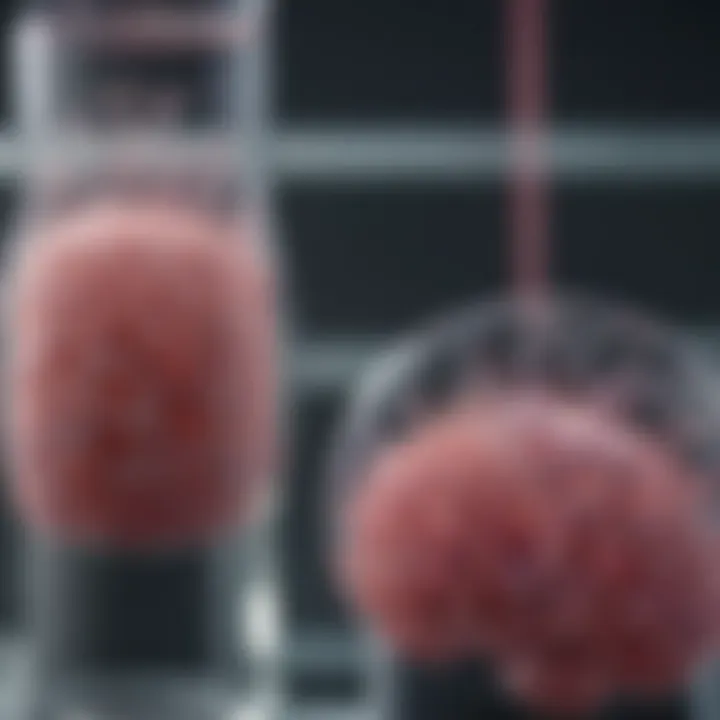
The unique feature of this analysis is its capacity to yield information on the efficiency of the PCR reaction. It allows for corrections of variations that may arise during the amplification process. One drawback of standard curve analysis is that it requires the generation of standard samples, which can be time-consuming and may introduce variability if not prepared correctly.
Software and Tools
Commonly Used Software
Data interpretation in Real-Time qPCR often relies on specific software tools designed for analyzing amplification data. Some widely used software includes Bio-Rad’s CFX Manager, Applied Biosystems’ Data Collection Software, and Qiagen’s QIAgility. Each of these tools offers user-friendly interfaces to facilitate data input, reaction setup, and analysis.
The key characteristic of these software solutions is the automation of data analysis, which reduces human error and increases reproducibility. They allow researchers to extract relevant information quickly and with minimal effort. However, there may be a learning curve involved when using advanced features, which can frustrate less experienced users.
Data Visualization Techniques
Data visualization techniques are essential for presenting results in an understandable format. Common methods include heat maps, scatter plots, and bar graphs, which represent the quantitative data from Real-Time qPCR results visually.
These techniques are beneficial as they enable researchers to spot trends, relationships, and outliers in the data easily. Furthermore, visually represented data is often more engaging for audiences and can aid in conveying complex information succinctly. Still, important details might get lost in overly simplified representations, necessitating a keen understanding of how to interpret such visuals accurately.
Statistical Analysis Approaches
Error Analysis
Error analysis plays a vital role in ensuring the reliability of Real-Time qPCR results. By identifying potential sources of error—such as pipetting inaccuracies, temperature variations during PCR, and reagent inconsistencies—researchers can mitigate risks and improve data quality. Error analysis contributes significantly to the overall credibility of findings, making it a crucial part of data interpretation.
The main advantage of including error analysis in research is that it strengthens the conclusions drawn from the data by providing insights into its reliability. However, it can be quite complex and requires a thorough understanding of statistical principles, which some researchers might find challenging.
Validation Measures
Validation measures are essential in confirming the results obtained from qPCR experiments. This includes verifying the specificity and sensitivity of the assay. Effective validation ensures that the reactions yield consistent results, which are reproducible across different experiments.
The key characteristic of validation measures is their ability to increase confidence in the experimental findings. However, implementing these measures can be resource-intensive and may require additional experimental setups, creating potential delays in research timelines.
Applications of Real-Time qPCR
Real-Time quantitative polymerase chain reaction (qPCR) has transformed multiple fields by enabling precise molecular detection and quantification. Its versatility is evident in clinical diagnostics, research, and agricultural biotechnology. By allowing real-time monitoring of the PCR process, it provides significant advantages over traditional methods. The applications not only underscore the method's utility but also highlight its influence on advancements in molecular biology.
Clinical Diagnostics
Pathogen detection
Pathogen detection using Real-Time qPCR is crucial in clinical settings. It can identify infectious agents quickly and accurately, which is important for prompt diagnosis and treatment. The key characteristic of this application is its sensitivity. It can detect even low levels of pathogens in a sample, making it a beneficial choice during outbreak investigations.
A unique feature of pathogen detection in this context is the use of targeting specific genetic sequences unique to pathogens. The advantages include swift results and the ability to differentiate between similar organisms, which is essential in developing effective treatment strategies. However, there are disadvantages such as the requirement for highly trained personnel and the risk of false positives in contaminated samples.
Genetic disorder testing
Genetic disorder testing is another significant application of Real-Time qPCR. This method allows for the rapid identification of genetic mutations associated with various hereditary diseases. One key characteristic is the method’s specificity. By designing primers that are unique to the altered sequence, it ensures higher accuracy in diagnosis. This specificity makes it a popular choice in clinical genetics.
A notable aspect of genetic disorder testing is how it enables early detection, which can be critical for timely intervention. Nevertheless, potential drawbacks include the emotional impact on patients and families receiving genetic information, as well as issues related to privacy and data security.
Research Applications
Gene expression analysis
Gene expression analysis benefits greatly from Real-Time qPCR techniques. It provides researchers with the ability to quantify mRNA levels, offering insights into gene activity under various conditions. Its key characteristic lies in the precision of quantification. Accurate measurement of gene expression is crucial for understanding many biological processes.
A unique feature of this application is how researchers can examine multiple genes simultaneously through multiplexing. This ability greatly enhances throughput in experiments. However, potential disadvantages include the complexity of primer design and the necessity for proper control samples to ensure data validity.
Genotyping and mutation detection
Genotyping and mutation detection are further applications where Real-Time qPCR excels. This method streamlines the identification of genetic variations in populations or individual samples. The key characteristic of this application is its capacity for high-throughput analysis, which allows for the examination of numerous samples in parallel.
The unique aspect of genotyping is its applicability in studying genetic diversity among organisms or analyzing traits. Advantages include cost efficiency and speed compared to traditional methods. On the downside, the method may not capture all variations, particularly in complex regions of the genome, which necessitates the use of complementary techniques in some cases.
Agricultural Biotechnology
Crops performance assessment
In agricultural biotechnology, Real-Time qPCR plays a vital role in crops performance assessment. It allows scientists to evaluate gene expression related to important traits, contributing to improved crop varieties. The key characteristic is its ability to analyze various factors affecting growth and yield.
One unique feature of this application is that it can facilitate marker-assisted selection in breeding programs. The advantages include accelerated breeding processes and the development of crops with desirable traits. However, one limitation may be the dependence on previously characterized genes, which could restrict innovation in crop improvement efforts.
Germplasm characterization
Germplasm characterization is another important application of Real-Time qPCR in agriculture. This involves assessing genetic diversity and the conservation of genetic resources. A critical aspect is obtaining detailed genetic information, which helps in making informed decisions for conservation and breeding.
The unique feature here is the detailed resolution of genetic background that contributes to biodiversity management. Benefits include the conservation of existing resources, ensuring the survival of various species. Nevertheless, challenges persist in terms of the necessary investment in technology and the skills required to perform such analyses effectively.
Real-Time qPCR's capacity for precise quantitative measurement and rapid result generation has positioned it as an indispensable tool across various domains.
Challenges and Limitations
The exploration of Real-Time qPCR brings forth a landscape filled with both promise and challenges. Recognizing these issues plays a critical role in advancing the technology while ensuring reliable results in research and diagnostics. In the following subsections, we will delve into the specific areas of concern that practitioners and researchers must navigate when utilizing Real-Time qPCR. By addressing these limitations, we can enhance the overall efficacy and reproducibility of the results.
Sensitivity and Specificity Issues
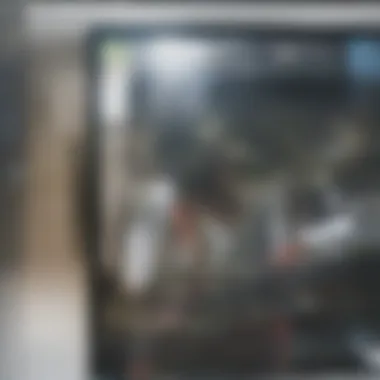
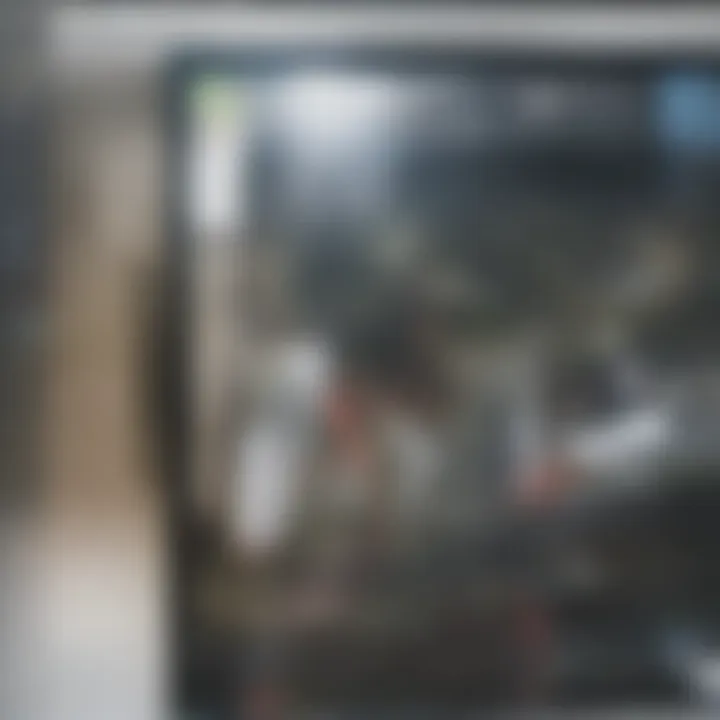
Sensitivity and specificity are two crucial parameters in any qPCR analysis. Sensitivity refers to the method's ability to detect low amounts of targets, while specificity describes how well the test can differentiate between similar sequences. In Real-Time qPCR, these factors can be challenged by a variety of elements.
To ensure accurate results, appropriate primer design is critical. Poorly designed primers can lead to non-specific binding, resulting in false positives. Also, variations in the quality of the template nucleic acid can influence sensitivity. It’s vital to optimize reaction conditions and confirm the specificity of primers through experiments like melt curve analysis. High sensitivity and specificity enhance the credibility of qPCR results, making them essential for both clinical and research applications.
Contamination Risks
Contamination is an ever-present risk in any molecular biology experiment, and Real-Time qPCR is no exception. The presence of even minute amounts of contaminating DNA or RNA can lead to significantly skewed results. Such contamination can arise from various sources: reagents, lab equipment, or even during sample handling.
To mitigate this risk, it is crucial to employ proper laboratory practices. Using dedicated equipment for qPCR workflows helps minimize cross-contamination. Implementing a strict workflow, where pre- and post-amplification samples are handled separately, can further reduce the possibility of contamination that may compromise the results.
Proper training and awareness among staff members about contamination risks can also make a substantial difference. Maintaining a clean working environment is essential for the integrity of Real-Time qPCR data.
Data Interpretation Complexity
Data interpretation in Real-Time qPCR is often complex and requires a thorough understanding of statistical principles. The results produced are not always straightforward and can be influenced by various factors, including the efficiency of amplification and the quality of the data collected.
It is essential to use appropriate statistical models for quantification. Misinterpretation can arise from discrepancies in data analysis settings or error in the baseline determination. Many researchers rely on software tools for data analysis, but varying algorithms and models can yield different interpretations of the same dataset. Therefore, it is vital to validate results through replication and peer review to ensure the conclusions are robust and reliable.
"Understanding these challenges is crucial for anyone working with Real-Time qPCR to improve the reliability of their findings and enhance the practicality of their applications."
In summary, while Real-Time qPCR technology offers significant advantages, being proactive about its inherent challenges can help researchers improve their methodologies and results in various applications. Addressing sensitivity and specificity issues, contamination risks, and data interpretation complexities is necessary for the credibility and success of Real-Time qPCR experiments.
Innovations in Real-Time qPCR
The realm of Real-Time quantitative polymerase chain reaction (qPCR) is dynamic and continuously evolving. Innovations in this field not only enhance the accuracy and efficiency of this vital technique but also open new avenues for research and diagnostics. Such advancements are critical for improving results, streamlining workflows, and addressing challenges that researchers face in various applications. As qPCR is widely applicable across scientific disciplines, understanding these innovations can bolster the methodological approaches taken by scientists.
Advancements in Reagents and Technologies
Next-generation sequencing applications
Next-generation sequencing (NGS) represents a significant innovation in molecular biology. It enables rapid sequencing of entire genomes and targeted areas. By integrating NGS with qPCR, researchers can improve the sensitivity of their assays. This integration allows for comprehensive data collection, reducing average turnaround time for results. A key characteristic of these applications is their ability to handle large volumes of data effectively. This makes them a popular choice for high-throughput environments where efficiency is vital.
A unique feature of NGS applications is their capacity for simultaneous analysis of multiple targets. This parallel processing capability is advantageous because it minimizes overlapping work and maximizes data output. However, the complexity of data generated can be a disadvantage, requiring sophisticated bioinformatics tools for proper analysis.
Novel fluorescent probes
The development of novel fluorescent probes adds another layer of innovation in real-time qPCR. These probes improve specificity and sensitivity beyond traditional methods. A noteworthy aspect is their ability to enable multiplex assays, allowing simultaneous detection of different targets within the same reaction. This characteristic enhances the throughput of experiments, making it a beneficial option for research that requires evaluation of multiple samples concurrently.
The unique feature of these probes lies in their advanced chemistries, which reduce background fluorescence and improve signal clarity. These enhancements facilitate more precise quantification. However, there can be challenges associated with their use, such as their higher costs compared to conventional probes, which can limit accessibility for some laboratories.
Integration with Other Techniques
Microarray integration
Integrating microarray technology with real-time qPCR allows for an enhanced understanding of gene expression patterns. This aspect facilitates the simultaneous analysis of thousands of genes, complementing the targeted approach of qPCR. The benefit of this integration lies in its capability to uncover complex biological interactions which can inform treatment strategies.
A key characteristic of this integration is the ability to validate findings from microarrays through qPCR. This validation process bolsters confidence in results, providing a more robust dataset. However, the complexity and cost of microarray technology can pose challenges, particularly for smaller labs or those with limited funding.
CRISPR applications
CRISPR technology is revolutionizing molecular biology, and its application in real-time qPCR is significant. The ability to create targeted modifications in DNA enhances the precision of experiments. CRISPR can be used to ensure validation of results from qPCR, allowing for more accurate gene editing studies.
The main characteristic of CRISPR applications is their adaptability. The technology can be tailored for various experimental needs, making it a versatile choice. However, a notable challenge lies in the need for meticulous design and execution, as off-target effects can complicate interpretation of results.
Innovations in Real-Time qPCR are not just about improved methods, but also about integrating new technologies that enhance research and diagnostics in a meaningful way.
Innovation in real-time qPCR continuously drives the field forward, providing tools and methods that expand possibilities within molecular biology. By understanding these advances and their implications, researchers position themselves at the forefront of scientific discovery.
Future Directions
The field of Real-Time quantitative Polymerase Chain Reaction (qPCR) is advancing rapidly, and understanding future directions is vital for researchers and practitioners alike. The continual evolution of qPCR techniques promises to enhance the precision and applicability of molecular biology. Emerging technologies and innovative methodologies are shaping its trajectory, thereby improving both research outcomes and clinical diagnostics significantly.
In terms of benefits, the future of qPCR holds potential for greater sensitivity and specificity. This is crucial for applications in fields such as genomics and personalized medicine. By leveraging advancements in hardware and reagents, scientists can better address issues related to contamination and data accuracy, which pose significant challenges in current practices. Additionally, enhanced automation and integration with other technologies may streamline labor-intensive processes, making them more efficient.
Furthermore, the consideration of regulatory changes is essential. As new applications for qPCR arise, regulatory bodies may introduce new guidelines. Adapting to these changes can foster public trust and improve the validation of molecular tests, which ultimately strengthens the impact of qPCR in clinical and research settings.
To summarize, the future directions in Real-Time qPCR concentrate on innovations that increase its effectiveness, accuracy, and field application. Engaging with these developments will allow the community to harness qPCR’s full potential while addressing its current limitations.
Emerging Trends
One of the most significant emerging trends in Real-Time qPCR is the integration of Artificial Intelligence (AI) and Machine Learning (ML) into data analysis processes. These technologies can help streamline data interpretation and improve predictive models used in a wide range of applications, conveying a more robust understanding of results.
Another notable trend involves the increase in multiplexing capabilities. Multiplex qPCR offers the ability to detect multiple targets in a single reaction. This method is particularly useful in clinical diagnostics, where saving time and samples is critical. Additionally, it minimizes the risk of contamination across multiple samples.
The use of CRISPR-based detection systems is also gaining traction. CRISPR provides higher specificity, making qPCR assays more accurate and reliable. The merger of these cutting-edge technologies signals a transformative period for molecular diagnostics.
Lastly, advancements in miniaturization and point-of-care testing are becoming prevalent. Portable and efficient qPCR devices will enable real-time monitoring and testing in various environments, such as remote locations, thereby expanding healthcare access.
Potential Research Areas
In exploring potential research areas, one key aspect is the refinement of quantitative methods in qPCR. Researchers are focusing on improving the standardization of protocols to ensure reproducibility and accuracy across various studies. This is essential for validating results, especially in clinical applications.
Furthermore, investigating alternative nucleic acid amplification techniques could lead to breakthroughs. Techniques such as isothermal amplification or loop-mediated isothermal amplification (LAMP) demonstrate promise as they do not require thermal cycling.
In addition, the exploration of new fluorescent dyes and probes can significantly enhance signal detection. Researchers are encouraged to examine non-traditional markers that could lead to improved sensitivity and specificity in various assays.
Research into the impact of biological variations on qPCR outcomes is another area of interest. Understanding how different biological factors influence results can help in designing more robust experiments, which is paramount in clinical research and diagnostics.
Lastly, examining the ethical implications and regulatory frameworks surrounding the extensive use of qPCR technologies is crucial. As its applications widen, addressing concerns related to data privacy, accuracy of diagnostic tests, and informed consent will ensure responsible advancement in the field.