Understanding 12 Well Plate Dimensions for Optimal Lab Use
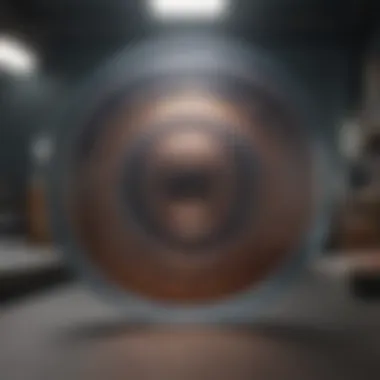
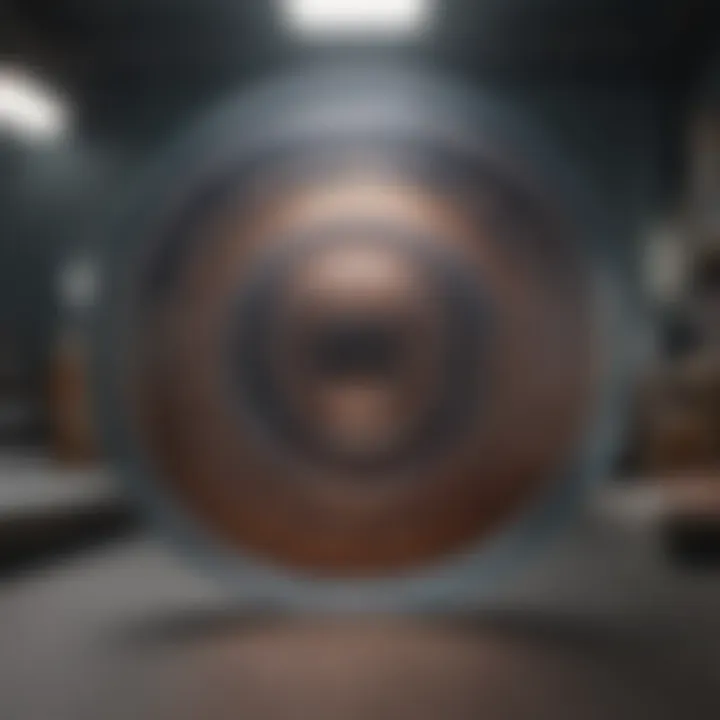
Intro
The dimensions of 12 well plates hold significant importance in laboratory settings. They play a crucial role in various experiments and research projects. Understanding these dimensions allows researchers and educators to select the most suitable plates for their specific needs. By examining physical characteristics and functional variations, this article aims to provide stakeholders with the knowledge needed for informed decisions.
Research Methodology
Description of Research Design and Approach
The research design selected for this exploration employed a qualitative approach. The aim was to gather detailed information about 12 well plate dimensions. This included a consideration of specifications and variations among different manufacturers. To achieve this, a thorough review of existing literature was performed. Peer-reviewed journals, product specifications, and reliable laboratory resources were integral.
Materials and Methods Used in the Study
The study consisted of primary and secondary sources. Several products were analyzed, including brands like Corning and Thermo Fisher Scientific. Technical specifications such as depth, diameter, and spacing of wells were evaluated. Specifics included:
- Well Diameter: Generally around 16 mm.
- Well Depth: Typically varies from 10 mm to 15 mm.
- Plate Dimensions: A standard 12 well plate is approximately 3.5 x 5 inches.
Data collected helped in creating a comprehensive overview of 12 well plates used across scientific fields. Practitioners can reference this data when making choices for their experimental designs.
Discussion and Interpretation
Interpretation of Results in the Context of Existing Literature
The findings align with existing literature, highlighting the necessity of well plate dimensions in experimental success. Studies demonstrate that appropriate dimensions maximize sample yield and aid in accurate data collection. Variations in size can impact assay compatibility and the volume of reagents needed.
Implications for Future Research or Practical Applications
Understanding dimensions can lead to improved experimental design. For example, choosing the right well plate can minimize error rates and enhance reproducibility. Future research might focus on innovative materials or coatings for better performance. Manufacturers are called to prioritize usability based on these findings.
Ultimately, this exploration underscores the importance of well plate dimensions in achieving reliable and accurate laboratory results.
In summary, this detailed examination of 12 well plate dimensions equips the reader with the data and insights necessary for effective lab practice. With this nuanced understanding, students, researchers, and professionals alike can navigate their scientific endeavors with greater confidence.
Intro to Well Plates
Laboratory experiments often rely on standardized equipment to ensure consistent and reliable results. The 12 well plate is a pivotal tool in various scientific fields, particularly in biological and biochemical research. Understanding the dimensions and specifications of 12 well plates can significantly enhance experimental design. Selecting the appropriate well plate can influence the accuracy of test results, ease of handling, and overall throughput of the experiment.
Purpose of Well Plates
The primary purpose of 12 well plates is to provide a controlled environment for conducting multiple experiments simultaneously. Each well can hold different samples, allowing researchers to explore various conditions or concentrations in a single run. This multi-well format reduces reagent usage and labor, thereby increasing efficiency.
Additionally, 12 well plates are designed to promote reproducibility, which is essential in scientific research. By maintaining uniform dimensions and surface characteristics, researchers can ensure that their experiments yield comparable results across different trials. A standardized format also allows for easier sharing of data among the scientific community.
Common Applications in Research
12 well plates are versatile and widely used across numerous fields. In cell biology, they serve as culture vessels for incubating cells under varied conditions. Researchers often use them for drug testing, toxicity screening, and even gene expression studies. Their compact design is suitable for high-throughput screening methods, which are crucial in drug discovery.
In addition to biological applications, 12 well plates can be employed for biochemical assays. Enzyme kinetics, protein assays, and other molecular biology protocols frequently utilize them. Their ability to hold small volumes of solutions makes them ideal for reactions requiring limited reagents.
Overall, understanding the dimensions and usage specifics of 12 well plates is essential for optimizing laboratory workflows. This knowledge not only aids in experiment design but also facilitates accurate data interpretation, ultimately leading to better scientific outcomes.
Physical Dimensions of Well Plates
The physical dimensions of 12 well plates are crucial to various experimental procedures. Understanding these dimensions helps in selecting the right plate for specific applications. It allows researchers to effectively plan experiments, ensuring repeatability and reliability of results. Accurate knowledge of dimensions directly influences the well plates’ compatibility with existing laboratory equipment and protocols while optimizing workflows.
Standard Well Dimensions
Diameter
The diameter of each well in a 12 well plate typically ranges from 16 to 25 millimeters. This measurement is significant as it governs the surface area available for cell growth or reagent reaction. A larger diameter can enhance cell spreading and nutrient uptake, making it a popular choice for cell culture experiments. The key characteristic of diameter is its direct impact on the well's effective use in various tests.
However, selecting a diameter that’s too large might lead to unnecessary reagent usage. Conversely, a diameter that’s too small can limit the volume of samples or culture medium, impacting the experiment’s outcome. Therefore, researchers should carefully consider well diameter based on their experimental needs and objectives.
Depth
Depth refers to how deep each well is, which usually ranges from 20 to 30 millimeters in standard 12 well plates. The depth is critical because it affects how many cells can be grown within a well and the total volume of liquid it can accommodate. A deeper well may be beneficial for cultures requiring greater fluid volumes, aiding in minimizing evaporation during longer experiments.
The depth of the wells often improves the retention of samples during mixing or agitation. However, deeper wells may present challenges for accessibility during pipetting. This makes calibration of depth essential for efficient experimental design, as it has to harmonize with pipetting techniques being used in the laboratory.
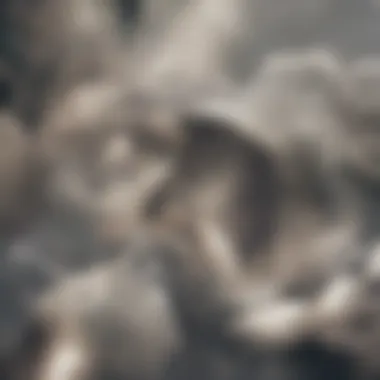
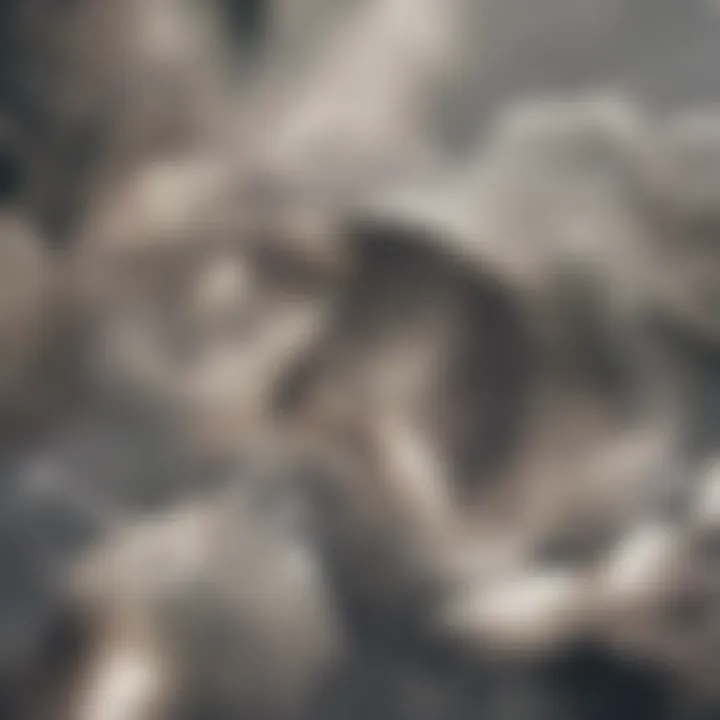
Volume Capacity
Volume capacity of standard 12 well plates typically encompasses a range of 3 to 5 milliliters per well. This specification is vital as it determines the maximum amount of reagents, samples, or cell cultures that can be employed in experiments. Ample volume capacity helps in achieving better experimental accuracies and ensuring that reactions have enough substrates available.
The unique feature of volume capacity is its ability to allow for diverse applications, from high-throughput screening to drug testing. Too low a volume capacity might lead to limited experimental options while higher capacities may restrict the number of samples manageable in a single batch. Therefore, it is important to select wells with adequate capacity as per the experimental requirements.
Variations in Plate Dimensions
Variations in plate dimensions can effectively expand the functionality of 12 well plates in laboratory settings.
Microplate Formats
Microplate formats extend beyond the standard 12 well arrangement. They include various configurations such as 24, 48, or 96 well plates designed to cater to specific experimental protocols. The notable characteristic of microplate formats is their scalability, allowing researchers to conduct multiple experiments simultaneously or perform high-throughput screenings.
Utilizing microplate formats enhances efficiency, enabling higher sample throughput without significant increases in laboratory space. However, researchers should ensure that the formatting aligns with their lab’s existing equipment. Compatibility issues may arise if the formats are not standardized, leading to difficulties in data analysis and sample handling.
Other Well Configurations
In addition to standard dimensions, there are other well configurations that can offer unique benefits. For instance, some plates feature conical or flat-bottom wells, which influence cell attachment and growth. Conical wells often facilitate easier pipetting, while flat-bottom wells are advantageous for applications requiring uniform surface access.
These configurations are significant as they can greatly influence experimental results. Choosing the right configuration can enhance assay performance but may also lead to increased costs associated with specialized plates. It is essential for researchers to analyze the requirements of their specific experiments and select the well configuration that best meets their needs.
Understanding the physical dimensions and variations of 12 well plates is a linchpin in experimental design, significantly impacting protocols and outcomes.
Material Considerations
When selecting a 12 well plate, material considerations play a crucial role in its effectiveness and suitability for specific experiments. Different materials exhibit various physical and chemical properties that can directly affect the outcome of laboratory procedures. This section explores the types of materials commonly used in 12 well plates and their influence on experimental results.
Types of Materials Used
Polystyrene
Polystyrene is one of the most widely used materials for 12 well plates due to its favorable properties. It is lightweight and has excellent optical clarity, which is essential for assays that require visual readings. The key characteristic of polystyrene is its ability to be molded into different shapes and sizes, allowing for uniform dimensions that conform to standard microplate specifications. This makes it a popular choice in many laboratory settings, particularly for cell culture and enzyme-linked immunosorbent assays (ELISAs).
A unique feature of polystyrene is its surface treatment options. Plates can be treated to enhance cell adherence, benefiting experiments where cell attachment is necessary. However, it is essential to note that polystyrene is prone to leaching substances that may interfere with sensitive experiments. This factor is an important disadvantage to consider when planning experiments that require high accuracy.
Polypropylene
Polypropylene offers a different profile compared to polystyrene. It is known for its chemical resistance and durability, making it suitable for experiments involving harsh solvents or extreme conditions. The key characteristic of polypropylene is its high melting point, which allows autoclaving and reuse, thereby making it an economical choice for many labs.
Moreover, polypropylene plates have a unique feature that competes well with polystyrene: they are less likely to undergo adsorption of biomolecules, leading to more reliable results in assays. Advantages of using polypropylene include reduced risk of contamination and better compatibility with aggressive compounds. However, polypropylene does not provide the same optical clarity as polystyrene, which can limit its use in applications requiring visual assessment.
Glass and Others
Glass plates are used in specific applications where precision and scientific integrity are paramount. One of the key characteristics of glass is its non-reactive nature, preventing biochemical interference during experiments. This property makes glass a beneficial choice for applications necessitating high accuracy.
A unique feature of glass plates is their ability to be easily cleaned and sterilized, ensuring no residual contaminations affect experimental results. While glass offers several advantages like superior optical clarity and chemical inertness, it is important to acknowledge its disadvantages: glass plates can be heavier and more fragile than plastic alternatives. They are also generally more expensive, which could be a consideration for labs with budget constraints.
Influence of Material on Experiments
The material of a 12 well plate can significantly impact experimental outcomes. Different materials can interact differently with reagents or other substances used in experiments. For instance, adsorption characteristics vary; while polystyrene may bind proteins, polypropylene tends to minimize this issue. It is this interaction that affects cellular behavior, assay efficiency, and ultimately, the reliability of experimental results.
When choosing a well plate, researchers must consider these factors to ensure their material choice aligns with their experiment's requirements. The compatibility of the chosen material with the biological system or assay type is crucial. Thus, an in-depth understanding of the material properties is essential for optimizing experimental design.
Technical Specifications
Understanding the technical specifications of 12 well plates is essential for maximizing their utility in laboratory research. These specifications influence how the plates are used, the compatibility with experiments, and overall experiment outcomes. Proper understanding of technical details, such as sealing options and surface treatments, aids researchers in choosing the right products for specific applications. Also, it can ultimately enhance data reliability and reproducibility in the lab.
Sealing Options
Non-Sterile vs. Sterile
Non-sterile and sterile sealing options have different implications for laboratory work. Non-sterile options are usually more cost-effective, making them a popular choice for less sensitive experiments. They tend to be produced in bulk, which allows accessibility to numerous laboratory settings. In contrast, sterile sealing is crucial for experiments where contamination can skew results. Sterile plates are treated using gamma irradiation or ethylene oxide, ensuring that they are free from viable organisms.
Key characteristic of using non-sterile plates is their availability and lower cost, which can benefit budget-constrained labs. However, this option does require extra care to maintain a contamination-free environment during handling. Sterile plates, although more expensive, provide peace of mind in terms of minimizing the risk of microbial contamination during sensitive experiments. Their unique advantage lies in ensuring that results are due strictly to the experimental variable being tested, rather than external factors.
Types of Seals
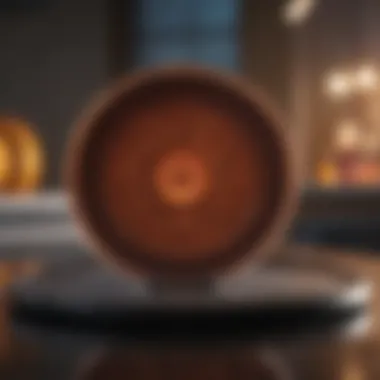
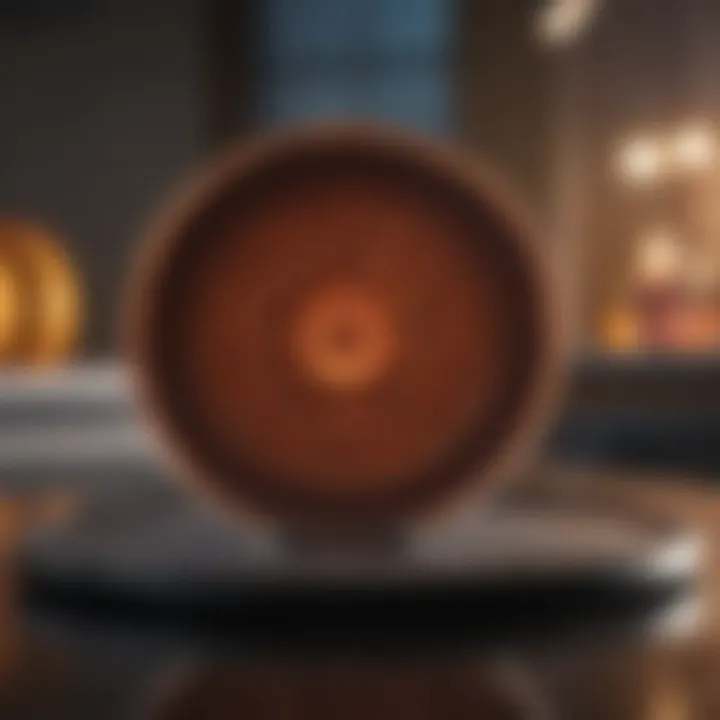
Different types of seals contribute significantly to the protection of samples in 12 well plates. Common seals include adhesive films, lids, or heat-sealed membranes. Adhesive films are often favored for their ease of use and ability to create a nearly airtight environment. This helps prevent evaporation, which is particularly vital in long-term cell culture experiments.
Each seal type has distinct advantages. For example, heat-sealed membranes can provide a more secure and sterile environment, while adhesive films allow for easier access to wells. On the downside, the adhesive might lose its efficacy over extended periods, leading to potential evaporation. In contrast, heat seals might be challenging to remove without damaging the plates. Choosing between these seals requires careful consideration of the specific experiment needs and timeframes involved.
Surface Treatments
Coated Surfaces
Coated surfaces play a pivotal role in enhancing the function of 12 well plates. These plates can be coated with substances like extracellular matrix proteins or poly-D-lysine to promote cell attachment and growth. Such treatment increases cell viability and adherence, making it invaluable for cell-based assays.
The key advantage of using coated plates is their ability to support various cell types that might struggle to adhere to standard surfaces. As an example, certain sensitive cell lines thrive better in coated environments, translating into more reliable experimental outcomes. However, these coatings often have a finite lifespan, and the treatment can alter the overall characteristics of the plate, which might affect subsequent experiments.
Non-Coated Surfaces
Non-coated surfaces represent the standard design of 12 well plates. These are generally made from polystyrene or polypropylene and are suitable for many basic laboratory applications. Non-coated plates are particularly advantageous for assays that do not require specific cell adhesion or where the natural behavior of cells in suspension is being studied.
The primary benefits of non-coated surfaces include lower costs and broader availability. They simplify the experimental setup since they do not require additional treatments. However, some researchers may find limitations in cell attachment when working with specific cell lines or sensitive assays. Choosing non-coated plates is often dictated by the experiment type and the specific requirements of the cells being used.
Choosing the Right Well Plate
Choosing the right 12 well plate is critical for the success of any laboratory experiment. A well-chosen plate can enhance reliability and reproducibility in results. Selecting an appropriate plate involves understanding various elements such as the experiment’s purpose and compatibility with existing equipment. The wrong choice can lead to issues that affect data quality, efficiency, and even safety in the lab.
Factors to Consider
Experiment Purpose
The purpose of the experiment directly influences the selection of the 12 well plate. Different experiments require different types of surfaces and well capabilities. For example, cell culture experiments necessitate plates with treated surfaces to support cell adhesion and growth. On the other hand, assay development might focus on plates with specific well volumes to minimize reagent consumption. Therefore, defining the experiment's goals is key.
This consideration is beneficial because it helps refine the selection process. The right well plate not only supports the experimental setup but also ensures that results are consistent across trials. A unique feature of this aspect is its focus on experimental precision; choosing a plate optimized for the intended application can dramatically enhance the data quality. However, a potential disadvantage lies in the specificity required; overly specialized plates can limit the versatility for a broader range of experiments.
Compatibility with Existing Equipment
Compatibility with existing equipment is another essential factor. Microplate readers, pipettes, and incubators must be able to accommodate the dimensions and configurations of the chosen plates. A common characteristic of this consideration is the adaptability of well plates to various laboratory setups. Using plates that align with existing tools avoids complications in data processing and sampling.
This aspect is popular because it minimizes additional costs and training associated with new equipment. The unique feature lies in the standardization of laboratory procedures. However, relying heavily on compatibility can limit options, pushing researchers towards specific models that may not be ideal for their needs, reducing flexibility in experimentation.
Assessing Quality and Reliability
Assessing the quality and reliability of 12 well plates is vital. Quality materials ensure that the plates remain intact during experiments, preventing leaks or breakdowns. Reliable sources should also provide consistent manufacturing processes to ensure uniformity in dimensions and surface treatments.
This consistency is crucial for experimental reproducibility.
Researchers should consider well-known manufacturers who routinely test their products for performance. By choosing high-quality plates, researchers can maintain confidence in their experimental data, leading to more credible outcomes.
Experimental Utilization
The application of 12 well plates in laboratory settings goes beyond mere functionality. Understanding how to effectively utilize these plates can significantly enhance experimental outcomes. This section emphasizes the essential practices and considerations that researchers must adopt to maximize the utility of 12 well plates. Key benefits include improved reproducibility of results and increased efficiency in data collection processes.
Best Practices for Use
Proper Pipetting Techniques
Proper pipetting techniques are foundational to ensuring accuracy in experiments using 12 well plates. This practice involves precise liquid handling methods that reduce variability in sample volumes. One key characteristic of proper pipetting is the use of consistent technique when drawing up and dispensing liquids. This method is beneficial because it minimizes the risk of cross-contamination and helps maintain sample integrity.
A unique feature of proper pipetting techniques is their adaptability to different liquid viscosities, which can affect how liquids are dispensed. Using the correct pipette tips designed for various liquids can lead to more accurate results. However, improper pipetting can introduce errors, making training vital for researchers to understand the nuances of this skill.
Maintaining Cell Viability
Maintaining cell viability is crucial for any experimental success, particularly in cell culture studies conducted in 12 well plates. This aspect stresses the importance of ensuring that cells remain healthy throughout the experimental period. A key characteristic is the consideration of environmental factors such as temperature and CO2 levels, which can greatly influence cell health.
This practice is popular among researchers because it directly impacts the quality of data collected. A unique feature is the use of specialized media and supplements that aid in cell survival. However, failure to maintain optimal conditions can result in cell death and flawed experimental outcomes, so it is imperative to implement rigorous monitoring procedures.
Data Collection and Analysis
The effectiveness of data collection and analysis hinges on a structured approach to interpreting results obtained from experiments. Researchers must consider how well the data correlates with the initial hypotheses. A key characteristic of interpreting results is the ability to analyze variations and determine the significance of findings. It is essential because it guides the conclusions drawn from the research.
A unique feature of interpreting results is the application of statistical tools, which provides a framework to validate findings. However, over-reliance on these tools without a thorough understanding of the data set can lead to misleading conclusions.
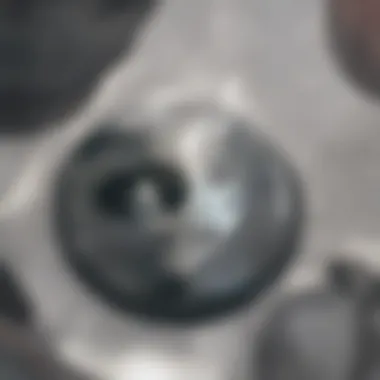
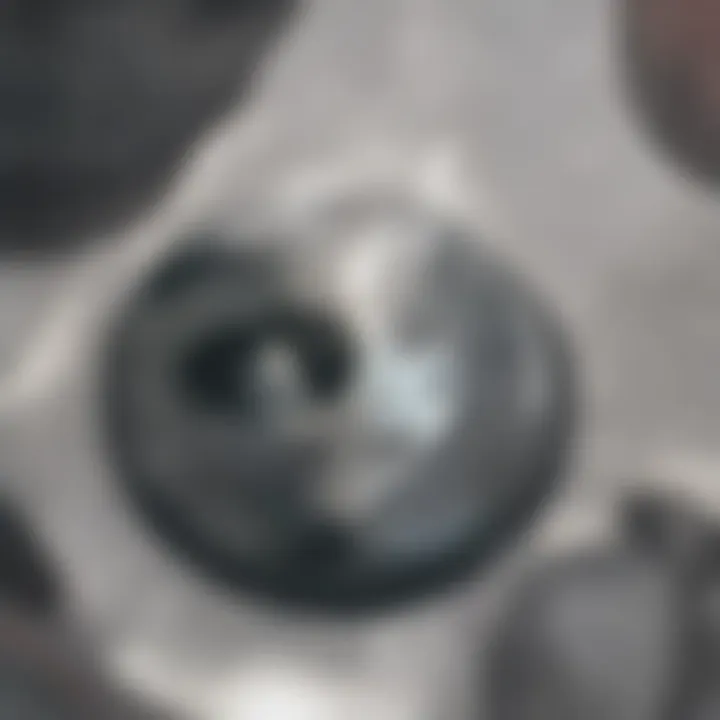
Standardizing Procedures
Standardizing procedures is vital for ensuring consistent results across different experiments using 12 well plates. This practice highlights the need for clear protocols that outline every step in the process, from preparation to data analysis. A key aspect is ensuring that all researchers involved are following the same guidelines. This consistent approach increases the reliability of the results obtained.
A unique feature of standardizing procedures is the establishment of controls and benchmarks. These allow researchers to compare their results against a known standard. However, overly rigid procedures can stifle creativity in experimental design, so a balance must be struck between standardization and adaptability.
Limitations and Challenges
Understanding the limitations and challenges faced in the use of 12 well plates is crucial for making informed decisions in experimental design. This section aims to delve into specific issues that can arise when working with these laboratory tools and offers solutions on how to mitigate them. Being aware of these challenges not only helps in optimizing experiments but also ensures reliability in results.
Potential Issues with Well Plates
Cross-Contamination
Cross-contamination is a prevalent issue that affects the integrity of experimental results. This problem typically arises when reagents or samples are unintentionally transferred from one well to another. Such contamination can lead to skewed results and make it difficult to replicate findings. The key characteristic of cross-contamination is that it can occur in various forms, including aerosol generation or fluid leakages. This aspect makes awareness and prevention strategies essential for anyone utilizing 12 well plates. One unique feature of cross-contamination is its often hidden nature; sometimes the contamination may not be immediately visible, complicating the detection process. The consequences include wasted resources, time, and potentially flawed research conclusions.
Limitations in Scaling Up
Scaling up experiments from 12 well plates to larger volume plates or applications presents its own set of challenges. One significant limitation is that the conditions used in a small scale may not directly translate to a larger setup. Factors such as nutrient availability and oxygen diffusion can behave differently in larger volumes. The key characteristic of these limitations is often related to biological systems' nonlinear responses to changes in concentration or volume. The unique feature of scaling up is that protocols optimized for 12 well plates might require significant adjustments for larger scales. Disadvantages may include increased complexity in data interpretation and additional experimental validation that can extend project timelines.
Mitigation Strategies
Quality Control Measures
Implementing quality control measures is foundational in addressing the limitations discussed. These measures help in maintaining the integrity of reagents and samples. Regular audits of the lab environment, protocols, and sample handling are essential. The key characteristic of quality control measures is their systematic approach, which promotes a higher standard among laboratory practices. Moreover, these measures are beneficial in minimizing variability in experimental outcomes. One unique feature of quality control is that it creates a framework for consistency across different experiments, ensuring that each test can be validated against established standards. This consistency is especially important when using 12 well plates across various research projects.
Optimizing Protocols
Optimizing protocols can significantly enhance the reliability of results obtained from 12 well plates. This involves refining experimental conditions and ensuring they are suitable for the specific assay being conducted. The key characteristic of protocol optimization is its adaptive nature; it allows for iterative testing and adjustment based on preliminary results. This practice is beneficial as it helps identify the best conditions for achieving reproducible outcomes. A unique feature of optimizing protocols is the potential for increased efficiency in resource usage, which can lead to cost savings in the long run. Additionally, optimized protocols can shorten experiment duration while maintaining quality, making them indispensable for high-paced research environments.
Future Outlook and Innovations
The future of 12 well plates is shaped by ongoing trends in technology and scientific research. The need for efficiency, reliability, and advanced functionality drives innovations in the design and use of these laboratory tools. In this aspect, understanding the emerging developments is crucial for anyone involved in laboratory experiments. The advancements not only enhance its applications but also help researchers optimize workflows.
Trends in Microplate Technology
Developments in Material Science
Developments in material science play a significant role in the evolution of 12 well plates. New materials help improve the performance of these plates, aiming for enhanced durability and compatibility with various experimental conditions. A key characteristic of these advancements is the introduction of advanced polymers and composites that exhibit better chemical resistance and physical stability compared to traditional materials like polystyrene or polypropylene. These materials reduce issues like leaching or degradation, leading to more reliable results.
A unique feature of these new materials is their ability to be tailored for specific applications. For example, coatings can be applied to make plates more hydrophilic or hydrophobic based on the experiment's needs. This flexibility is a considerable advantage, as it can lead to improved outcomes in experiments involving cell cultures or assays. However, the cost associated with novel materials may pose a challenge for some labs, especially those operating on a limited budget.
Automation in Microplate Handling
Automation in microplate handling represents another important trend in the field. Automated systems for liquid handling are becoming increasingly sophisticated. They allow for precise control over pipetting, reducing the variability that can occur with manual techniques. This aspect significantly contributes to achieving more consistent results across experiments.
One key characteristic of automation is its ability to increase throughput in laboratories. With robotic systems, multiple plates can be handled efficiently, saving researchers valuable time. Additionally, automation minimizes the risk of human error, ensuring that protocols are followed accurately.
Despite its many advantages, the implementation of automation comes with challenges. Initial setup costs can be high, and training staff on the new equipment can take additional resources. Moreover, not all experiments may require such level of automation, potentially leading to underutilization of these systems in smaller labs.
Implications for Research
The implications of these innovations extend into various research fields. They allow scientists to conduct more complex experiments with greater precision. By integrating new materials and automation, researchers can achieve results that were previously difficult to obtain with traditional methods. This could pave the way for breakthroughs in critical areas such as drug discovery and biological research, ultimately enhancing our understanding of complex biological processes.
Epilogue
In the field of laboratory research, the choice of equipment can significantly affect both the outcomes of an experiment and the efficiency of procedures. This article has emphasized the various dimensions and specifications of 12 well plates, making it a crucial resource for students, researchers, educators, and professionals engaged in scientific work. The conclusion serves to synthesize the key findings while reinforcing the importance of an informed choice in selecting the right well plate for specific applications.
When evaluating 12 well plates, researchers must consider factors such as the dimensions of the wells, the material they are composed of, and the technical specifications required for their particular experiments. This understanding is vital because the physical characteristics of these plates directly impact cell growth, reagent interactions, and overall experimental reliability.
Summary of Key Points
- Physical Dimensions: Understanding well dimensions, including diameter, depth, and volume, is essential to ensure that the plates meet the specific needs of the experiments.
- Materials Used: The choice of polystyrene, polypropylene, or glass can affect the results, particularly in sensitive applications such as cell culture.
- Specifications: Sealing options and surface treatments can enhance the usability of well plates, influencing contamination control and the viability of experimental samples.
- Best Practices: Employing proper techniques in pipetting and maintaining conditions conducive to cell health leads to more accurate results and reliable data.
- Limitations: Recognizing challenges like cross-contamination and the difficulties in scaling up experiments is important for planning successful research strategies.
- Trends and Innovations: Keeping abreast of advancements in microplate technology can provide insights into more efficient laboratory workflows and enhanced experimental outcomes.
Final Thoughts on Selection and Use
Selecting the appropriate 12 well plate extends beyond mere dimensions. It requires a thorough understanding of the experimental context. Each laboratory scenario presents unique requirements; thus, researchers should judiciously evaluate their needs, which may include cell type, assay sensitivity, and environmental conditions. For instance, when working with sensitive biological samples, one must ensure that the selected plate minimizes any adverse reactions between materials and reagents.
Moreover, meticulous attention to detail during the preparation and execution of experiments can significantly increase reproducibility. Consistency in methodology reinforces the validity of collected data. Familiarizing oneself with the specifications and applications of various 12 well plates can ultimately lead to optimized lab processes and better scientific discoveries.
"Informed choices in laboratory settings can make the difference between a successful experiment and a failed hypothesis."
By synthesizing information on dimensions, materials, and practical applications, this article aims to empower laboratory professionals to navigate their experiments with confidence and precision.