Exploring the Diverse Types of Vaccines Available Today
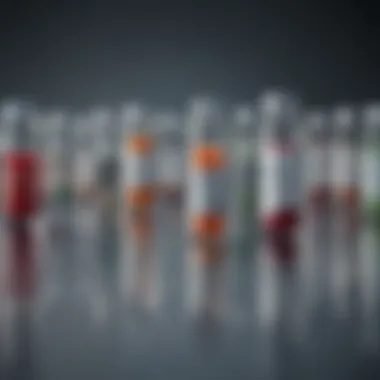
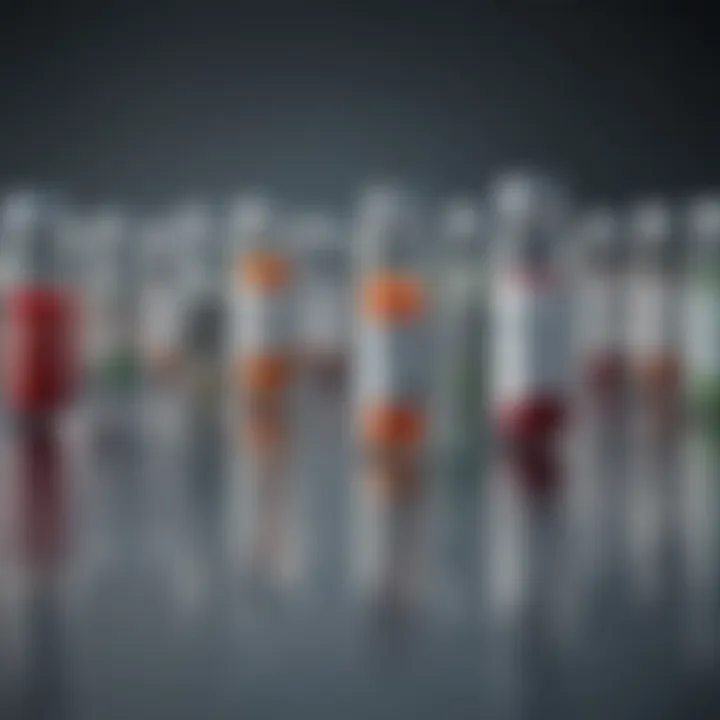
Intro
Vaccines are a cornerstone of public health, profoundly impacting the management of infectious diseases. This article will reveal various types of vaccines currently available. The goal is to provide clarity on their mechanisms, advantages, limitations, and the specific diseases they protect against. Understanding these elements is crucial for students, researchers, and healthcare professionals engaged in vaccine development and policy-making.
A vaccine essentially trains the immune system to identify and combat pathogens. Yet, not all vaccines operate in the same manner, nor do they target identical diseases. Clarifying this scope is significant to appreciate their diverse roles in medicine.
Research Methodology
The research design for this article follows a comprehensive literature review approach. This includes examining peer-reviewed journals, public health records, and current vaccine guidelines. The intention is to distill complex information into an easily digestible format suitable for an educated audience.
Description of Research Design and Approach
The approach primarily involves analyzing existing research on vaccines. This encompasses both classical vaccines, like those for polio and measles, and modern innovations such as mRNA vaccines. Special attention is given to how different vaccines interact with the immune system.
Materials and Methods Used in the Study
Materials include scientific articles sourced from databases like PubMed, as well as vaccine studies from the World Health Organization and Centers for Disease Control and Prevention. Methods involve synthesizing data on efficacy, formulation differences, and historical context to produce a well-rounded overview of vaccine types.
Discussion and Interpretation
Existing literature on vaccines demonstrates the shifting landscape of immunology and vaccine development. Each vaccine type contributes uniquely to health interventions.
Interpretation of Results in the Context of Existing Literature
For example, live attenuated vaccines, such as the vaccine for measles, rely on a weakened form of the virus. Alternatively, inactivated vaccines like the polio vaccine use killed pathogens. Each methodology possesses distinct advantages and risks.
"Understanding the diversity and mechanisms of vaccines helps prepare for future health crises and fosters proactive prevention strategies."
Implications for Future Research or Practical Applications
The analysis points to the necessity of ongoing research. This is especially true for developing novel vaccines against emerging diseases. Furthermore, there is a demand for enhancing public understanding of vaccine science to combat misinformation.
Prelude to Vaccines
Historical Context
The concept of vaccination dates back several centuries. Edward Jenner's pioneering work in 1796 laid the foundation for modern immunology. He introduced the smallpox vaccine, using material from cowpox lesions. This marked the beginning of the practice to protect against diseases through vaccination. Over the years, this practice evolved significantly. With advancements in science and technology, various methods were developed to create vaccines. For example, Louis Pasteur introduced the principles of attenuation and killed vaccines in the late 19th century, further expanding the repertoire of vaccination techniques. Today, the historical context of vaccines is vital for understanding their impact on global health and the ongoing fight against infectious diseases.
Importance of Vaccination
Vaccination is vital for individual and community health. Vaccines not only protect the vaccinated individuals but also contribute to herd immunity, reducing the overall spread of diseases. The benefits of vaccination go beyond mere disease prevention. They drastically lower healthcare costs, reduce the burden on medical systems, and enhance overall quality of life. Moreover, vaccination plays an essential role in controlling outbreaks of infectious diseases.
The effectiveness of vaccines in eradicating diseases like smallpox and significantly reducing cases of diseases such as polio and measles showcases their importance in public health. Vaccination programs are crucial for maintaining a healthy population and preventing disease resurgence. For students, researchers, healthcare professionals, and the general public, understanding the significance of vaccines is essential in fostering informed discussions about health practices and policies.
"Vaccines are not just individual protections. They are community safeguards."
Basic Classification of Vaccines
Understanding the classification of vaccines is crucial for grasping their role in public health. Different types of vaccines operate through various mechanisms, leading to distinct immune responses. This section delves into five primary categories of vaccines: live attenuated, inactivated or killed, subunit, recombinant, conjugate, messenger RNA, and viral vector vaccines. Each type has unique benefits and limitations that directly impact their application in disease prevention. Knowledge of these classifications aids healthcare professionals in selecting appropriate vaccines for specific populations. It also informs policymakers about vaccine strategies vital for controlling outbreaks and improving immunization rates.
Live Attenuated Vaccines
Live attenuated vaccines use a weakened form of the virus or bacterium that causes the disease. This weakening is often achieved through processes like genetic engineering or repeated culture in specific conditions. As a result, these vaccines can provoke a robust immune response similar to natural infection without causing the actual disease.
Common examples include:
- Measles, Mumps, and Rubella (MMR) vaccine
- Oral Polio Vaccine (OPV)
- Yellow Fever vaccine
Advantages:
- Strong and long-lasting immunity
- Generally require fewer doses
Disadvantages:
- Not suitable for immunocompromised individuals
- Some risk of reversion to virulent form
Inactivated or Killed Vaccines
Inactivated or killed vaccines contain viruses or bacteria that have been destroyed by heat, chemicals, or radiation. This process ensures that the pathogens cannot replicate or cause disease. The immune response from these vaccines may not be as strong as that from live vaccines, often requiring booster shots for sustained immunity.
Common examples of inactivated vaccines include:
- Hepatitis A vaccine
- Rabies vaccine
- Influenza vaccine
Benefits:
- Safe for immunocompromised individuals
- Does not pose a risk of disease
Risks:
- Weaker immune response requiring multiple doses
- Possible mild side effects like soreness at the injection site
Subunit, Recombinant, and Conjugate Vaccines
These vaccines contain specific pieces of the pathogen, such as proteins or sugars, rather than the whole virus or bacterium. Subunit vaccines include purified proteins, while recombinant vaccines are produced using genetic engineering techniques. Conjugate vaccines link polysaccharides from the pathogen to a protein carrier, enhancing the immune response.
Some well-known examples are:
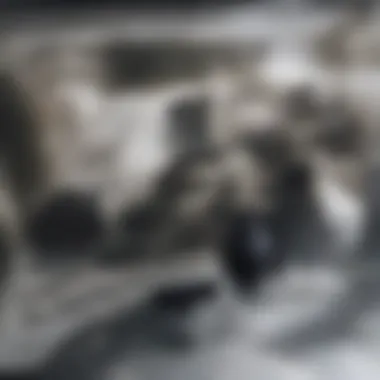
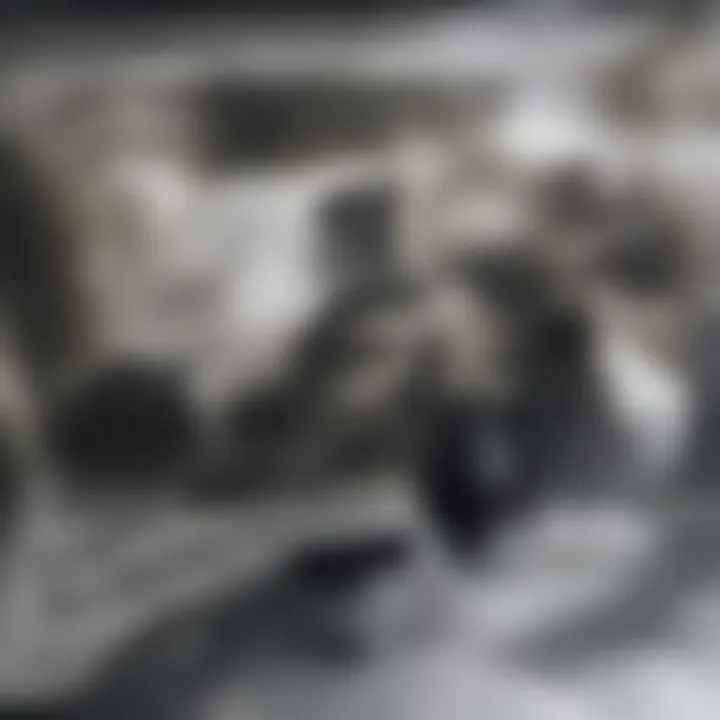
- Human Papillomavirus (HPV) vaccine
- Haemophilus influenzae type b (Hib) vaccine
- Pneumococcal conjugate vaccine
Mechanism of action:
- Targeted immune response against specific antigens
- Limited risk of adverse reactions compared to live vaccines
Clinical applications:
- Effective against diseases where full pathogen vaccination poses risks
- Used in childhood vaccinations where safety is paramount
Messenger RNA Vaccines
Messenger RNA (mRNA) vaccines deliver genetic material that instructs cells to produce a harmless piece of the pathogen, triggering an immune response. This novel approach has gained attention recently, particularly during the COVID-19 pandemic.
Examples include:
- Pfizer-BioNTech COVID-19 vaccine
- Moderna COVID-19 vaccine
What sets them apart:
- Rapid deployment and adaptability to emerging pathogens
- Strong immune responses with less risk of infection
Recent developments:
- Research is ongoing for their application in various diseases, like cancer and flu, showcasing their potential beyond infectious diseases.
Viral Vector Vaccines
Viral vector vaccines use a harmless virus to deliver genetic material from the target pathogen into the body’s cells. This method results in the production of antigens that stimulate an immune response.
Notable examples include:
- Johnson & Johnson COVID-19 vaccine
- AstraZeneca COVID-19 vaccine
Operating Mechanism:
- Utilization of a different virus as a delivery system
- Induces both antibody and cell-mediated immune responses
Applications in infectious disease control:
- Effective against diseases like Ebola, prompting interest in their rapid development for COVID-19
- Vectors can be adjusted for different diseases, enhancing versatility
By classifying vaccines this way, we can better implement vaccination strategies tailored to specific diseases and populations, ultimately improving health outcomes.
Mechanism of Action
Understanding the mechanism of action of vaccines is essential not just for healthcare professionals but also for students and researchers studying immunology and public health. It helps to clarify how vaccines trigger the body’s immune responses to provide protection against specific diseases. This section focuses on two main aspects: immune response activation and antibody production, which are crucial for the efficiency of any vaccine.
Immune Response Activation
The activation of the immune response is a key event in vaccine function. When a vaccine is administered, it introduces an inactive or weakened form of a pathogen (like a virus or bacteria) or its components into the body. This exposure is sufficient for the immune system to recognize it as a threat without causing the disease itself.
The immune response involves multiple components. T-cells and B-cells play significant roles in this process. T-cells help in recognizing and responding to antigens presented by the vaccine. They can distinguish between infected and non-infected cells and facilitate the destruction of those that are infected. Meanwhile, B-cells are responsible for producing antibodies, which neutralize pathogens and mark them for destruction.
"The success of a vaccine largely depends on its ability to activate the immune system effectively."
Furthermore, the response can be either humoral or cell-mediated. Humoral immunity primarily involves antibodies that circulate in blood, while cell-mediated immunity relies on T-cells targeting infected cells. Both pathways are essential for long-term immunity and the formation of memory cells, which ensure a quicker response during potential future infections.
Antibody Production
The second significant aspect of vaccination's mechanism of action is the production of antibodies. Antibodies are proteins produced by B-cells in response to an antigen. When a vaccine introduces an antigen, it stimulates these B-cells to proliferate and differentiate into plasma cells, which then secrete specific antibodies against that antigen.
These antibodies bind to the pathogen in various ways:
- Neutralization: Prevents the pathogen from entering or damaging healthy cells.
- Agglutination: Clumps pathogens together, making it easier for immune cells to eliminate them.
- Opsonization: Flags the pathogens for destruction by phagocytic immune cells, such as macrophages.
This antibody production is also crucial for creating long-term immunity. After the initial exposure to the vaccine, memory B-cells retain a record of the pathogens, enabling the body to respond more swiftly during subsequent exposures.
Live Attenuated Vaccines
Live attenuated vaccines hold significant importance in the landscape of immunology and public health. These vaccines utilize a weakened form of the pathogen that causes disease. This method often triggers a robust immune response, mimicking the natural infection without causing the actual illness. The immune system develops defense mechanisms, including both cellular and humoral immunity, providing long-lasting protection.
Live attenuated vaccines can potentially confer stronger immunity compared to inactivated vaccines. They promote comprehensive immune responses, allowing the body to combat the disease effectively upon subsequent exposure. However, there are essential considerations regarding their use. "Live" viruses may pose risks to individuals with compromised immune systems and may also require careful storage and handling to maintain their effectiveness.
Examples of Live Attenuated Vaccines
Several well-known vaccines are based on the live attenuated approach. Examples include:
- Measles, Mumps, and Rubella (MMR) vaccine: This combined vaccine protects against three viral infections, demonstrating the efficacy of live attenuated techniques.
- Varicella (Chickenpox) vaccine: The varicella vaccine reduces the risk of contracting chickenpox, which can lead to serious complications.
- Oral Polio Vaccine (OPV): Used widely in the past, this vaccine has significantly reduced polio incidence globally.
- Rotavirus vaccine: This vaccine helps protect infants and young children from rotavirus gastroenteritis, which can lead to severe dehydration.
Advantages and Disadvantages
Live attenuated vaccines come with their own set of advantages and disadvantages, which are crucial for their informed use.
Advantages:
- Strong immune response: They often induce robust immune responses that can provide long-lasting protection.
- Community immunity: They contribute to herd immunity, potentially protecting unvaccinated individuals.
- Less frequent dosing: Some live vaccines confer long-term immunity, possibly reducing the need for multiple doses.
Disadvantages:
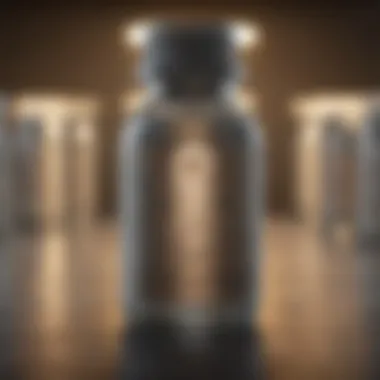
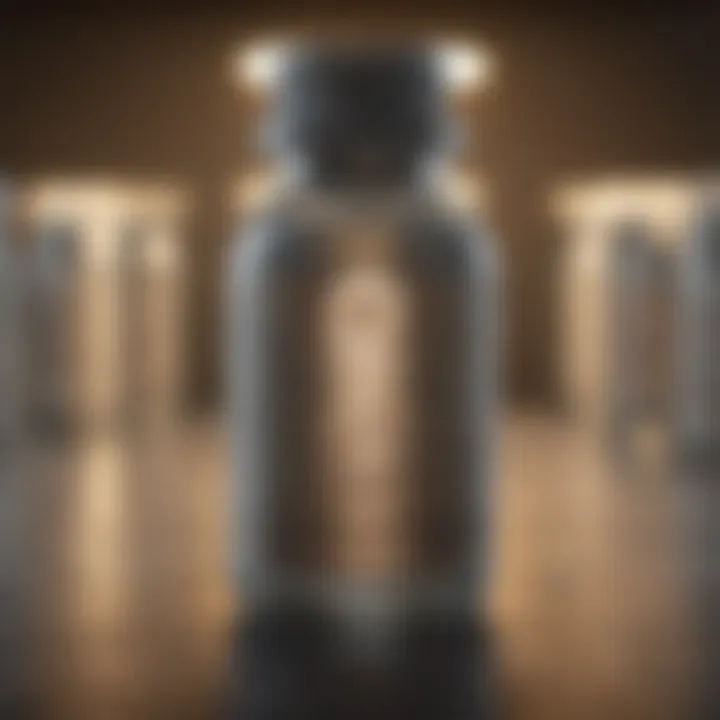
- Safety concerns: Individuals with weakened immune systems may experience adverse reactions.
- Storage requirements: These vaccines typically require strict temperature control to maintain efficacy.
- Potential reversion: There is a slight risk that the vaccine strain can revert to a more virulent form under certain conditions.
Live attenuated vaccines remain a powerful tool in disease prevention, yet they require careful consideration of individual health factors and logistics in distribution.
The landscape of live attenuated vaccines illustrates the delicate balance between effective vaccination strategies and ensuring the safety of diverse populations. Investing in research and understanding these vaccines is equally imperative for future public health initiatives.
Inactivated or Killed Vaccines
Inactivated or killed vaccines are a crucial part of the vaccine landscape. These vaccines are made from viruses or bacteria that have been killed or inactivated, meaning they cannot cause disease. Examples include the polio vaccine and the hepatitis A vaccine. The significance of inactivated vaccines lies in their ability to provoke an immune response without the risk of causing an active infection.
Common Examples
- Polio Vaccine: This vaccine is essential in preventing poliomyelitis, a viral infection that can lead to paralysis. The inactivated polio vaccine was developed to eliminate the risk associated with live vaccines.
- Hepatitis A Vaccine: This vaccine protects against the hepatitis A virus, which causes liver disease. Since the virus is inactivated, the vaccine is safe for individuals, including those with weakened immune systems.
- Rabies Vaccine: This vaccine is given after potential exposure to rabies. It is effective because it contains inactivated rabies virus, ensuring patient safety while providing immunity.
- Influenza Vaccine: The inactivated form of the flu vaccine is commonly used and modified each year to match circulating strains. This effectiveness helps in controlling seasonal outbreaks.
These vaccines have been instrumental in reducing the incidence of the diseases they target.
Benefits and Risks
Inactivated vaccines present several advantages:
- Safety: Since they contain killed pathogens, there is no risk of causing disease in the vaccinated individual.
- Stability: Inactivated vaccines are generally stable and can be stored without the need for special conditions, making them easier to distribute.
- Strong Immune Response: They often stimulate a robust immune response due to the presence of inactivated pathogens, though multiple doses may be necessary.
However, there are risks and considerations to keep in mind:
- Potential for Adverse Reactions: As with any vaccine, inactivated vaccines can cause side effects, such as soreness at the injection site or mild fever.
- Immune Response Limitations: Some inactivated vaccines may not provide as strong or lasting immunity compared to live vaccines. Hence, booster doses might be required.
- Cold Chain Requirements: Some inactivated vaccines need refrigeration, which can be a barrier in areas with limited resources.
In summary, while inactivated or killed vaccines are a cornerstone in the prevention of infectious diseases, they must be integrated with broader vaccination strategies. Understanding their benefits and limitations is vital for effective public health planning.
Subunit, Recombinant, and Conjugate Vaccines
Subunit, recombinant, and conjugate vaccines represent significant advancements in immunization technology. Their development emphasizes the necessity to create targeted, efficient vaccines that offer protection without utilizing live pathogens. Understanding these types of vaccines is crucial in modern vaccine research and public health strategies.
Mechanism of Action
The mechanism of action for subunit, recombinant, and conjugate vaccines revolves around the presentation of specific antigens to the immune system. Unlike traditional vaccines that may introduce whole pathogens, these vaccines utilize only parts of the pathogen, such as proteins or sugars.
- Subunit vaccines contain purified pieces of the virus or bacterium. These pieces are usually proteins that have been isolated and inactivated. The immune response is initiated when these proteins are recognized by the immune cells, leading to the production of specific antibodies.
- Recombinant vaccines take this a step further by using genetic engineering to produce antigens. For instance, a gene coding for a viral protein may be inserted into bacteria or yeast. These microorganisms then produce the desired protein, which is harvested and used in the vaccine formulation.
- Conjugate vaccines link carbohydrates from the bacterial surface to a carrier protein. This combination allows the immune system to recognize the carbohydrate, which is often poorly immunogenic by itself. When linked to a protein, the immune response is significantly enhanced, leading to effective antibody production against the carbohydrate.
The immune response generated from these vaccines often results in long-lasting immunity, relying on both humoral and cellular components of the immune system.
Clinical Applications
Subunit, recombinant, and conjugate vaccines have a wide range of clinical applications. Here are some prominent examples:
- Hepatitis B Vaccine: This recombinant vaccine, using the surface antigen of the Hepatitis B virus, is widely administered to prevent liver infection. Its effectiveness has made it a routine part of many vaccination schedules.
- Human Papillomavirus (HPV) Vaccine: This also utilizes a recombinant approach, preventing types of HPV known to cause cervical cancer.
- Pneumococcal Conjugate Vaccine: This vaccine protects against pneumonia and other infections caused by Streptococcus pneumoniae. The conjugation of polysaccharides to proteins enhances immune recognition and efficacy.
These vaccines are critical in reducing the incidence of preventable diseases and have proven especially useful in vulnerable populations, including children and immunocompromised individuals.
The development of subunit, recombinant, and conjugate vaccines illustrates the move towards safer vaccination strategies, leveraging scientific advances to produce effective immune responses without the risks associated with live pathogens.
In summary, subunit, recombinant, and conjugate vaccines play an instrumental role in modern immunization efforts. Their ability to generate targeted immune responses with reduced risks has positioned them as a cornerstone in the fight against infectious diseases. Understanding their mechanisms and applications is essential for public health professionals and researchers alike.
Messenger RNA Vaccines
Messenger RNA (mRNA) vaccines represent a breakthrough in vaccine technology. Unlike traditional vaccines that often use inactivated microorganisms or protein subunits, mRNA vaccines provide instructions to cells to produce a protein that is part of the virus. This new class of vaccines played a critical role in the rapid response to the COVID-19 pandemic. The importance of understanding mRNA vaccines lies in their potential to address various infectious diseases efficiently and their implications for future vaccine development.
How mRNA Vaccines Work
mRNA vaccines operate by using a synthetic version of messenger RNA. When injected into the body, this mRNA enters cells and instructs them to produce a harmless piece of the target virus's spike protein. This protein then triggers an immune response. The body recognizes it as foreign and produces antibodies against it. To elaborate:
- Delivery: Once administered, lipid nanoparticles help deliver the mRNA into the cells.
- Translation: Inside the cells, ribosomes read the mRNA and synthesize the viral protein.
- Immune Activation: The immune system identifies this protein as non-self, mounts a response, and remembers it for future encounters with the actual virus.
With this approach, the body can quickly recognize and attack the virus if exposed. This swift response can be crucial in combating real infections.
Recent Developments
The realm of mRNA vaccine technology is evolving rapidly. Initially developed for COVID-19 by Pfizer-BioNTech and Moderna, researchers are exploring this platform for applications beyond SARS-CoV-2. Some noteworthy developments include:
- Cancer Vaccines: Research is underway to use mRNA for personalized cancer treatments by targeting specific tumor antigens.
- Universal Influenza Vaccine: Scientists are investigating the potential for mRNA technology to create more comprehensive vaccines against influenza strains.
- Zika and Rabies: Clinical trials are ongoing to ascertain the effectiveness of mRNA vaccines against these viruses.
These advancements highlight the flexible nature of mRNA vaccines, making them applicable in various contexts. As researchers continue to explore this technology, it could reshape how vaccines are conducted and developed in the future.
The potential of mRNA vaccines suggests a new era in infectious disease prevention and treatment, promising quicker, more effective responses to emerging health threats.
Understanding mRNA vaccines and their capabilities will be crucial for students, researchers, and healthcare professionals as they navigate the ever-changing landscape of vaccine science.
Viral Vector Vaccines
Viral vector vaccines have gained significant attention in recent years, particularly during the global response to pandemics. These vaccines utilize modified viruses to deliver genetic material into human cells. This approach falls under the category of innovative vaccine technologies and plays a critical role in combating infectious diseases. Understanding their benefits and considerations is vital for students, researchers, and healthcare professionals alike.
Operating Mechanism
The core principle behind viral vector vaccines revolves around using harmless viruses as delivery vehicles. In most cases, a virus is altered so it cannot cause disease. For instance, in the case of the Oxford-AstraZeneca vaccine, a modified version of the chimpanzee adenovirus (ChAdOx1) is employed. This modified virus carries genetic instructions for a protein found on the surface of the target virus, be it SARS-CoV-2 or another pathogen.
Once administered, the viral vector enters human cells and uses their machinery to produce the target protein. This prompts the immune system to recognize the foreign protein as a potential threat. Consequently, the immune response is activated, leading to the production of antibodies and the activation of immune cells. This sets the stage for a robust defense against future exposures to the actual virus.
"Viral vector vaccines represent a breakthrough in vaccine technology, helping to deliver targeted immune responses effectively."
Applications in Infectious Disease Control
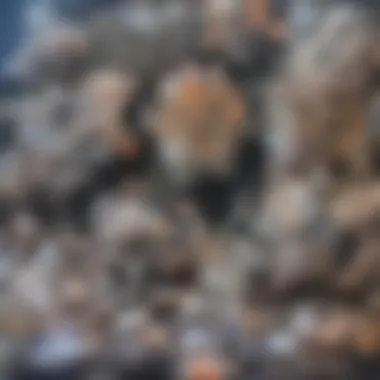
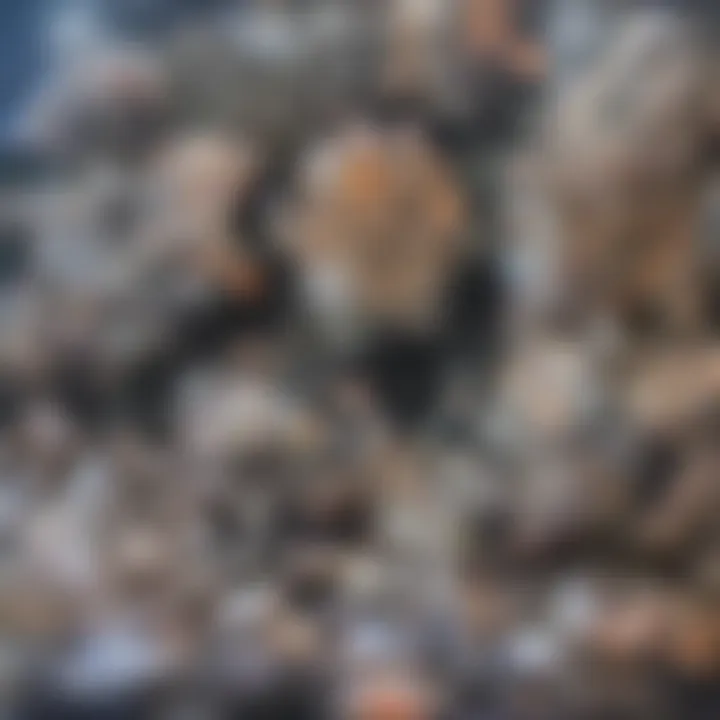
Viral vector vaccines have shown promise in various applications, particularly in the control of infectious diseases. Some key applications include:
- Ebola Virus Disease: The rVSV-ZEBOV vaccine utilizes a viral vector to produce an Ebola virus protein. It has been shown to be effective in preventing Ebola outbreaks.
- COVID-19: The Johnston & Johnston and Oxford-AstraZeneca vaccines have played crucial roles in the fight against SARS-CoV-2. Their rapid development showcased the potential of viral vector technologies to respond to emerging diseases swiftly.
- Zika Virus: Research is ongoing regarding the utility of viral vectors to create vaccines for Zika virus, which is transmitted by mosquitoes and can have severe effects during pregnancy.
The diversity of diseases these vaccines address highlights their adaptability and importance. Their effectiveness depends on factors such as the choice of vector, the targeted immune response, and population demographics. Thus, ongoing research is essential to optimize these vaccines for maximum public health impact.
Common Vaccines and Their Targets
Understanding the types of vaccines is essential for grasping the broader landscape of public health. Vaccines play a pivotal role in preventing diseases, reducing morbidity and mortality rates in populations. Each vaccine has specific targets, addressing various pathogens that threaten human health. The categorization of vaccines based on their targets helps clarify the specific roles they play in disease prevention. Additionally, it provides insight into the ongoing efforts in vaccine development aimed at tackling emerging infections.
A well-designed vaccination program can lead to herd immunity, protecting those who cannot be vaccinated. This underlines the significance of comprehensive vaccination strategies that consider both viral and bacterial infections.
Vaccines for Viral Infections
Viral vaccines serve as a foundational element in the arsenal against viral diseases. These vaccines stimulate the immune system to recognize and combat specific viruses. Common examples include the measles, mumps, and rubella (MMR) vaccine, as well as the influenza vaccine. These vaccines primarily function by introducing harmless components of the virus into the body, allowing for an immune response without causing disease.
Key examples of viral vaccines include:
- Measles, Mumps, and Rubella (MMR) Vaccine: This vaccine protects against three serious viral infections.
- Influenza Vaccine: Given annually, this vaccine targets different influenza strains, adapting each year to current variants.
- Hepatitis B Vaccine: This vaccine is essential for preventing liver disease and cancer related to hepatitis B infections.
The major benefit of these vaccines is their effectiveness in reducing the incidence of viral infections. However, challenges like vaccine hesitancy and the evolution of viral pathogens complicate the landscape. Education about the importance and safety of these vaccines remains crucial in maintaining high vaccination rates.
Vaccines for Bacterial Infections
Bacterial vaccines play a vital role in combating diseases caused by bacterial pathogens. They are designed to elicit a strong immune response against specific bacteria, resulting in long-lasting immunity. Vaccines like the DTaP vaccine (diphtheria, tetanus, and pertussis) and the pneumococcal vaccine exemplify successful bacterial vaccination strategies.
Common bacterial vaccines include:
- DTaP Vaccine: Protects against diphtheria, tetanus, and pertussis. It is a fundamental childhood vaccination.
- Pneumococcal Vaccine: Reduces the incidence of pneumonia and other serious infections caused by Streptococcus pneumoniae.
- Haemophilus influenzae type b (Hib) Vaccine: Protects against bacterial infections, especially in young children.
Despite the success of bacterial vaccines, challenges persist. Variability in public acceptance and the emergence of antibiotic-resistant bacteria necessitate continued emphasis on vaccination. It is essential for healthcare professionals to advocate for vaccination as a primary preventive measure against bacterial infections. These vaccines not only safeguard individual health but also contribute to public health by controlling outbreaks and preserving antibiotic efficacy.
"Vaccines are a tool for prevention, essential for safeguarding community and individual health."
By improving awareness regarding these vaccines and their targets, one can foster a more informed public stance on vaccination programs, ultimately promoting a healthier society.
Challenges in Vaccine Development
Vaccine development is a complex process that presents numerous challenges. Understanding these challenges is essential for students, researchers, and healthcare professionals. It provides insight into how vaccines are made, their effectiveness, and how they can be improved. The challenges faced can significantly impact public health outcomes. Addressing these issues can lead to better vaccine formulations and distribution strategies.
Adverse Reactions
Adverse reactions to vaccines are a primary concern in vaccine development. These reactions can vary from mild symptoms, such as soreness at the injection site, to more severe effects, although the latter are quite rare. Understanding the nature and frequency of these reactions is crucial. Researchers conduct various clinical trials to assess the safety profile of vaccines before they gain approval.
- Possible minor side effects include:
- Rare but severe adverse effects might involve:
- Fever
- Fatigue
- Headache
- Allergic reactions
- Thrombocytopenia
The reporting system for adverse events after vaccination, like the Vaccine Adverse Event Reporting System (VAERS) in the United States, plays an important role in monitoring safety post-licensure. Moreover, public trust in vaccination can be affected by reported reactions, emphasizing the need for transparent communication about potential risks and benefits.
Global Vaccine Distribution Issues
Global distribution of vaccines presents significant challenges, which can hinder public health efforts. Factors such as logistics, market dynamics, and economic disparities contribute to these distribution hurdles.
Key distribution issues include:
- Cold chain requirements: Many vaccines require temperatures below zero degrees Celsius, making transportation and storage more complex.
- Supply chain vulnerabilities: Disruptions, such as natural disasters or political instability, can impede the distribution process.
- Access disparities: Developing countries often face shortages and limited access due to financial constraints.
- Regulatory barriers: Different countries have varying regulatory requirements for importing vaccines, complicating the approval process.
"Addressing the global challenges of vaccine distribution is essential for achieving equitable health outcomes and preventing disease outbreaks across populations."
Future Trends in Vaccination
The field of vaccination is rapidly evolving, influenced by advancements in science and technology. Future trends in vaccination are crucial for improving public health outcomes. Innovations in this area promise to enhance vaccine effectiveness, accessibility, and targeted responses to various diseases. This section delves into the upcoming trends and highlights their significance in current vaccination strategies.
Next Generation Vaccines
Next generation vaccines refer to novel vaccine technologies that improve upon traditional methods. These innovations leverage genetic engineering, nanotechnology, and more sophisticated adjuvants. Examples include nanoparticle vaccines and vector-based technologies.
The benefits of next generation vaccines are substantial. They can be designed to offer broader immunity against multiple strains of a virus. Moreover, they often require fewer doses than traditional vaccines, facilitating easier mass immunization. Advantages like stability at room temperature also enhance logistics, especially in regions with limited cold storage facilities.
"The future of vaccines lies in harnessing advanced technologies, ensuring faster response times to emerging health threats."
Personalized Vaccination Approaches
Personalized vaccination approaches focus on tailoring vaccines to an individual's genetic and immunological profile. This method holds promise for various diseases, particularly cancers and chronic infections. Rather than a one-size-fits-all approach, personalized vaccines can address specific markers present in an individual’s immune response.
This trend in vaccination offers several benefits:
- Enhanced efficacy by ensuring that the vaccine provokes a strong immune response tailored to the individual's needs.
- Reduced adverse reactions by minimizing inappropriate immune responses to antigens not relevant to the patient.
- Greater potential for preventative therapy by aligning vaccines with the patient’s unique risks and characteristics.
As research progresses, the integration of genetic data into vaccine development may revolutionize the way we approach immunization. By adopting personalized vaccination strategies, healthcare providers can address the specific diseases in the context of each patient's profile, ultimately leading to better health outcomes.
Epilogue
The significance of vaccines extends beyond individual protection. Vaccines contribute to herd immunity, reducing the spread of infectious diseases and protecting vulnerable populations. As more people are vaccinated, the chances of outbreaks diminish, leading to a healthier society overall.
Implications for Public Health
Vaccines play a vital role in enhancing public health across multiple dimensions.
- Disease Prevention: Vaccination has proven effective in controlling and eradicating diseases that once caused widespread morbidity and mortality. Diseases such as polio and measles have seen dramatic declines due to vaccination programs.
- Economic Impact: Reducing disease prevalence translates to lower healthcare costs. Fewer hospitalizations, less need for medical interventions, and a healthier workforce contribute to economic stability.
- Global Health Security: Vaccination is fundamental in the fight against emerging infectious diseases. By fostering global collaboration in vaccine development and distribution, we can mitigate the impact of potential pandemics.
- Addressing Vaccine Hesitancy: To maximize the effectiveness of vaccination programs, addressing public concerns and misinformation is crucial. Educational efforts must focus on the benefits of vaccination and emphasize the risks associated with unvaccinated populations.
"Vaccines are one of the most powerful tools we have for public health."