Sodium Batteries for Cars: A Comprehensive Exploration
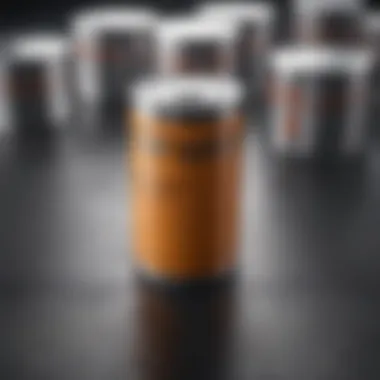
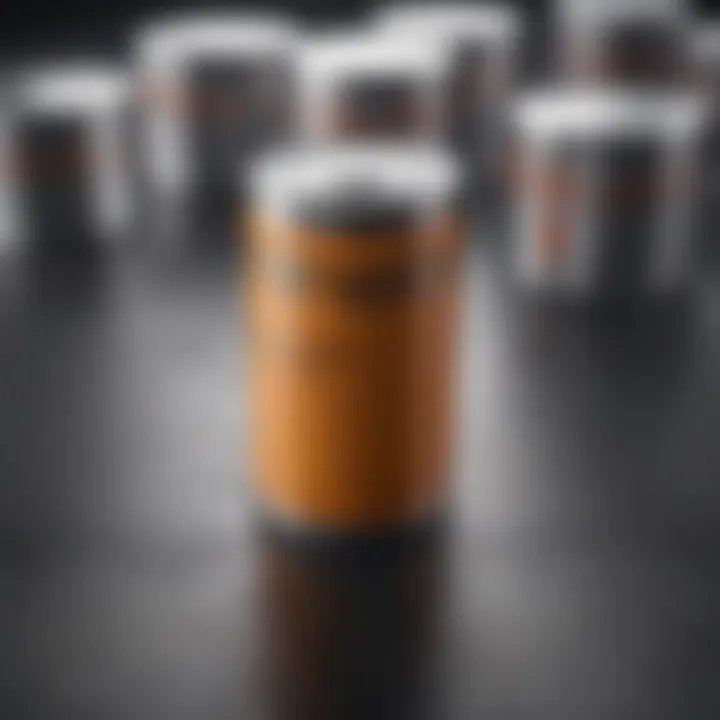
Intro
Sodium batteries have emerged as an intriguing alternative to traditional lithium-ion batteries, particularly within the context of automotive technology. This article seeks to provide a nuanced exploration of sodium batteries, emphasizing their unique chemical properties, potential advantages, and broader implications for sustainability in transport. Given the escalating demand for high-performance and environmentally friendly energy storage systems, sodium batteries present a critical area of exploration for researchers, manufacturers, and policy makers alike. Understanding the current landscape is vital, as the automotive industry is at a crossroads between innovation and environmental responsibility.
The growing interest in sodium batteries stems from several key factors. Firstly, the abundance and low cost of sodium compared to lithium offers a compelling economic advantage. Additionally, sodium's electrochemical properties are being optimized through research and development efforts. This opens a pathway for creating batteries that can compete with their lithium counterparts in terms of performance.
Another significant consideration is the environmental impact. As the world grapples with climate change, the need for sustainable energy solutions has never been more pressing. Sodium batteries, being largely based on materials that are more easily sourced and less harmful to the environment, offer a potential solution to some of the pressing issues faced by the automotive sector.
Through this article, readers will gain insights into the fundamental chemistry that underscores sodium battery technology, its current research landscape, and the engineering challenges it faces. Furthermore, the implications for future research and practical applications will be discussed in depth. We aim to create a comprehensive understanding of sodium batteries, paving the way for their possible transformative impact on the automotive industry.
Prelims to Sodium Batteries
Sodium batteries present a compelling alternative to lithium-ion technology in the field of automotive energy storage. This section highlights the urgency and relevance of developing sodium battery technology as the automotive industry seeks sustainable solutions. The focus is on understanding sodium batteries and identifying their potential benefits, which may reshape the future of electric vehicles.
Defining Sodium Batteries
Sodium batteries are energy storage devices that utilize sodium ions as the primary charge carriers. They operate through the movement of these ions between the anode and cathode during charge and discharge cycles. The basic components include a sodium-absorbing negative electrode, a sodium-based electrolyte, and a conducting positive electrode.
This configuration allows for energy release and absorption, similar to lithium-ion systems. However, sodium's abundance is a pivotal factor that distinguishes it from lithium. Sodium is, in fact, the sixth most plentiful element in the Earth's crust. This availability makes sodium batteries a potentially cost-effective and sustainable energy solution compared to their lithium-ion counterparts, ultimately presenting a more viable option for mass production in the automotive sector.
Historical Context
The exploration of sodium batteries dates back several decades; however, serious research accelerated only in the last twenty years. Initial developments can be traced to the search for alternatives to lithium, as concerns over lithium supply and environmental impact grew.
Early prototypes were characterized by low energy densities. These limitations hindered their practical applications in vehicles. However, with advances in material science and engineering, researchers have begun to address these issues. Notably, progress in sodium-ion chemistry has garnered renewed interest from development teams around the world.
Recent studies have shown that sodium batteries can approach the performance metrics of lithium-ion systems, particularly in terms of cycle stability and thermal safety. As a result, several automotive companies and researchers are now investigating sodium batteries as a potential game changer in energy storage, indicating a pivotal moment for this technology's adoption.
"With ongoing innovations, sodium battery technology holds the potential to address both performance and sustainability challenges faced by current energy systems."
In essence, the historical context illustrates the evolution of sodium batteries from theoretical concepts to a credible technology option in the contemporary automotive landscape. Understanding this trajectory is essential for comprehending the ongoing research efforts and potential applications of sodium batteries in vehicles.
Fundamentals of Sodium Chemistry
Understanding the fundamentals of sodium chemistry is crucial for grasping the entire ecosystem of sodium batteries. Sodium ions play a key role in energy storage and release, a process that defines how these batteries function. The atomic properties of sodium contribute to its potential as an alternative to lithium in energy applications. This section explores the electrochemical properties of sodium and compares its characteristics with lithium, aiming to shed light on the viability of sodium batteries in automotive applications.
Electrochemical Properties
The electrochemical properties of sodium are vital for its effectiveness in battery applications. Sodium, being in the same group as lithium on the periodic table, shares similar chemical behavior. However, the differences in their atomic mass and size influence their electrochemical behavior.
For sodium batteries, the typical reaction involves the movement of sodium ions from the anode to the cathode during discharge, which facilitates the flow of electrons through an external circuit. The energy density of sodium, while generally lower than lithium, can still provide a practical solution if managed effectively in chemistry and design.
The following points summarize the notable electrochemical properties of sodium:
- Ionic Conductivity: Sodium ions can move through electrolyte materials, ensuring efficient charge transfer.
- Electrode Potential: Sodium's standard electrode potential sits close to that of lithium, which means that the operating voltages are favorable for practical battery usage.
- Thermal Stability: Sodium batteries often exhibit better thermal stability, potentially enhancing safety when integrated into vehicle systems.
Sodium's ability to provide reliable energy transfer, along with its unique properties, renders it a compelling alternative for storage technologies.
Comparison with Lithium
The comparison between sodium and lithium is essential for positioning sodium batteries within the market. While lithium-ion technology has dominated the battery space, sodium batteries have emerged promising due to several distinct advantages.
- Abundance: Sodium is considerably more abundant and widely available than lithium, which may encounter supply chain constraints.
- Cost: The extraction and processing of sodium resources typically incur lower costs than their lithium counterparts. This may lead to reduced manufacturing costs for sodium-based batteries.
- Environmental Impact: The environmental footprint associated with sodium extraction is generally lower, presenting a more sustainable choice for future energy storage solutions.
Despite these favorable attributes, sodium batteries face challenges. For instance, the energy density does not yet match the capabilities of lithium-ion systems. Additionally, the cycle life and overall performance metrics need ongoing research and enhancement.
In summary, the fundamentals of sodium chemistry pave the way for ongoing advances in battery technology. Understanding the electrochemical properties and contrasts with lithium highlights both opportunities and obstacles for the evolution of sodium batteries in the automotive sector.
Advantages of Sodium Batteries
The conversation about sodium batteries is gaining traction, especially with their potential to disrupt the current automotive energy landscape. Understanding the advantages of sodium batteries is essential in evaluating their viability against established technologies. The following sections detail the key advantages—sodium's abundant supply, cost considerations, and the environmental impacts—that make these batteries appealing for future applications in automobiles.
Abundance of Sodium
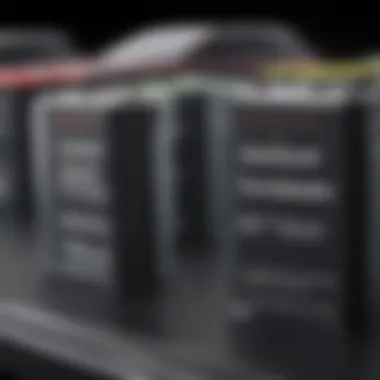
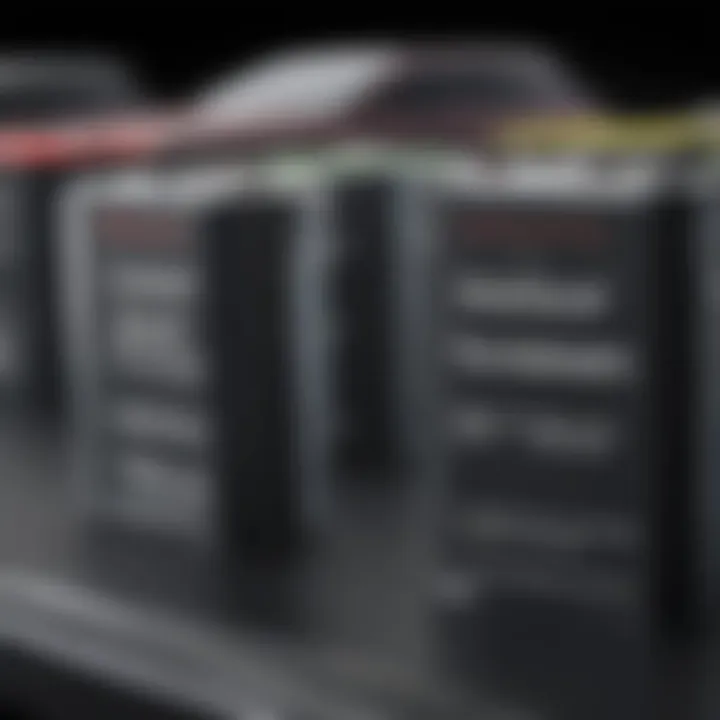
One of the most significant advantages of sodium batteries is the abundance of sodium itself. Unlike lithium, which is primarily extracted from a limited number of locations worldwide, sodium is plentiful and can be found in seawater and various minerals. This wide availability can lead to more stable and lower resource costs.
Sodium, being the sixth most abundant element in the Earth's crust, gives sodium batteries a sustainable edge. The increased geographic distribution of sodium resources may reduce geopolitical risks often associated with lithium mining. It can also facilitate more localized production, potentially decreasing transportation costs and emissions associated with battery manufacturing.
The ease of obtaining sodium could contribute to lower overall costs of battery production, expanding the usage of sodium batteries in various applications—not just vehicles.
Cost Considerations
Cost efficiency is another pivotal advantage of sodium batteries. As mentioned earlier, the extraction and processing of sodium are generally less expensive than lithium. This cost advantage may translate to lower retail prices for sodium batteries, making electric vehicles more accessible to a broader audience.
In addition, sodium batteries show promise for scalability. Current manufacturing techniques for sodium batteries are likely to be less complex than those used for lithium-ion batteries. Businesses could potentially build production facilities faster and with lower capital expenditures, allowing for a faster project turnaround.
However, it is important to consider that while initial costs may be lower, certain technological challenges must be overcome to achieve the desired performance metrics. Researchers are currently focusing on improving performance and energy density, which could impact the overall costs associated with sodium batteries in the future.
Environmental Impact
The environmental benefits of sodium batteries add another layer of appeal. Sodium extraction has lower ecological footprints when compared to lithium extraction, which often involves hazardous waste and significant water consumption. Sodium can be sourced with less environmental degradation, making it a more sustainable choice for energy storage.
Moreover, the recycling potential of sodium batteries is favorable. As research progresses, the development of effective recycling methods could lead to a closed-loop system, minimizing waste and encouraging the responsible disposal of battery components.
In summary, sodium batteries present a blend of favorable conditions: an abundant resource supply, cost advantages in manufacturing, and a lighter environmental footprint. These benefits support the argument for sodium batteries to play a significant role in the future of automotive energy storage.
"Sodium batteries may be crucial for achieving sustainable and affordable electric vehicles in the future."
When considering alternatives to current battery technologies, sodium batteries emerge as a serious contender with numerous advantages that warrant more in-depth evaluation.
Engineering Challenges
Understanding the engineering challenges surrounding sodium batteries is critical. These hurdles impact the design, efficiency, and ultimately the adoption of this technology in vehicles. While sodium batteries present advantages, they also bring significant obstacles that need innovative solutions. Engineers and researchers must address these challenges to tap into the full potential of sodium-based energy systems.
Energy Density Issues
A major challenge for sodium batteries is their energy density. Energy density refers to the amount of energy that can be stored in a given volume or mass. Sodium batteries typically exhibit lower energy density compared to their lithium-ion counterparts. This characteristic can lead to heavier battery packs, which affects vehicle performance and efficiency.
To understand this, it's important to recognize the chemistry involved. Sodium ions, while abundant, have larger atomic sizes compared to lithium ions. This difference influences how these ions move through the battery materials during charging and discharging. Slower ion transport can result in reduced capacity and efficiency. Addressing these energy density issues is necessary to make sodium batteries competitive for automotive applications.
Research into novel materials and design strategies is ongoing. Innovations include the use of advanced electrode materials that can enhance sodium ion mobility. By improving the architecture of sodium batteries, it may be possible to increase energy density substantially.
Cycle Life Limitations
Cycle life is another crucial consideration. It refers to the number of complete charge and discharge cycles a battery can undergo before its capacity falls below a specified threshold. For electric vehicles, having a long cycle life is essential for sustainability and user satisfaction. Unfortunately, sodium batteries often struggle with cycle life compared to existing technologies.
One reason for these limitations is the degradation of materials within the battery during operation. Repeated cycling can lead to structural changes in the electrodes, diminishing their effectiveness over time. This degradation can be caused by various factors, such as the formation of solid electrolyte interfaces or the loss of active material stored in the electrodes.
Ongoing research aims to understand these issues better. Solutions may involve engineering advanced electrode shapes or using protective coatings to minimize degradation during cycles. Furthermore, optimizing battery management systems can help mitigate cycle life limitations by improving the way batteries are charged and discharged.
"Advancing sodium battery technology involves overcoming critical engineering challenges that can reshape the automotive energy landscape."
In summary, addressing the engineering challenges of sodium batteries—particularly energy density issues and cycle life limitations—is vital. The progress in these areas will determine their viability as an alternative energy source in electric vehicles. As research continues, the ability to solve these problems will be central to the eventual adoption of sodium batteries in the automotive industry.
Current Research Trends
Current research in sodium battery technology is significant, especially in the context of automotive applications. With the rise of electric vehicles and the need for sustainable energy solutions, sodium batteries present a viable alternative to traditional lithium-ion systems. This section will explore innovations in material science and prototype development. These areas are critical, as they address the performance, cost-efficiency, and scalability of sodium technologies.
Innovations in Material Science
Research into material science is at the forefront of sodium battery advancements. Scientists are investigating various compounds that can improve the efficiency and longevity of sodium batteries. Key areas of focus include:
- Cathode Materials: Researchers are designing new cathode materials that enhance the battery's energy density. Transition metal oxides and polyanionic compounds are examples that show promise.
- Electrolytes: Advancements in electrolytes can reduce resistance during battery operation. Solid-state electrolytes and polymer gels are being explored as a means to improve conductivity and safety.
- Nanotechnology: The application of nanomaterials can lead to increased surface area in electrodes. This enhances the charge transfer rates, resulting in better overall performance.
By prioritizing these innovations, researchers aim to make sodium batteries more competitive with lithium-ion counterparts. A deeper understanding of materials leads to higher efficiency and lower costs.
Prototype Development
Prototype development is essential for transitioning sodium battery technology from laboratory to practical application. This phase involves creating working models that can be tested in real-world scenarios. Important aspects include:
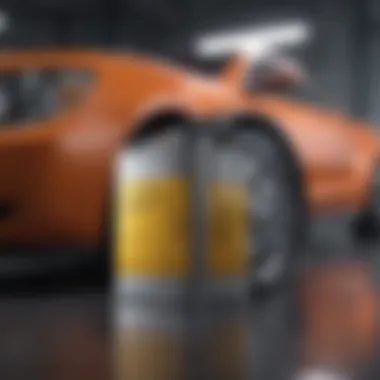
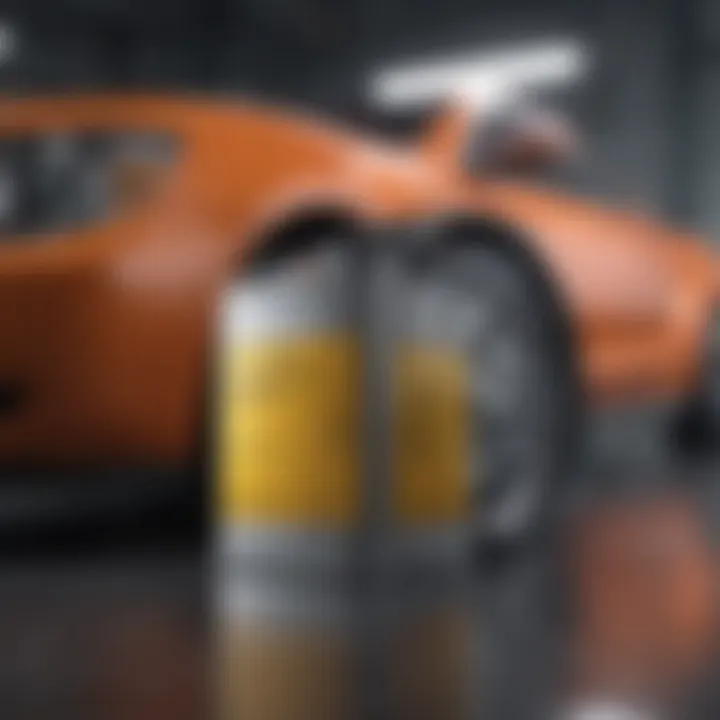
- Testing and Validation: Prototypes undergo rigorous testing to evaluate their performance under various conditions. Metrics like charge/discharge cycles, temperature stability, and overall lifespan are carefully measured.
- Collaborations: Many research institutions partner with automotive companies to develop prototypes. This collaboration aids in the practical application of research findings, facilitating the integration of sodium battery technology into vehicles.
- Iterative Design: The development process is iterative. Feedback from testing phases leads to design revisions that enhance battery performance and reliability.
Through effective prototype development, researchers are paving the way for sodium batteries to enter the market. They are continuously refining designs to mitigate challenges and meet industry standards.
Innovations in material science and effective prototype development are crucial for the successful deployment of sodium battery technology in vehicles.
The exploration of current research trends in sodium batteries reveals significant potential. Addressing material and prototype challenges can lead to widespread adoption within the automotive industry.
Sodium Battery Applications in Vehicles
The application of sodium batteries in vehicles signifies a shift away from traditional battery technologies. As the automotive industry seeks more sustainable and cost-effective solutions, sodium batteries emerge as a viable option. They offer several notable advantages, particularly concerning availability, cost, and environmental impact. These factors play a crucial role in the ongoing discussions around electric vehicles and hybrids.
Electric Vehicles
Electric vehicles (EVs) represent the forefront of automotive innovation, aiming to reduce greenhouse gas emissions and dependence on fossil fuels. Sodium batteries could enhance this movement. Compared to lithium-ion batteries, sodium batteries tend to be less expensive due to the abundance of sodium. This lower cost can lead to more affordable EVs for consumers, increasing accessibility to electric mobility.
Moreover, sodium batteries can have longer lifespans and provide stable energy output, which is essential for the efficiency of electric vehicles. The ability to endure a greater number of charge-discharge cycles can mitigate the costs associated with battery replacement. This durability can lead to less electronic waste, a priority for environmental considerations in automotive design.
Despite their benefits, sodium batteries also face challenges. Their energy density is lower than that of lithium, which impacts the driving range of EVs. This is a crucial factor that manufacturers must address to compete effectively with current lithium-ion technology. Additionally, ongoing research into improving energy density and performance is vital for broad adoption in the electric vehicle market.
Hybrid Systems
Hybrid vehicles combine an internal combustion engine with an electric propulsion system. The incorporation of sodium batteries can play a transformative role in this domain as well. Sodium batteries are naturally suited for operations requiring less overall energy but frequent, rapid discharge and recharge cycles. This characteristic aligns well with the functioning of hybrid systems, which need to manage energy efficiently during operation.
In hybrid vehicles, sodium batteries can act as a complement to conventional power systems, optimizing fuel usage and extending driving range. However, engineers and manufacturers must consider the specific needs of hybrid vehicles in terms of power output and efficiency. Balancing these variables can result in a hybrid model, that not only functions effectively but is also more sustainable.
As the automotive industry continues to evolve, manufacturers are exploring the potential of sodium batteries to fill this particular niche. Ultimately, the success of sodium batteries in both electric and hybrid vehicles will depend on overcoming existing technical hurdles and establishing a robust production framework.
"Sodium batteries present a cost-effective and sustainable alternative, potentially revolutionizing how we approach energy storage in automobiles."
This exploration into sodium battery applications is critical for understanding their role in future vehicle technologies. By addressing the unique benefits and challenges associated with these batteries, manufacturers and consumers can make informed decisions regarding the next generation of automotive energy solutions.
Manufacturing and Production
Manufacturing and production of sodium batteries play a crucial role in determining their viability as a mainstream energy storage solution for vehicles. This industry encompasses various aspects, including the sourcing of materials, the processes employed in battery assembly, and the scale at which these batteries can be produced. Understanding these elements is vital for the successful integration of sodium batteries in the automotive sector.
Supply Chain Considerations
The supply chain for sodium batteries is significantly different from that of lithium-ion batteries. Sodium, being abundant and widely available in the earth’s crust, offers unique advantages. However, there are also challenges in sourcing and processing sodium for high-performance batteries.
- Raw Material Availability: Sodium is more plentiful than lithium. Its availability can lead to fewer geopolitical risks associated with sourcing materials. However, efficient extraction and processing techniques are still necessary to ensure consistent quality.
- Component Sourcing: Besides sodium, other materials such as conductive additives and separators must be sourced. The quality of these components influences the overall performance of the batteries.
- Logistical Coordination: As manufacturers aim to scale production, logistics becomes a hurdle. Coordinating the transport of raw materials and finished products is essential to optimize the supply chain.
Adapting to these considerations will help manufacturers streamline production and minimize costs. Moreover, building strong partnerships with suppliers will enable manufacturers to secure reliable streams of necessary materials.
Scalability Challenges
Scaling production of sodium batteries is accompanied by a series of challenges that need careful attention. The transition from laboratory prototypes to mass production is often fraught with hurdles that can impede development.
- Production Techniques: Existing lithium-ion production techniques may not transfer directly to sodium batteries. Adjustments in manufacturing processes and technology are required to cater to sodium's unique chemical properties.
- Quality Control: As production scales up, maintaining consistent quality becomes difficult. Battery performance is highly dependent on precise material properties. Any variance in materials can lead to performance degradation, impacting adoption rates.
- Cost Efficiency: Scaling production often requires significant initial investment. Manufacturers need to find ways to reduce costs while still meeting the demand for high-quality sodium batteries. Achieving economies of scale is critical.
To address these scalability challenges, ongoing research and investments in innovative manufacturing technologies will be necessary. This way, manufacturers can expand capacity without compromising performance.
Sodium Batteries vs. Other Technologies
The comparison of sodium batteries with other emerging technologies is essential in understanding their place in the automotive landscape. As the market shifts towards sustainable solutions, knowing how sodium batteries stack up against alternatives helps evaluate their potential impact. This section includes a detailed examination of sodium batteries in relation to lithium-ion technology and solid-state batteries. These comparisons reveal not just the strengths, but also the weaknesses of sodium batteries in practical applications.
Comparison with Lithium-Ion Technology
Lithium-ion batteries have been the dominant force in energy storage for vehicles. Their popularity stems from high energy density, long cycle life, and performance reliability. However, sodium batteries present several competitive advantages worth considering.
- Availability of Materials: Sodium is more abundant and less expensive than lithium. This lower material cost can significantly affect overall battery pricing in large-scale applications.
- Environmental Impact: The mining and processing of lithium involve considerable environmental damage. In contrast, sodium extraction is less harmful. Thus, sodium batteries can be a more sustainable option in the long run.
- Thermal Stability: Sodium batteries often exhibit better thermal stability compared to lithium-ion counterparts. This characteristic can enhance the overall safety profile in automotive applications where overheating and fires are potential risks.
Despite these advantages, there are notable challenges. Sodium batteries struggle with lower energy density, which affects range and performance, particularly in electric vehicles. These limitations must be addressed thoroughly before they can compete effectively with lithium-ion technologies.
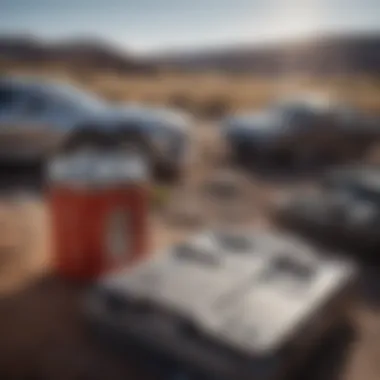
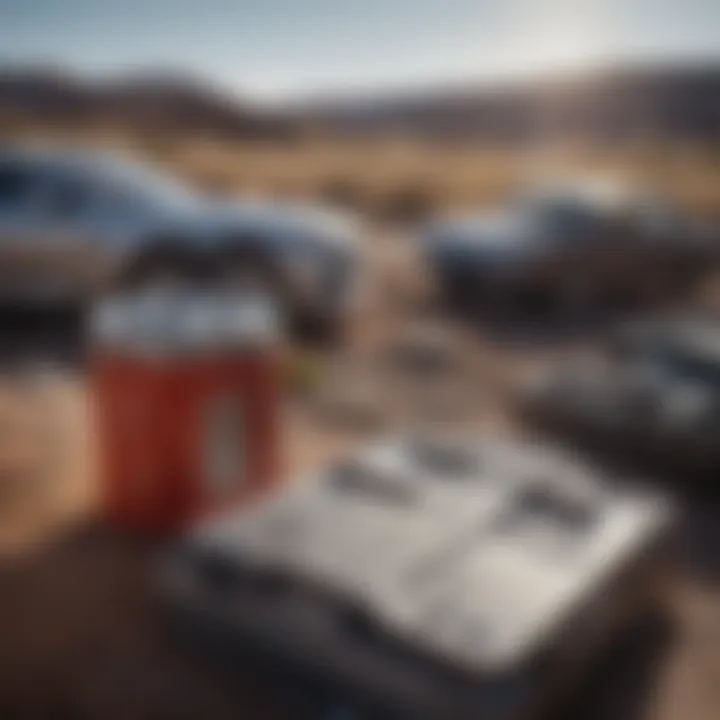
Positioning with Solid-State Batteries
Solid-state batteries represent the next frontier in battery technology, promising improved safety, energy density, and durability. Sodium batteries occupy an interesting position relative to solid-state solutions.
- Market Readiness: Currently, solid-state technology is still in the development stage with limited commercial viability. Sodium batteries, being in various stages of commercial testing, can fill the gap in the interim period as automakers transition to more advanced battery solutions.
- Cost: Solid-state batteries are generally more expensive to produce due to complex materials and manufacturing processes. Sodium batteries, with their cheaper components, can provide a cost-effective alternative until solid-state technology becomes more affordable.
- Applications in Hybrid Systems: While solid-state batteries are often seen as the future, sodium batteries may find their niche in hybrid systems where cost-efficiency and moderate performance are acceptable.
Sodium batteries offer a plausible route to greener technology in the automotive sector, potentially transforming energy storage solutions.
Regulatory Landscape and Industry Standards
Understanding the regulatory landscape and industry standards surrounding sodium batteries is crucial for their successful integration into the automotive sector. Regulations can profoundly affect how quickly and widely sodium batteries are adopted in vehicles. As manufacturers push to meet consumer demands, they also navigate a complex web of local and international rules. Compliance with these directives helps ensure safety and promote consumer confidence. Furthermore, adherence to industry standards can facilitate interoperability and enhance the overall market appeal of sodium battery technology.
Government Regulations
Government regulations play a pivotal role in the development and deployment of sodium batteries for automotive applications. These regulations primarily focus on ensuring safety, environmental protection, and standards for performance. Various agencies, such as the Environmental Protection Agency (EPA) in the United States and equivalent bodies in other countries, set forth requirements that manufacturers must adhere to.
Key considerations include:
- Emissions standards: Regulations often dictate acceptable levels of pollutants released during production and usage.
- Recycling protocols: Governments are increasingly mandating recycling to reduce environmental impact.
- Testing and certification: Before sodium batteries can be commercialized, they must undergo rigorous testing to meet safety and efficiency benchmarks.
These government regulations not only protect end-users but also encourage manufacturers to innovate. \n
Safety Standards
Safety standards specifically address the reliability and risk management of sodium battery technology. Ensuring that these batteries can operate safely under different conditions is crucial for widespread acceptance. Adherence to established safety protocols also minimizes the risk of incidents, which is fundamental when dealing with any energy storage system.
Important safety standards include:
- Thermal stability: Standards assess the temperature ranges within which sodium batteries must operate safely.
- Electrical integrity: Safety assessments ensure that batteries can handle short-circuits and other electrical malfunctions without catastrophic failure.
- Impact resistance: Tests determine how well batteries withstand physical stress, such as crashes or impacts, ensuring they remain safe during accidents.
Compliance with rigorous safety standards builds trust among consumers and regulators alike, paving the way for the broader adoption of sodium battery technologies.
In summary, navigating the regulatory landscape and adhering to industry standards are essential for the successful integration of sodium batteries into the automotive industry. As these technologies evolve, ongoing dialogue between manufacturers and regulatory bodies will enhance the development of supportive policies and frameworks that foster innovation and ensure safety.
Future Prospects of Sodium Batteries
The future of sodium batteries holds significant promise for the automotive industry, positioned as a critical alternative to traditional lithium-ion technology. This section explores various elements that underline the significance of sodium batteries, focusing on market predictions and technological advancements. Understanding these facets may guide stakeholders in making informed decisions regarding investments and developments in battery technology.
Market Predictions
Market predictions suggest a growing acceptance of sodium batteries in the automotive sector. Analysts estimate that by 2030, sodium batteries could capture a substantial share of the energy storage market, especially in electric vehicles. Factors contributing to this potential include:
- Cost Competitiveness: As sodium is more abundant and less expensive than lithium, production costs for sodium batteries are likely to decrease over time.
- Diverse Applications: Sodium batteries show versatility for use not only in electric vehicles but also in hybrid systems and energy storage solutions for renewable energy sources.
- Policy Shift: Governments worldwide are introducing policies favoring sustainable technologies, leading to increased adoption of sodium batteries. These policies create a conducive environment for innovation and investment.
Predictions vary, but key analysts believe that the sodium battery market could reach several billion dollars within the next decade. Additionally, competition with lithium-ion batteries will intensify, leading to innovations that may further enhance the effectiveness of sodium batteries.
Technological Advancements
Technological advancements are crucial to the future success of sodium batteries. Continued research is taking place to overcome current limitations, paving the way for practical applications. Significant areas of focus include:
- Material Innovations: Researchers are investigating new materials, such as advanced sodium-ion cathodes and anodes, to improve energy density and performance.
- Electrolyte Optimization: Developing efficient and safe electrolytes is vital. The goal is to enhance conductivity and stability while reducing flammability risks.
- Scaling Up Production: As demand rises, scalable manufacturing processes must be developed. Techniques that allow for rapid production can help meet market needs effectively.
"Technological advancements in sodium battery chemistry are crucial to unlocking the true potential of this alternative technology in automotive applications."
The intersection of these innovations will likely lead to better performance characteristics, longer life cycles, and increased safety profiles for sodium batteries. As these technological frontiers are explored, their successful implementation may not only benefit the automotive industry but also contribute to broader advancements in energy storage and sustainability.
Finale
The conclusion of this article serves as a vital summarization of key insights into sodium batteries. This technology represents a significant advancement in the field of energy storage, particularly for automotive applications. With rising concerns about the sustainability and cost of lithium-ion batteries, sodium batteries can potentially fill this gap. Here, I will highlight specific elements that underpin this promising technology.
Summary of Findings
Throughout the article, we have explored multiple facets of sodium batteries. Some of the main findings include:
- Sodium's abundance provides a renewable resource for battery technology.
- Cost-effectiveness positions sodium batteries as a competitive alternative to current technologies.
- Environmental impacts are notably lower, which fosters a more sustainable approach to energy storage in vehicles.
- Engineering challenges remain, particularly regarding energy density and cycle life. However, ongoing research shows progress in overcoming these barriers.
These points illustrate that sodium batteries have the potential to reshape the automotive energy storage landscape. As research continues, these technologies might not only become viable but essential.
Final Thoughts on Adoption
Adopting sodium battery technology in vehicles hinges on several considerations. First, industries must prioritize overcoming engineering challenges to improve performance metrics. Safety regulations and standards need to be established to ensure consumer confidence. Finally, collaboration among scientists, manufacturers, and policymakers will be essential in scaling production and meeting market demands.