Exploring Two-Photon Polymerization in 3D Printing
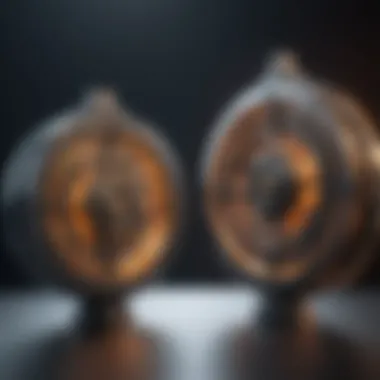
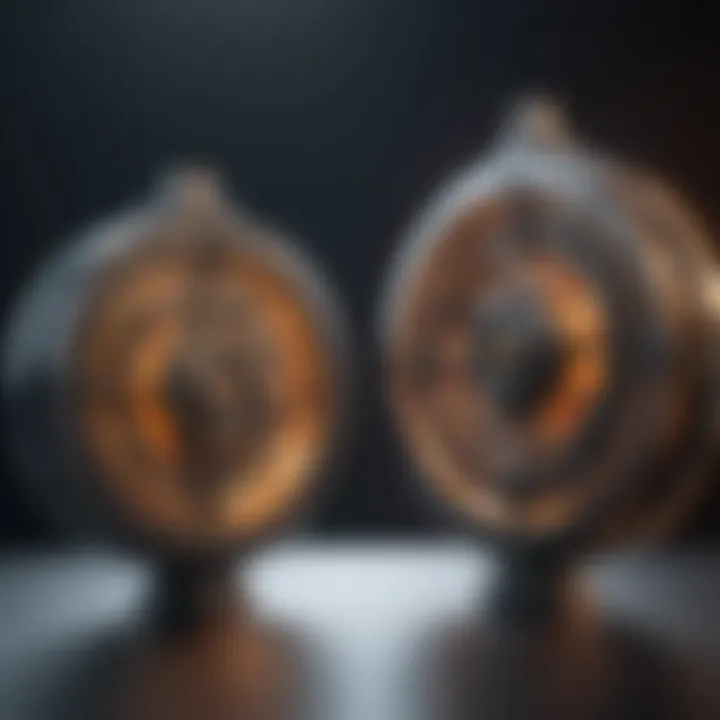
Intro
Two-photon polymerization (TPP) represents a significant advancement in the field of 3D printing. This technology utilizes the principles of quantum mechanics to enable unprecedented precision in creating intricate microstructures. As industries increasingly pursue advanced manufacturing techniques, understanding TPP's capabilities becomes essential. The unique aspects of TPP not only enhance the fidelity of 3D-printed objects but also expand the potential applications across sectors such as healthcare, electronics, and materials science.
The process exploits the nonlinear absorption of two-photon excitation, allowing for the polymerization of photosensitive materials with high spatial resolution. This leads to the creation of complex geometries that are difficult to achieve with conventional 3D printing methods. It is crucial to explore the underlying principles of TPP, the advantages it provides, and the current challenges within this evolving field.
Research Methodology
Description of Research Design and Approach
In order to explore TPP, a systematic approach was utilized that included both theoretical and experimental components. The research design focused on a multidisciplinary framework integrating physics, materials science, and engineering. By collating recent studies and data from various sources, including peer-reviewed journals and industry reports, insights into TPP's effectiveness were garnered.
Key aspects of the research involved:
- Analyzing existing literature on TPP to establish a comprehensive theoretical foundation.
- Conducting experiments with different photopolymer materials to evaluate performance in terms of speed, resolution, and structural integrity.
- Comparing TPP techniques with traditional additive manufacturing methods to highlight distinct advantages.
Materials and Methods Used in the Study
The materials utilized in this research included a variety of photopolymers, notably those sensitive to two-photon excitation. These materials were characterized to assess their physical and chemical properties. Experimental setups typically featured a laser system capable of generating pulses at specific wavelengths suitable for initiating TPP.
Methods involved:
- Laser Pulsing: Employing a femtosecond laser to trigger the polymerization process, enabling precision down to the micrometer scale.
- Sample Preparation: Fabricating samples through iterative layers to construct the desired microstructures.
- Imaging Techniques: Using scanning electron microscopy (SEM) and atomic force microscopy (AFM) to inspect the structural details of the printed components after fabrication.
Understanding these methodologies provides a clear context for analyzing the implications of TPP in modern manufacturing.
Discussion and Interpretation
Interpretation of Results in the Context of Existing Literature
The findings from current research demonstrate that TPP not only yields higher resolution than traditional methods, but it also enables the creation of structures with complex architectures, such as lattices and scaffolds. Such capabilities are not extensively reported in existing literature, substantiating TPP's uniqueness and importance.
TPP stands out by enabling the creation of objects that possess complex internal structures and geometries not feasible with conventional 3D printing.
Implications for Future Research or Practical Applications
As industries continue to evolve, the implications of TPP's capabilities are vast. Future research may potentially lead to the development of novel applications in bioprinting for tissue engineering or in creating customizable electronic components. The pursuit of efficiency and cost-effectiveness remains a priority, presenting opportunities for further innovation within TPP technology. By addressing current challenges—such as scaling production and improving material properties—TPP could drastically redefine norms within manufacturing sectors.
Foreword to Two-Photon Polymerization
Two-photon polymerization (TPP) emerges as a significant milestone in the landscape of 3D printing technology. It fundamentally transforms how materials are structured and enables the creation of intricate 3D objects at the micro and nanoscale. Understanding TPP is essential, as it harnesses advanced photonic techniques to achieve results unattainable by traditional 3D printing methods.
The importance of TPP lies in its unique ability to facilitate high-resolution feature generation. Unlike conventional methods, TPP allows for the precise construction of complex geometries and intricate designs, which can have substantial applications across multiple fields including biology, optics, and materials science. This precision is crucial, particularly when creating components that demand tight tolerances and specific performance characteristics.
Additionally, TPP significantly reduces material waste compared to its counterparts. The focused light only initiates polymerization at the desired site, minimizing excess material. This efficiency is essential, especially in industries where material costs can quickly escalate.
Moreover, TPP is intrinsic to advancements in biomedical applications. With its capacity for producing intricate biomaterials, it plays a vital role in tissue engineering and the development of scaffolds that closely replicate natural tissue structure. This allows researchers to explore new therapeutic avenues in regenerative medicine.
As we delve into the complexities of two-photon polymerization, it is necessary to understand the foundational concepts that support this innovative technology.
Definition and Basic Principles
Two-photon polymerization is a process that employs a dual-photon absorption mechanism to initiate polymerization. When two photons are absorbed simultaneously, they excite a photo-sensitive resin, triggering chemical reactions that lead to solidification. This phenomenon allows for the precise location of polymerization at the focus of a laser beam, enabling the fabrication of intricate three-dimensional structures.
The basic principle of TPP revolves around the use of a laser beam, typically in the femtosecond range. Focusing the laser light into a small volume of resin causes localized polymerization. The resulting polymerized region can exhibit a variety of properties, depending on the chosen resins and post-processing methods.
Due to its ability to confine light to a minuscule spot, TPP stands out for its unparalleled resolution. This allows for feature sizes down to the nanometer scale, making it particularly advantageous for applications that require extreme precision.
Historical Development of TPP Technology
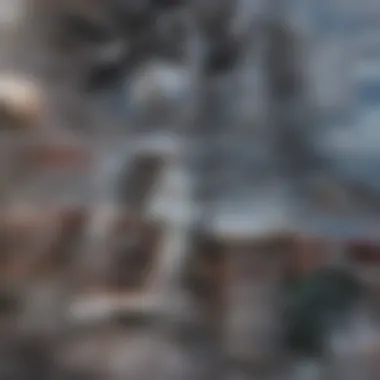
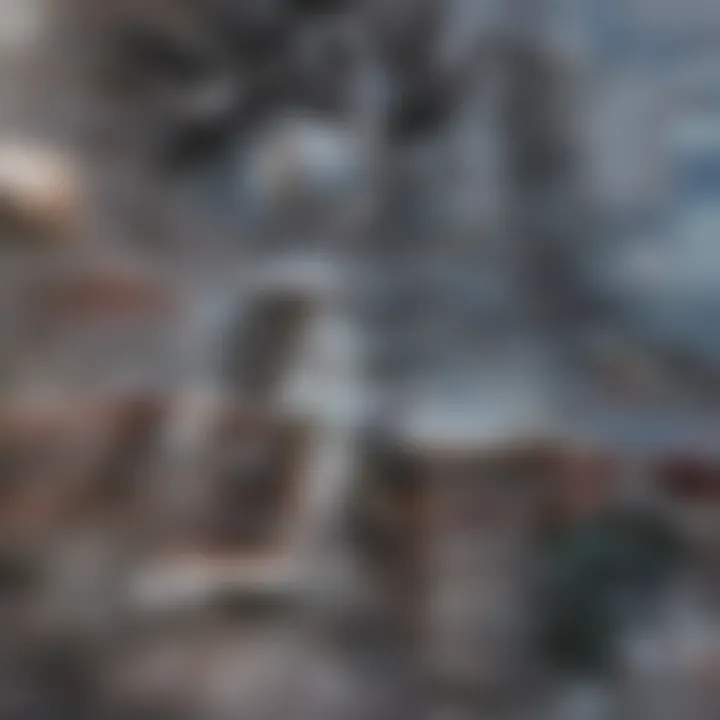
The roots of two-photon polymerization can be traced back to the early 1990s, with significant breakthroughs made in imaging and laser technologies. Pioneering work by scientists such as W.E. Moerner and many others laid the groundwork for understanding two-photon absorption processes. With advancements in laser technology, it became feasible to utilize TPP for applications beyond microscopy.
In 1999, the first demonstration of using TPP for 3D microfabrication was published, showcasing the promise of this technology for creating complex three-dimensional structures. This marked the beginning of a new era in microfabrication. Subsequent research led to the refinement of TPP techniques and materials, paving the way for commercial applications.
Throughout the 2000s and beyond, there has been a steady increase in interest and investment in TPP technology. Research expanded into diverse fields, focusing on biomaterials, optical devices, and advanced manufacturing processes. Today, TPP is an established method in various industrial applications, demonstrating its significance and potential within the realm of 3D printing.
"Two-photon polymerization represents a crucial intersection of optics and material science, demonstrating how fundamental physics can unlock novel manufacturing techniques."
In summary, two-photon polymerization is not merely a technical advancement; it is a disruptive force that reshapes our approach to design and production in 3D printing. Understanding its fundamentals and historical context is essential for appreciating its contemporary applications and implications.
Mechanics of Two-Photon Polymerization
The mechanics of two-photon polymerization (TPP) are central to understanding its effectiveness and advantages in the field of 3D printing. This process is distinct in its utilization of highly localized polymerization, allowing for the creation of structures with unmatched precision and resolution. The intricate balance of photon absorption and emission, the generation of free radicals, and the kinetics of polymerization all play significant roles in the operational efficiency of TPP. By delving into these specific elements, we can appreciate how TPP stands out among traditional 3D printing technologies and its broader applicability in various fields.
Photon Absorption and Emission Process
In TPP, the process begins with the absorption of photons by a photosensitive resin. These resins are typically designed to only polymerize when exposed to light of specific wavelengths, commonly in the infrared spectrum. The unique aspect of two-photon absorption is that it requires the simultaneous absorption of two photons to reach an excited state.
This characteristic results in a very localized region of polymerization, confined to the focal point of the laser. The energy from these photons induces an emission process, crucial for the subsequent creation of a polymer network. Understanding this dual process is vital for optimizing TPP as it enables the fabrication of micro and nanostructures with profound detail while minimizing unwanted reactions outside the targeted area.
Production of Free Radicals
The production of free radicals is a crucial step in TPP. Upon successful absorption of two photons, the photosensitive material undergoes a transformation that generates free radicals. These highly reactive particles serve as initiators for the polymerization reaction. The radicals propagate, joining monomer units and creating a polymer chain.
One of the significant advantages here is the efficiency of this process. Compared to traditional one-photon absorption methods, the two-photon mechanism minimizes the required energy input, thus reducing the risk of unwanted side reactions. This allows for greater control over the polymerization process and enhances the quality of the final structures.
Polymerization Kinetics in TPP
Polymerization kinetics in TPP refer to the monitoring and understanding of how fast the polymer chains form and how this affects the overall structure developed. The kinetics can be influenced by several factors, such as light intensity, exposure time, and the molecular characteristics of the resin used.
The non-linear nature of light absorption in TPP introduces complexities not seen in other forms of polymerization. With TPP, the polymerization rate can be controlled precisely, allowing for gradual or rapid changes in structure based on the desired outcome. This fine-tuned control is paramount, particularly when developing intricate geometries that traditional methods may struggle to achieve.
"Two-photon polymerization not only expands the limits of traditional 3D printing but also opens new avenues for applications in biotechnology, microfluidics, and beyond."
In summary, the mechanics of TPP—rooted in photon absorption, free radical production, and polymerization kinetics—enable the creation of high-resolution and complex structures. These features highlight the transformative potential of TPP in the 3D printing landscape.
Advantages of Two-Photon Polymerization
Two-photon polymerization (TPP) stands out in the crowded field of 3D printing technologies due to its remarkable advantages. This innovative technique allows for the creation of highly intricate structures with superior precision, providing various applications across different sectors. Understanding these advantages is crucial for anyone analyzing modern manufacturing techniques. The following highlights key benefits of TPP:
High Resolution and Precision
TPP is known for its unparalleled high resolution, which is a significant advantage over many traditional 3D printing methods. The technique utilizes the nonlinear absorption of photons, enabling the polymerization process to occur at a very small scale, often at the micron or sub-micron level.
This high level of detail is invaluable in fields requiring intricate designs, such as microelectronics and biomedical applications. For instance, research in tissue engineering relies on the ability to create precise scaffolds that can mimic the natural tissue architecture. Structures created with TPP can incorporate features such as channels for nutrient flow, which enhances cellular growth and functionality. The precision offered by TPP not only improves the designs but also enhances the performance of the final products.
Reduction of Material Waste
One of the pressing concerns in modern manufacturing is material waste. TPP contributes significantly to reducing this waste. The focused laser beam used in this technique delivers energy only to selected volumes of the resin, allowing for selective curing. This is in contrast to conventional methods, where excess material often becomes scrap.
By minimizing the need for support structures and allowing for more efficient use of the material, TPP helps in reducing costs and enhancing sustainability. This aspect is particularly appealing to companies looking to improve their eco-friendliness. An efficient use of materials aligns with the growing demand for sustainable manufacturing practices.
Capability for Complex Structures
TPP excels in creating not just simple shapes, but complex geometries that are often challenging for traditional fabrication methods. The laser's ability to move in three dimensions enables the formation of sophisticated internal structures and architectures. This is critical in applications like photonic devices and advanced biomaterials.
For example, the design of optical devices that require specific light manipulation often necessitates structures with intricate patterns. Additionally, TPP is advantageous in creating structures with varying mechanical properties within a single component. This capability allows for a tailored approach in engineering applications, offering significant progress over past limitations in 3D printing technologies.
The advantages of two-photon polymerization extend beyond mere technical specifications. They encapsulate a transformative potential across industries, pushing the boundaries of what is manufacturing possible.
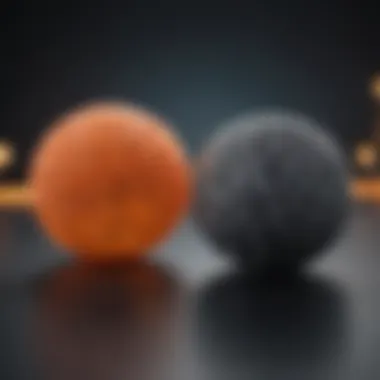
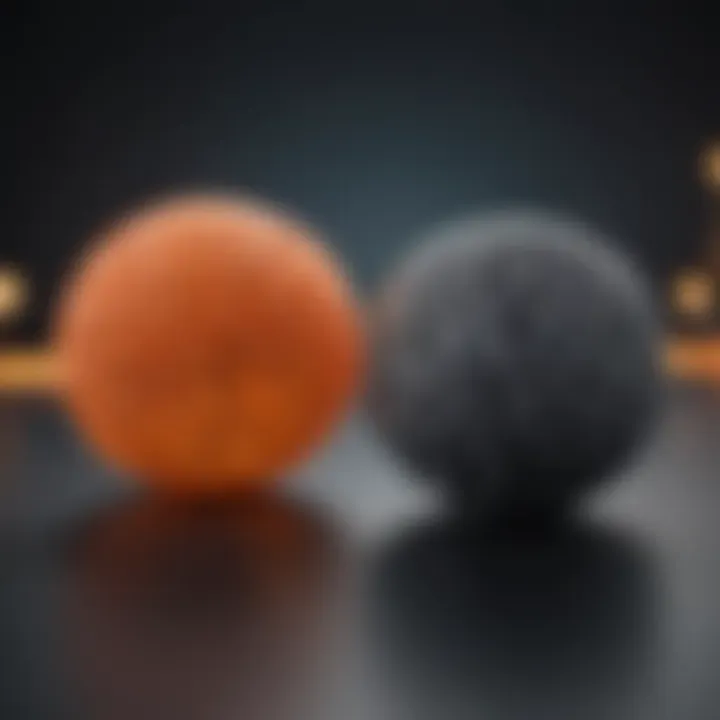
In summary, two-photon polymerization stands as a powerful method in the 3D printing landscape, characterized by high resolution, reduced waste, and the ability to create complex structures. Each of these advantages plays a critical role in its growing importance in technology and industry today.
Applications of Two-Photon Polymerization
The applications of two-photon polymerization (TPP) represent a significant area of interest in the field of 3D printing. The unique properties of TPP make it suitable for various fields, contributing to advancements that were previously unattainable by traditional manufacturing methods. This section elucidates key applications that harness the capabilities of TPP, highlighting its relevance and the benefits it brings across diverse industries.
Use in Microfabrication
Microfabrication is one of the paramount applications of TPP. This technique allows for the creation of intricate microstructures with high precision. Thanks to the nonlinear phenomenon of two-photon absorption, TPP can achieve resolutions down to a few hundred nanometers, making it ideal for manufacturing microdevices.
- Key Advantages: TPP reduces the challenges commonly associated with traditional microfabrication methods, such as photolithography. In TPP, the polymerization occurs only at the focal point of the laser, minimizing the exposure of surrounding areas. This precision results in less material usage and the ability to create complex geometries that are often required in micro-electromechanical systems (MEMS) and other advanced applications.
- Applications in Industry: Industries like electronics, optics, and biomedical sectors utilize microfabrication techniques for their products. For example, TPP can create micro-lenses and micromechanical components that are essential in advanced optical systems. Moreover, it plays a role in developing sensors, actuators, and other critical applications which benefit from its high resolution and design flexibility.
Biomaterials and Tissue Engineering
The integration of two-photon polymerization in biomaterials and tissue engineering marks a transformative approach to creating biological structures. TPP allows for the fabrication of scaffolds that mimic the extracellular matrix in living organisms.
- Customizable Porosity and Morphology: Using TPP, researchers can design scaffolds with various porosity levels and architectural complexity. This ability to customize the microenvironment significantly enhances cell attachment and proliferation.
- Applications in Regenerative Medicine: These scaffolds can be used in bone and cartilage regeneration, among other applications. By tailoring the polymer properties, it is also possible to create drug delivery systems that release therapeutics in a controlled manner, enhancing treatment outcomes.
Optical Devices and Metamaterials
Optical devices benefit markedly from the unique properties of two-photon polymerization. It is employed to craft complex 3D photonic structures that operate on sub-wavelength scales, which enables the manipulation of light in novel ways.
- Metamaterials Creation: TPP is advantageous for constructing metamaterials that possess unusual optical characteristics, including negative refractive indexes. These materials can lead to advancements in imaging, sensing, and information processing technologies.
- Applications in Telecommunications: The precision offered by TPP allows for the development of optical resonators and waveguides, which are critical components in telecommunication systems. The fabrication of these devices with high accuracy enhances signal transmission efficiency and opens pathways to improved communication technologies.
TPP has revolutionized the production of structures at the micro and nanoscale, greatly influencing multiple industry domains.
Challenges and Limitations of TPP
Two-photon polymerization, while promising and innovative, is not without its challenges and limitations. These hurdles must be carefully considered as they determine the feasibility and scope of TPP technology in practical applications. Understanding these issues is crucial for researchers and professionals seeking to enhance the efficiency and effectiveness of TPP in various industries.
Material Constraints
Material selection plays a vital role in the success and efficiency of two-photon polymerization. The materials used in TPP must exhibit specific properties to enable effective polymerization. Commonly, photopolymer resins are utilized, yet not all of them are suitable for the two-photon approach.
For instance, the sensitivity of the material to the wavelength of light is paramount. Many resins require precise tuning to ensure proper absorption at the two-photon energy levels. Furthermore, these materials need to maintain structural integrity after polymerization, a requirement not met by all types of resins available. Some materials may also have limitations in terms of thermal stability or mechanical strength, which affect their applicability in real-world situations.
Additionally, the development of new materials can be slow and resource-intensive. Researchers often face the challenge of balancing cost, performance, and availability, which can hinder progress in adopting TPP technology. Achieving patient uniformity and consistency across batches further complicates material choice, making it necessary to invest ample resources for effective testing and refinement.
Technological Barriers
Technological limitations are another significant challenge facing TPP. The process mainly requires sophisticated laser systems for the excitation of two photons. These systems can be costly and may not be widely accessible, particularly in smaller or resource-limited settings. The need for specialized equipment can create a barrier for widespread adoption of TPP in various sectors.
Moreover, the current imaging techniques used to monitor and control the polymerization process are often insufficient for real-time feedback. Effective monitoring is crucial, especially for applications demanding high precision and accuracy. As of now, improvements in imaging technologies are needed to better reflect the dynamic nature of the process, enhancing control and stability.
Efficiency in the printing process itself is another aspect that requires attention. Speed remains an issue, as TPP can be slower compared to other 3D printing techniques such as stereolithography and fused deposition modeling. While TPP offers precision, the trade-off for speed needs to be addressed if it is to compete on a larger scale.
In summary, the challenges and limitations in two-photon polymerization encompass both material and technological constraints. The successful advancement of TPP technology hinges on overcoming these obstacles.
Comparative Analysis with Other 3D Printing Technologies
The exploration of two-photon polymerization (TPP) inherently involves a comparative analysis with other dominant 3D printing technologies. Understanding how TPP stacks up against alternatives like stereolithography and fused deposition modeling is crucial for identifying its unique benefits and limitations. This analysis not only provides intellectual clarity on TPP's position in the broader landscape of additive manufacturing but also highlights its competitive advantages in terms of precision, material use, and design complexity. As the industry evolves, the capability of TPP to address specific shortcomings common to other methods plays a pivotal role in its adoption and further innovation.
Stereolithography
Stereolithography, or SLA, is one of the most established forms of 3D printing. It employs a laser to cure liquid resin into hardened plastic, layer by layer. SLA is renowned for its high accuracy and smooth surface finish, making it a favorite in industries such as prototyping and direct manufacturing.
Key Advantages of SLA:
- Precision: SLA offers fine details due to its capacity to create layers as thin as 25 microns.
- Surface Quality: Parts produced through SLA often exhibit minimal post-processing needs due to their smooth finish.

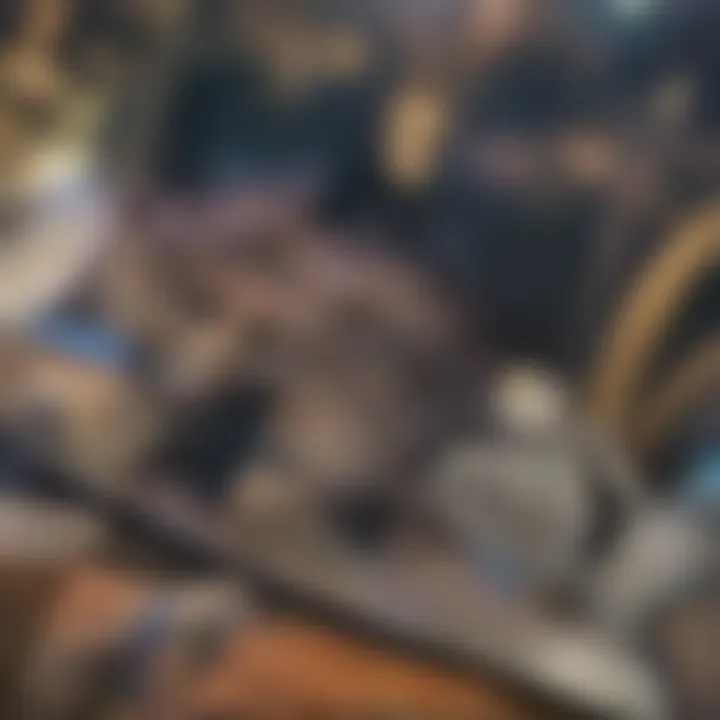
However, there are notable limitations to SLA:
- Speed: SLA can be slower than TPP, especially for larger prints, as it depends on layer-by-layer curing.
- Material Constraints: The types of materials suitable for SLA print are generally limited, leading to significant challenges in applications requiring specific material properties.
In direct comparison, TPP provides exceptional resolution owing to its two-photon absorption process. This mechanism allows for clearer delineation of microstructures, which can lead to more intricate designs. In applications where detail is non-negotiable, TPP outperforms SLA effectively.
Fused Deposition Modeling
Fused deposition modeling, or FDM, is another commonly used method in the realm of 3D printing. FDM works by extruding thermoplastic filament through a heated nozzle, gradually layering it until the desired object emerges. It is widely recognized for its ease of use and the broad array of thermoplastics available for printing.
Key Advantages of FDM:
- Material Variety: A wide range of thermoplastics, such as PLA and ABS, can be utilized, offering many choices for different applications.
- Cost-Effectiveness: FDM printers are generally more affordable, making this technology accessible for hobbyists and educational institutions.
However, FDM suffers from several key disadvantages:
- Resolution Limitations: Compared to TPP and SLA, FDM does not achieve the same level of detail, limiting its use in high-precision applications.
- Strength and Durability: The mechanical properties of FDM parts can be weaker due to layer adhesion issues.
When juxtaposed with TPP, FDM's resolution shortcomings are clear. TPP's ability to print complex geometries with unprecedented precision positions it as a more suitable choice for applications requiring intricate designs, such as biomedical scaffolds or advanced photonic devices.
“Two-photon polymerization stands apart from traditional methods, tailored for the detailed complexity of today’s advanced applications.”
In summary, the comparative analysis of TPP with techniques like stereolithography and fused deposition modeling reveals its strengths in precision and adaptability. As the technological landscape of 3D printing evolves, understanding these contrasts will aid in recognizing TPP's potential impact on future developments in manufacturing.
Future Directions in Two-Photon Polymerization Research
The research in two-photon polymerization (TPP) is evolving continuously. The future of this field holds significant potential to revolutionize various industries. As we explore future directions, it is essential to recognize the specific developments that may lead to enhanced efficiency and broader application of TPP technology. Understanding these elements not only informs current practices but also sets the stage for innovative breakthroughs in the coming years.
Innovative Materials Development
One of the pivotal areas of future TPP research is the development of innovative materials. Current TPP applications often use photoinitiators that are limited in performance, leading to constraints on the structures that can be created. Researchers are focusing on synthesizing novel photopolymerizable materials to enhance both the properties and capabilities of TPP.
- Biocompatibility: The creation of biocompatible materials for applications in tissue engineering is particularly promising. This will allow for better integration with biological systems, which is crucial for regenerative medicine.
- Smart Materials: Another interesting pathway involves the design of so-called smart materials that can respond to external stimuli like light or temperature. This adaptability will open doors for the creation of dynamic structures that can change their properties in real time.
- Hybrid Materials: Developing hybrid materials that combine different properties from traditional and innovative materials can lead to the fabrication of more complex structures. This versatility can greatly expand the possibilities in microfabrication applications.
Enhancements in Imaging Techniques
Imaging techniques play a vital role in two-photon polymerization. As precision is key in TPP, enhancing imaging techniques can drastically improve the outcomes of 3D printing processes.
- Real-time Monitoring: Implementing advanced imaging technologies, such as real-time monitoring systems, can help in assessing polymerization processes as they unfold. This capability can lead to improved accuracy and precision during the 3D printing process.
- Multi-Photon Excitation: Future research may explore multi-photon excitation methods to enhance the resolution and processing speed. This could be beneficial for applications requiring intricate designs, such as micro-optical devices.
- Image Analysis Algorithms: Developing new algorithms for image analysis can significantly improve the accuracy of defect detection in printed structures. Having precise feedback will guide adjustments in the printing parameters, resulting in higher quality outputs.
The integration of innovative materials and enhanced imaging techniques will drive the advancement of TPP, transforming its application landscape in 3D printing.
The interplay of these future directions holds great potential for TPP, fostering a more comprehensive understanding of its capabilities and expanding its application across different fields. As researchers continue to push the boundaries, the implications for technology and industry are profound.
Epilogue
The conclusion serves as the pivotal end point of this article, synthesizing the key themes and insights shared throughout the discussion on two-photon polymerization (TPP) in 3D printing. Emphasizing its significance is essential as TPP represents a transformative approach to additive manufacturing. The ability to create high-resolution structures with intricate details and complex geometries sets TPP apart. Its applications span various fields, including microfabrication, biomaterials, and optical devices, showcasing its versatility and potential impact on multiple industries.
Summary of Key Points
In this comprehensive examination, several vital aspects have been covered including:
- Definition and Basic Principles: Understanding TPP as a unique polymerization process that uses two-photon absorption.
- Advantages: Highlighting the high resolution, reduced material waste, and ability to create complex structures.
- Applications: Discussing real-world applications in microfabrication and tissue engineering which demonstrate TPP's utility.
- Challenges: Addressing material limitations and technological barriers that continue to stymie broader adoption.
These key points underscore how TPP does not merely enhance 3D printing but also opens new realms of possibilities for technology and science.
Implications for the Future of 3D Printing
Looking ahead, the implications of two-photon polymerization are substantial. As research progresses, innovations in materials could lead to even more robust applications, possibly integrating TPP with other additive manufacturing techniques. Enhanced imaging techniques promise to refine the fabrication process further.
Moreover, as industries recognize the value of precision and customization, TPP could become increasingly preferred. This will likely drive further investment and research, resulting in a cycle of continual improvement and wider adoption. Investing time in understanding TPP can lead to advancements in various fields from healthcare to industrial applications.
Overall, the future of TPP in 3D printing is not only promising but pivotal, shaping how we think about manufacturing in the modern age.
"The synthesis of high precision and adaptability makes TPP a cornerstone technology for the next generation of 3D printing materials and applications."
As technology evolves, the focus on refining and expanding the capabilities of two-photon polymerization will likely yield groundbreaking advancements that may redefine industry standards.