Exploring T7 Polymerase Buffer: Components and Applications
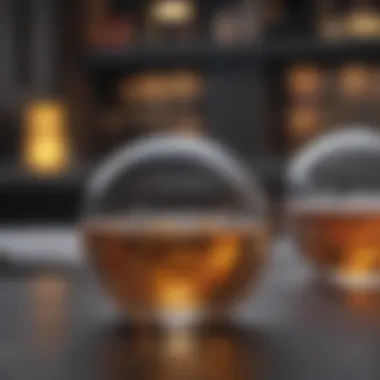
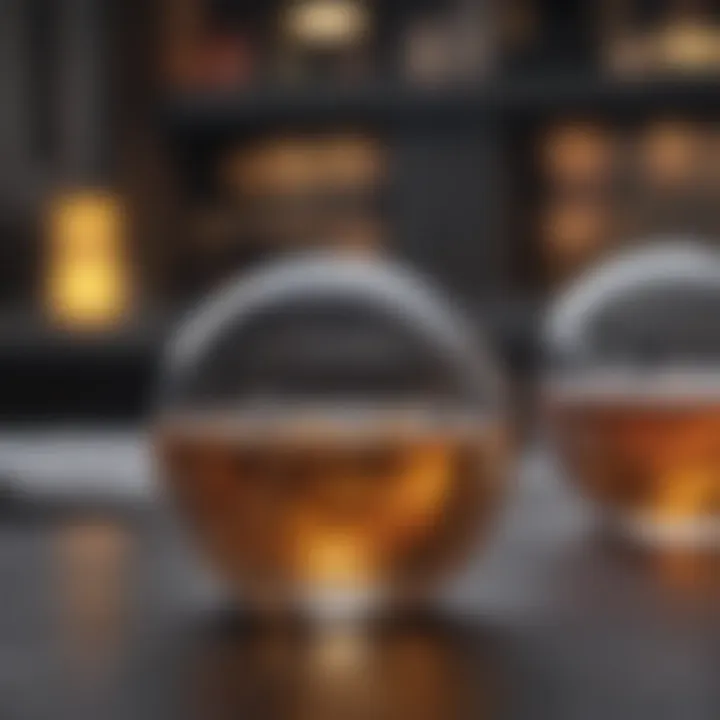
Intro
T7 polymerase buffer plays a crucial role in molecular biology. It enhances the efficiency of T7 RNA polymerase, which is essential in synthesizing RNA from a DNA template. Researchers rely on this buffer not only for its supportive role in transcription but also for its adaptability in various applications. Understanding its components, preparation, and practical uses is vital for anyone involved in genetic studies, diagnostics, or biotechnological advancements.
Research Methodology
In exploring T7 polymerase buffer, a systematic and thorough approach is required to provide meaningful insights into its effectiveness and applications.
Description of Research Design and Approach
The research design focuses on examining the biochemical properties of T7 polymerase and how its performance varies with different buffer compositions. This involves both experimental and comparative analyses. A series of assays are conducted to evaluate the transcription efficiency across different conditions. This approach permits an understanding of the underlying mechanisms and advantages of using T7 polymerase buffer in various settings.
Materials and Methods Used in the Study
The main materials include:
- T7 RNA polymerase: The enzyme used for RNA synthesis.
- DNA template: The source material for transcription.
- Buffer components: These typically include Tris, NaCl, MgCl2, and dNTPs.
- Reaction tubes: Used to conduct the assays.
- Incubator: To maintain optimal temperature for enzyme activity.
Procedures involve mixing the components in specific ratios and assessing the transcription product through electrophoresis. This process confirms the efficiency and reliability of the polymerase buffer.
Discussion and Interpretation
The findings from the assessment of T7 polymerase buffer underscore its importance in molecular biology. In the context of existing literature, the results align with previously documented studies emphasizing the significance of buffer composition in enzymatic reactions.
"The efficiency of T7 polymerase is significantly influenced by the buffer system, which determines not only enzymatic activity but also stability."
The implications for future research are substantial. Researchers can explore newer formulations of buffers that enhance transcription efficiency, reduce costs, and streamline workflows. Comparing T7 polymerase buffer with alternative buffers can lead to improved protocols in laboratories, fueling advancements in genetic engineering and diagnostics.
Ultimately, understanding the finer details of T7 polymerase buffer will empower scientists to make informed decisions about the tools they use, maximizing the reliability and success of their experiments.
Prelude to T7 Polymerase
T7 polymerase serves a crucial role in the realm of molecular biology, offering an efficient mechanism for synthesizing RNA. Its specificity and robust activity make it a staple in various applications, from gene expression studies to producing RNA for therapeutic use. This section delves into the fundamental aspects of T7 polymerase, providing readers with essential insights into its function and significance in research and biotechnology.
Overview of T7 Polymerase
T7 polymerase is a DNA-dependent RNA polymerase derived from the bacteriophage T7. It transcribes DNA into RNA, operating with high fidelity and speed. This polymerase recognizes specific promoter sequences, allowing for precise initiation of transcription. The enzyme requires a suitable buffer environment to maintain optimal activity, which is where T7 polymerase buffer comes into play. Components of the buffer are critical, as they help stabilize enzymatic reactions and create ideal conditions for nucleic acid synthesis. T7 polymerase has become essential in laboratory protocols, particularly in applications like in vitro transcription, where the accuracy of RNA synthesis directly impacts the quality of downstream applications.
Historical Context
The discovery of T7 polymerase marked a significant advancement in molecular biomechanics. Initially characterized in the 1970s, this molecule emerged as a powerful tool for genetic research. As comprehension of cellular mechanisms deepened, T7 polymerase proved beneficial for various laboratory techniques, especially with the rise of recombinant DNA technologies. Over the years, researchers recognized its versatility and efficiency, which led to developments in buffer composition tailored for optimized enzyme performance. This historical journey emphasizes the relevance of T7 polymerase in modern scientific applications, illustrating how foundational research has evolved into sophisticated methodologies seen in current laboratories.
"T7 polymerase is not just an enzyme; it is a cornerstone of modern molecular biology, foundational in both academic research and industrial applications."
Understanding T7 polymerase is essential for anyone involved in genetic research, diagnostics, or biotechnological applications. Its historical significance, coupled with its current functionality, positions it as a pivotal element in the toolkit of researchers and professionals alike.
Significance of T7 Polymerase Buffers
T7 polymerase buffers hold great significance in the realm of molecular biology. These buffers serve as the foundation for reactions conducted with T7 polymerase, thus underscoring their role in essential processes like nucleic acid synthesis. Their importance is multifaceted, touching on various aspects of research, diagnostics, and biotechnology applications. The components within these buffers are carefully selected to create an optimal environment for enzymatic activity.
Role in Nucleic Acid Synthesis
The primary role of T7 polymerase buffers is to facilitate the synthesis of RNA and DNA. The specific components included in the buffer are designed to maintain the correct pH and ionic strength, which are crucial for enzyme activity. T7 polymerase functions optimally under particular conditions, and the buffer helps achieve these. For instance, the presence of magnesium ions is essential because they act as cofactors that stabilize the enzyme-substrate complex, thereby promoting efficient transcription.
The balanced conditions provided by the buffer allow for the accurate copying of genetic material. This reliability in synthesis is vital for applications that depend on high yields of specific nucleic acid sequences.
Additionally, during the synthesis process, any variations in buffer composition can greatly affect the efficiency of T7 polymerase. The correct formulation ensures that nucleotides, which are the building blocks of RNA and DNA, are readily available for incorporation into the growing chain. A well-constructed buffer not only enhances output but also minimizes errors during the synthesis, which is paramount for research requiring precision, such as in cloning experiments or the preparation of probes.
Importance in Research and Diagnostics
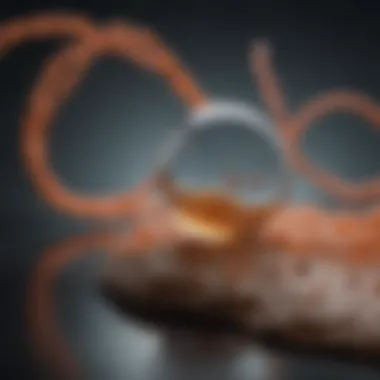
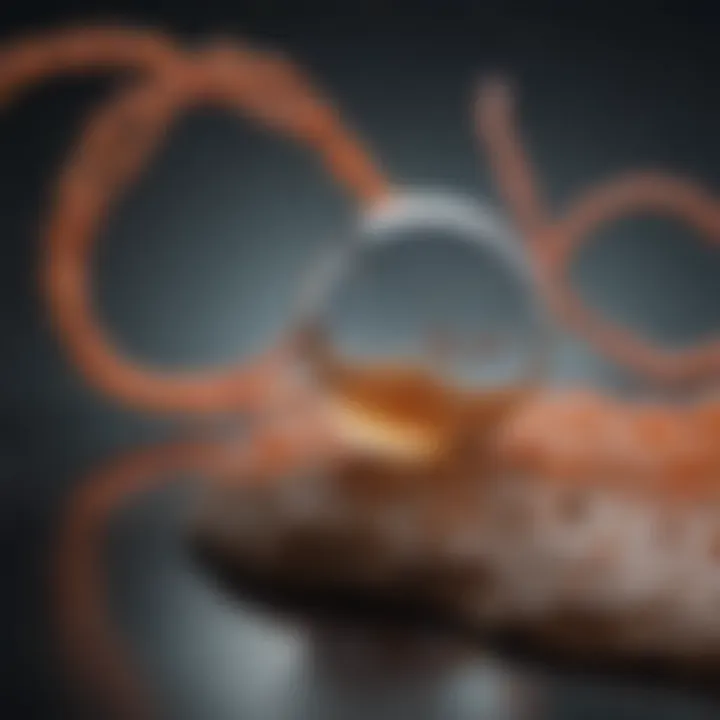
In addition to their role in nucleic acid synthesis, T7 polymerase buffers are also integral to research and diagnostic applications. In research settings, the buffers are fundamental in in vitro transcription. This process allows for the generation of large amounts of RNA, which can be used in various downstream applications, including gene expression studies and the development of RNA-based therapeutics.
In diagnostics, the accurate synthesis of nucleic acids using T7 polymerase buffers can assist in the detection of pathogens, genetic mutations, and other biomarkers. By ensuring high fidelity during transcription, researchers can obtain reliable data. This reliability ultimately contributes to better understanding disease mechanisms and the development of targeted treatments.
"The precision in nucleic acid synthesis provided by T7 polymerase buffers is essential for groundbreaking research and innovative diagnostic techniques."
Moreover, the versatility of T7 polymerase buffers allows for their adaptation to different experimental needs. Their formulation can be tweaked depending on whether the focus is on quantitative studies, qualitative assessments, or exploratory research. This flexibility further emphasizes their significant role in advancing molecular biology research and its applications in real-world scenarios.
Components of T7 Polymerase Buffer
In molecular biology, the composition of a buffer can greatly influence the efficiency and fidelity of enzymatic reactions. The T7 polymerase buffer is no exception, as its components work synergistically to provide the ideal environment for nucleic acid synthesis. Understanding these components is crucial for researchers and practitioners looking to optimize their reactions. Each element contributes specific properties that enhance the overall performance of T7 polymerase, ensuring accurate transcription of RNA from DNA templates.
Salts and Ionic Strength
Salts play a vital role in the T7 polymerase buffer, influencing the ionic strength of the environment. The presence of salts helps stabilize the interactions between the enzyme, the nucleotides, and the DNA template. Commonly used salts include sodium chloride and potassium chloride. These ions can assist in minimizing non-specific interactions, increasing the overall specificity of the T7 polymerase. Furthermore, the right ionic strength can lead to improved enzyme activity. However, excessive salt concentrations can inhibit the reaction, underscoring the need for careful optimization.
Tris buffer: pH Stability
The Tris buffer is widely utilized in biological systems due to its excellent buffering capacity. In the context of the T7 polymerase buffer, Tris helps maintain a stable pH environment, which is crucial for enzymatic activity. The optimal pH for T7 polymerase is generally around 7.5 to 8.0. Fluctuations in pH can disrupt enzyme activity and messenger RNA stability. Therefore, using Tris ensures that subtle changes in experimental conditions do not compromise the results.
Magnesium Ion Concentration
Magnesium ions are crucial for the activity of T7 polymerase. They facilitate the catalytic mechanism of the enzyme by binding to the active site and stabilizing the formation of the RNA-DNA hybrid. The standard concentration of magnesium in T7 buffers typically ranges from 5 to 10 mM. It is important to note that too little magnesium can lead to low transcription yields, while too much can cause enzyme inactivation or non-specific transcription. Moderation in magnesium ion concentration is thus important for achieving optimal results during experiments.
Nucleotide Triphosphates
Nucleotide triphosphates (NTPs) are the building blocks required for RNA synthesis. The inclusion of adenosine triphosphate, cytidine triphosphate, guanosine triphosphate, and uridine triphosphate in the T7 polymerase buffer is essential for the transcription process. Each of these NTPs provides the necessary substrate for polymerization. The balance of these nucleotides affects the synthesis rate and RNA quality. Insufficient availability of any NTP can impede the transcription process, highlighting the need for precise formulation of the buffer.
Detergents and Stabilizers
Detergents and stabilizers help maintain enzyme activity over time. Commonly used detergents, like Triton X-100, can prevent protein aggregation and help maintain proper folding of the enzyme. Stabilizers such as glycerol may be included to prolong the shelf life of the buffer, ensuring that the T7 polymerase remains active during storage. The combination of these components contributes to a reliable and robust buffer system, minimizing the chances of degradation and loss of function.
The careful selection and precise combination of each component in the T7 polymerase buffer are paramount to achieving high yields and purity of RNA transcripts.
Each of these components plays a specific role in creating an optimal buffer environment for T7 polymerase. Understanding their individual contributions allows researchers to tailor the buffer for their specific applications, which can lead to more successful experimental outcomes.
Preparation of T7 Polymerase Buffer
The preparation of T7 polymerase buffer is a crucial step in ensuring the efficiency and effectiveness of nucleic acid synthesis. A well-prepared buffer provides the necessary ionic environment, pH stability, and essential components that facilitate the optimal function of T7 polymerase. Understanding the elements involved in this preparation can significantly improve experimental outcomes.
Protocol for Buffer Preparation
When preparing the T7 polymerase buffer, follow a stringent protocol to attain the ideal conditions. Here is a simplified detail of the steps:
- Gather Ingredients: Ensure you have all necessary components, including Tris-HCl, sodium chloride, magnesium acetate, and the appropriate dNTPs.
- Mix the Tris-HCl: Start with Tris-HCl, adjusting the pH to 7.5 using dilute hydrochloric acid. This buffering agent maintains the pH stability crucial for polymerase activity.
- Add Salts: Slowly mix sodium chloride into the solution to regulate ionic strength. A typical concentration is around 50 mM.
- Include Magnesium Ions: Add magnesium acetate to the mixture, adjusting to a final concentration of 10 mM. Magnesium ions are pivotal for T7 polymerase activity.
- Incorporate Nucleotide Triphosphates: Finally, add the required dNTPs into the buffer. They should be present at 500 µM for efficient polymerization.
- Adjust Volume: Complete the buffer preparation by adding distilled water to reach your desired final volume.
- Store Properly: Store the prepared buffer at -20°C for long-term usage.
This protocol should be followed closely to prevent variations that might impact polymerase efficiency.
Common Pitfalls in Preparation
During the preparation of T7 polymerase buffer, several common pitfalls can arise. It is essential to be mindful of these to avoid compromising experimental results:
- pH Mismanagement: Incorrect pH can adversely affect polymerase activity. Always verify the pH with a calibrated pH meter after adjustments.
- Ionic Strength Errors: Over or underestimating the sodium chloride concentration can alter the buffer’s performance. Adhere strictly to the specified amounts.
- Contamination Risk: Cross-contamination of reagents can result in unwanted experimental variables. Always practice good laboratory techniques.
- Inaccurate Measurements: Use precise instruments for weighing solids and measuring liquids. Inaccuracies can lead to an ineffective buffer composition.
- Temperature Fluctuations: Preparing buffers at too high or too low temperatures can impact enzyme activity. Ensure a controlled environment during preparation.
Being aware of these pitfalls allows for a more effective preparation process, leading to enhanced performance in subsequent experiments.
"A solid understanding of buffer preparation can greatly enhance the reliability of nucleic acid synthesis experiments."
Optimal Conditions for T7 Polymerase Activity
Understanding the optimal conditions for T7 polymerase activity is a crucial aspect in the field of molecular biology. Proper enzyme performance is essential for effective nucleic acid synthesis, which has implications in various labs and applications. The success of experiments often hinges on meeting specific parameters such as temperature, pH levels, and ionic strength. Each of these conditions directly influences the efficiency and fidelity of T7 polymerase, guiding researchers in achieving their desired results in RNA and DNA synthesis.
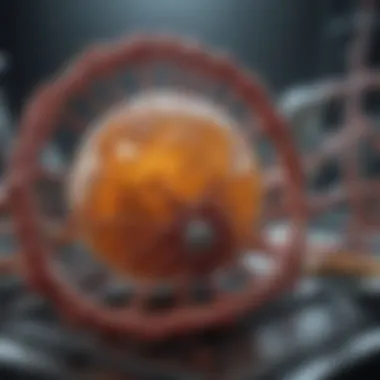
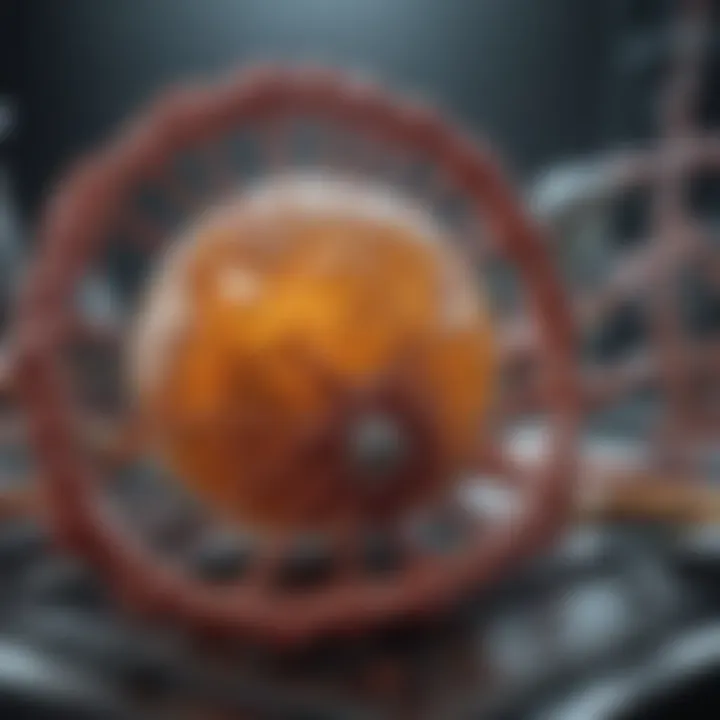
Temperature Considerations
Temperature plays a pivotal role in enzymatic reactions. T7 polymerase typically operates effectively at a range of temperatures, usually between 37°C and 42°C. At these temperatures, the enzyme exhibits optimal activity while minimizing potential denaturation risk.
It is important to note that deviations from this temperature range can lead to significant declines in enzyme activity.
- Too low: A temperature that is too low may reduce the kinetic energy of the molecules involved. This can lead to a slower reaction rate and an increase in the time needed for synthesis.
- Too high: Conversely, excessive heat can denature the enzyme, leading to irreversible loss of function.
"Maintaining a consistent temperature is fundamental for reproducible results in experiments utilizing T7 polymerase."
pH Levels and Their Impact
The pH of the buffer solution also significantly impacts the performance of T7 polymerase. The enzyme typically functions best at a neutral to slightly alkaline pH, around 7.5 to 8.5. Within this range, the active site of the enzyme remains optimally charged, facilitating substrate binding and activity.
If the pH strays from this optimal range:
- Acidic conditions may protonate the active site and interfere with substrate binding.
- Alkaline conditions can lead to deprotonation of essential amino acids in the active site, compromising enzyme functionality.
Researchers should routinely verify and adjust buffer pH to ensure enzymatic activity remains robust throughout the reactions.
Ionic Strength Optimization
Ionic strength is another critical factor that influences T7 polymerase activity. The presence of salts in the reaction buffer affects how the polymerase interacts with nucleic acid templates. An ideal ionic strength can help stabilize the enzyme and assist in proper substrate alignment.
Key points to consider include:
- Optimizing salt concentrations: Typically, potassium chloride is used, and concentrations optimizing T7 polymerase activity usually range between 50-100 mM.
- Maintaining enzyme-substrate interactions: Proper ionic strength can help prevent non-specific binding, which otherwise could lead to reduced efficiency and yield.
In summary, maintaining optimal temperature, pH levels, and ionic strength is crucial for T7 polymerase activity. Each condition is interconnected, and slight variations can have significant impacts on experimental outcomes. Researchers must be diligent and methodical in optimizing these parameters to ensure the fidelity and efficiency of their nucleic acid synthesis endeavors.
Applications of T7 Polymerase Buffer
T7 polymerase buffer plays a critical role in multiple facets of molecular biology. Its well-defined components create an optimal environment for enzyme activity. This leads to precise nucleic acid synthesis. Understanding the applications of this buffer illuminates its significance in genetic research and biotechnological advancements.
In Vitro Transcription
In vitro transcription is a powerful method for synthesizing RNA molecules. T7 polymerase is especially efficient in this process due to its high specificity and speed. The buffer allows for the proper ionic conditions and pH necessary for maximum enzyme activity.
Using T7 polymerase buffer improves transcript yield and quality. This is vital for applications such as constructing probes for diagnostics. Furthermore, purified RNA produced from in vitro transcription serves as templates in further experiments. Researchers can also use this RNA in functional studies. Proper buffer preparation is key in obtaining high-quality RNA samples.
Cloning and Vector Construction
Cloning involves the insertion of DNA fragments into vectors for propagation and study. T7 polymerase buffer is relevant here as it supports the synthesis of the required transcripts. These transcripts may include coding sequences for genes of interest or regulatory elements. In the context of vector construction, the buffer ensures that the polymerase works efficiently when synthesizing the RNA products. The generated RNA can then be reverse transcribed into cDNA, which is essential for cloning applications.
The accuracy of cloning is significantly influenced by the effectiveness of the buffer. A well-prepared buffer minimizes errors during the synthesis of the transcripts. Thus, it contributes directly to the reliability of cloning experiments.
Synthetic Biology Applications
Synthetic biology aims to design and build new biological parts and systems. T7 polymerase buffer is essential in this emerging field. Here, it aids in constructing synthetic circuits and pathways. This may include pathways for biosynthesis or genetic controls. The buffer provides a stable environment for enzyme activity, enhancing yields of desired products.
This usability extends to various platforms, such as expression systems. Scientists can incorporate genes of interest into relevant systems by utilizing T7 polymerase. The flexibility of applications in synthetic biology is vast.
Troubleshooting Common Issues
In the realm of molecular biology, where precision is critical, troubleshooting common issues with T7 polymerase buffers stands as a vital topic. Understanding the reasons behind failures in nucleic acid synthesis can save valuable time and resources. Addressing these problems requires an analytical approach, wherein one can identify and rectify the variables affecting the yield and quality of transcripts. This section discusses three prevalent issues: low yield of transcripts, non-specific binding problems, and degradation of nucleic acids. Identifying and resolving these issues not only enhances the efficiency of experiments but also ensures reproducibility and reliability of results.
Low Yield of Transcripts
A frequent concern when working with T7 polymerase is the low yield of transcripts. There are several underlying factors that contribute to this issue. One essential aspect is the quality and concentration of the template DNA. If the template does not contain the appropriate promoter sequence or is degraded, the polymerase may not efficiently initiate transcription. Ensuring the purity of DNA can mitigate this situation.
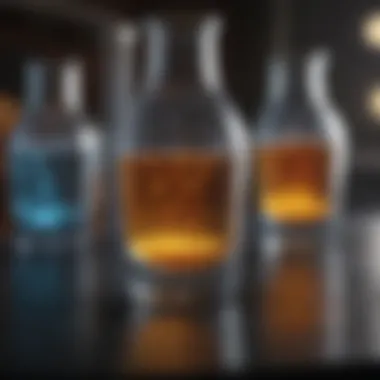
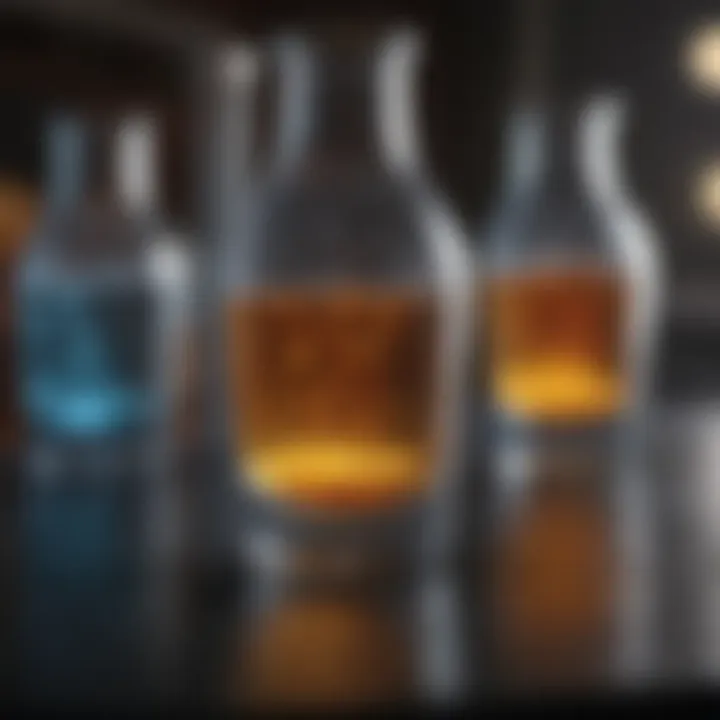
Additionally, inadequate concentrations of nucleotide triphosphates may lead to limited transcription activity. Monitoring the balance of components in the buffer is crucial. Lastly, factors such as temperature settings and incubation times also impact yield. For optimal performance, T7 polymerase often requires a specific temperature range, generally around 37°C. Implementing these strategies can help overcome low transcript yields.
Non-Specific Binding Problems
Non-specific binding during transcription can severely disrupt experimental outcomes. When T7 polymerase interacts with unintended regions of the template, it can produce undesired products. This issue often arises from improper buffer composition. Various salts, such as potassium chloride or sodium chloride, influence ionic strength and can reduce non-specific interactions by stabilizing the polymerase-template complex. Furthermore, the addition of detergents like Triton X-100 can assist in reducing these non-specific interactions.
In many cases, optimizing the concentration of magnesium ions in the buffer can also alleviate binding issues. It is essential to find a balance that enhances specific activity without encouraging non-specific associations. Fine-tuning these elements can lead to more accurate and defined transcripts, essential for downstream applications.
Degradation of Nucleic Acids
Degradation of nucleic acids is a significant issue that can compromise the integrity of experimental results. Various factors contribute to this concern, including inadequate storage conditions, contamination with nucleases, and the inherent instability of RNA. Ensuring that all reagents are nuclease-free is paramount. Utilizing high-quality water, such as DEPC-treated water, can prevent contamination.
Proper storage conditions also play a critical role in maintaining nucleic acid integrity. Wrapping samples in appropriate containers and storing them at temperatures that prevent degradation can provide substantial protection. Avoiding repeated freeze-thaw cycles is essential as well, as these processes can steadily contribute to the degradation of both DNA and RNA.
Regular monitoring of conditions and components can help mitigate these issues significantly and enable smoother workflows in molecular biology experiments.
Comparative Analysis with Alternative Buffers
In molecular biology, buffers play a crucial role in ensuring the functionality of enzymes like T7 polymerase. Understanding how T7 polymerase buffer compares with alternative buffers is key for optimizing experiments. The right buffer enhances enzyme activity, while inappropriate choices may hinder results. This comparison is important for researchers aiming to maximize the efficiency of nucleic acid synthesis.
Comparison with T7 Standard Buffer
The T7 standard buffer is widely used for various transcription reactions. Its composition typically includes Tris buffer for pH stability, magnesium ions for enzymatic function, and nucleotide triphosphates. However, researchers often need to explore alternatives based on specific needs. For example, some studies indicate that buffers with modified ionic strengths can enhance yield in terms of RNA production.
- Comparison factors:
- pH Stability: Non-standard buffers may offer better pH stability under specific conditions, leading to improved performance.
- Magnesium Concentration: Some researchers find altered magnesium levels increase transcript yield, highlighting the importance of buffer composition.
- Presence of Additives: Elements such as BSA or additional salts can be included in custom buffers to reduce non-specific binding, a common concern with the standard formulation.
The T7 standard buffer remains a go-to choice but customizing can yield greater benefits depending on the experimental context.
Advantages of Customized Buffers
Customized buffers provide several advantages over the T7 standard buffer. These adjustments tailor conditions to fit the precise requirements of a given experiment. Here are some aspects to consider:
- Increased Transcript Yields: As mentioned, optimized magnesium levels can lead to higher yield. The selective addition of salts can significantly affect transcription efficiency.
- Minimized Non-specific Binding: Custom formulations may include stabilizers or detergents, which can mitigate issues related to unwanted interactions between reagents. This reduces degradation and enhances the overall quality of results.
- Adaptation to Specific Reactions: Certain experiments require unique conditions due to variations in template design or desired output. Customized buffers enable scientists to meet these specific needs.
"Choosing the right buffer is not merely a technical detail; it can vastly alter the outcome of molecular operations."
The flexibility in designing buffers allows researchers to innovate and adapt to advance their work, particularly in fields requiring precision like synthetic biology or genetic engineering.
With ongoing exploration in buffer design, the understanding of T7 polymerase function can significantly improve, making this area ripe for future research.
Future Directions in Polymerase Research
The field of polymerase research is continually evolving, influenced by advancements in technology and increasing demands for precision in nucleic acid synthesis. This section addresses the importance of exploring future directions in polymerase research, particularly for T7 polymerase buffers. Understanding innovative approaches can significantly enhance the efficiency and specificity of biochemical applications. Moreover, recognizing trends helps researchers adapt their methods to remain at the forefront of molecular biology.
Emerging Technologies in Buffer Development
Emerging technologies are vital for refining the composition and effectiveness of T7 polymerase buffers. Recent developments in high-throughput sequencing and automation have revolutionized various aspects of buffer preparation. For instance, microfluidics allows for precise control over buffer components. This control can lead to better optimization of salt concentrations and pH levels, ultimately improving enzyme activity and yield.
Additionally, advancements in smart reagents have been introduced, which can adjust conditions in real-time. These adjustments could facilitate more robust reactions, minimizing variations in nucleic acid synthesis. Novel formulations that include specific additives can enhance enzyme stability in diverse experimental environments.
Interdisciplinary Applications
The applications of T7 polymerase buffers extend beyond traditional molecular biology laboratories. Interdisciplinary collaboration plays a significant role in expanding these applications. For example, insights from computer science and bioinformatics contribute to the modeling of enzymatic reactions. This modeling allows researchers to predict reactions' outcomes based on different buffer conditions, leading to informed experiments.
In environmental science, T7 polymerase buffers are critical for bioremediation efforts. By helping to clone genes responsible for degrading pollutants, T7 polymerase enhances the ability to study and implement clean-up strategies. The fusion of molecular biology with disciplines like material science is also gaining traction. This can lead to the development of new materials for drug delivery systems that utilize nucleic acid-based therapies.
Potential Breakthroughs
Looking ahead, the potential breakthroughs in T7 polymerase buffer research are promising. One area of focus is the optimization of buffers for single-molecule sequencing applications. As this technology becomes more prevalent, improvements in buffer formulations will be crucial for reliability and accuracy.
The advent of CRISPR and gene-editing techniques relies heavily on efficient and effective buffers. Future breakthroughs may forge new guidelines for optimizing buffers that can significantly impact these technologies. These enhancements could streamline protocols, reduce costs, and improve outcomes in various nucleic acid-based applications.
Furthermore, investigating novel buffer ingredients could unlock new capabilities in gene expression systems. The integration of synthetic biology tools provides another avenue for innovative buffer solutions that meet specific experimental needs.
In summary, the future of polymerase research is intertwined with advancements in buffer technology and interdisciplinary approaches, fostering exciting opportunities in various fields.