Understanding ADME: Key Components in Pharmacology
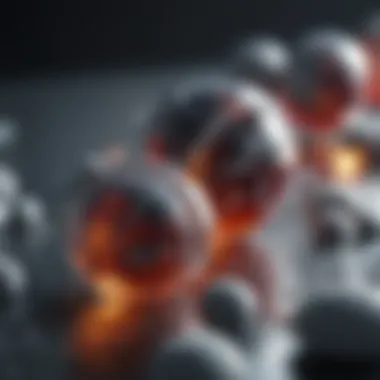
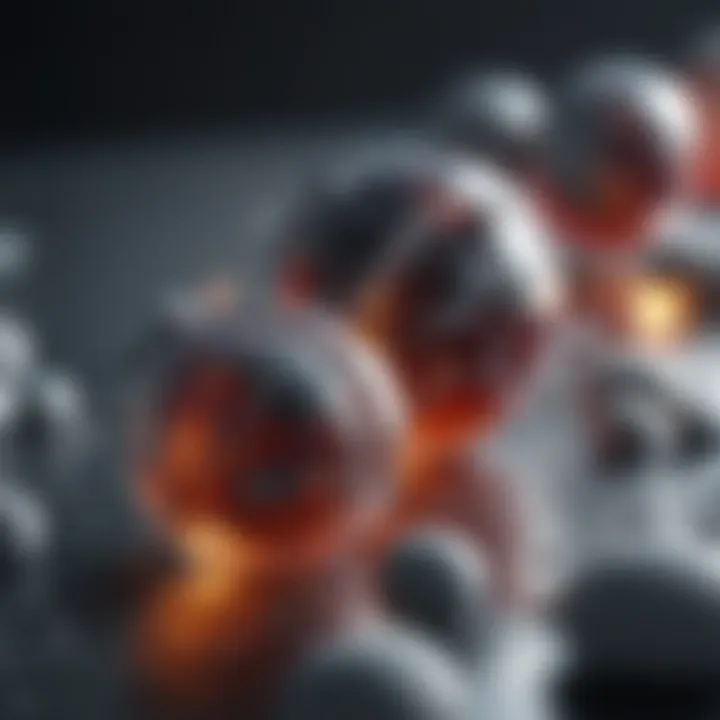
Intro
Understanding the process of drug behavior in the body is crucial in the field of pharmacology. The acronym ADME stands for Absorption, Distribution, Metabolism, and Excretion. These four components are essential for evaluating the pharmacokinetics of a drug. Each segment offers insights into how a drug interacts with the biological system, influencing both its efficacy and safety.
Absorption refers to how a drug enters the bloodstream after administration. It can vary depending on the route of administration, such as oral or intramuscular. Distribution describes how the drug spreads throughout the body and is influenced by factors like blood flow and the drug's affinity for tissues.
The Metabolism phase involves chemical modifications of the drug, primarily in the liver. This process is key for eliminating the active substance or activating prodrugs. Finally, Excretion involves the removal of the drug from the body, mainly via urine or feces. Each of these processes plays a pivotal role in determining the overall therapeutic effectiveness of a medication.
By exploring ADME, we highlight the implications for drug development and patient care. This understanding is particularly relevant in the context of personalized medicine, where treatments are tailored to individual patients based on their unique responses to drugs. As we explore these components further, we will uncover their significance in improving health outcomes and mitigating risks.
Moreover, current trends show a growing interest in the integration of ADME studies with innovative research approaches. The goal is to enhance drug design by considering how these processes affect patients in real-world scenarios. Thus, a thorough comprehension of ADME is essential for researchers, students, educators, and healthcare professionals alike.
Prolusion to ADME
The concept of ADME—Absorption, Distribution, Metabolism, and Excretion—serves as the backbone of pharmacokinetics. Understanding ADME is essential for anyone involved in drug development and therapeutics. It is crucial to recognize how each component influences drug efficacy and safety in patient care.
Defining ADME
ADME is an acronym that encapsulates four key processes that govern the lifecycle of a drug in the body.
- Absorption refers to how a drug enters the bloodstream. This can be influenced by route of administration, formulation, and specific physiological conditions.
- Distribution covers the dispersal of the drug throughout the body and its subsequent reach to various tissues. Factors such as blood flow and tissue affinity come into play here.
- Metabolism involves biochemical transformations that a drug undergoes, primarily in the liver. This process can affect the duration and intensity of a drug's effects.
- Excretion is the elimination of the drug from the body, primarily through urine or feces. This process determines how long a drug remains effective in the system.
Each component must be understood in detail to predict how well a drug will perform and in which patients it will be most effective.
Historical Background of ADME Research
The study of ADME dates back many decades, starting from early pharmacological practices. Initially, the focus was largely on the therapeutic effects of drugs, with less consideration given to their absorption, distribution, metabolism, and excretion.
As scientific understanding advanced, researchers began to recognize that the way a drug was processed in the body could vastly alter its effectiveness and safety profile. During the mid-20th century, the introduction of concepts such as drug half-life and clearance rates laid the foundation for contemporary pharmacokinetics. Methamphetamine and the therapeutic index were among early drugs studied in the context of ADME processes, revealing significant variances in human responses.
Today, understanding ADME has become indispensable in both preclinical and clinical drug development. Regulatory agencies require comprehensive ADME data to ensure that new drugs are both safe and effective. The evolution of ADME research reflects a broader trend towards personalized medicine, where individual patient characteristics can significantly impact drug responses. In summary, the historical context underscores the importance of ADME in constructing effective therapeutic strategies.
Absorption: The First Phase of ADME
Absorption is a critical phase in the ADME process, serving as the entry point for drugs into the systemic circulation. Understanding how drugs are absorbed is vital for pharmacology and drug development. It directly impacts bioavailability, which determines how much of an administered drug reaches its target site at sufficient concentrations. Any variability during this phase can significantly affect therapeutic outcomes and patient safety.
Detailed knowledge of absorption mechanisms assists in formulating more effective drugs. The design of drug delivery systems can be optimized based on these insights to enhance drug efficacy and minimize side effects. Overall, absorption shapes the pharmacokinetic profile of medications, influencing patient therapy outcomes.
Mechanisms of Drug Absorption
Drugs can be absorbed through various mechanisms. The primary routes include passive diffusion, facilitated diffusion, and active transport.
- Passive diffusion occurs when drugs move from an area of higher concentration to one of lower concentration without energy input. This method predominantly applies to lipophilic drugs, that are able to integrate into lipid membranes.
- Facilitated diffusion utilizes specific transport proteins to aid drug movement across membranes. This method does not require energy but is dependent on concentration gradients.
- Active transport involves the use of energy to move drugs against their concentration gradient. This process often allows for the absorption of drugs that are not easily absorbed through passive methods.
Each mechanism plays a role depending on the chemical properties of the drug substance and the characteristics of the absorption site.
Factors Influencing Absorption
Multiple factors affect drug absorption, altering the onset and intensity of drug action. Important factors include:
- Physicochemical properties of the drug, such as solubility and ionization, which can dictate how well a drug is absorbed in specific environments.
- Formulation characteristics, including the presence of excipients that can enhance solubility or protect drugs from degradation.
- Route of administration, where oral, intravenous, and subcutaneous methods each involve different absorption dynamics.
- Gastrointestinal factors, especially for orally administered drugs. These include gastric pH, motility, and the presence of food which can enhance or impede absorption rates.
Understanding these factors is crucial for predicting a drug's behavior within the body, in turn guiding effective dosing regimens.
Clinical Implications of Absorption Variability
Variability in absorption can lead to significant clinical consequences. Differences among individuals, due to factors such as genetics or gastrointestinal health, can lead to varied drug effectiveness and risks of adverse effects. This variability necessitates careful monitoring and sometimes individualized dosing strategies.
- Patient-specific factors, such as age, sex, and underlying health conditions, can have a profound impact on absorption rates.
- Interactions with other medications may inhibit or enhance the absorption of a drug, complicating therapeutic management.
Consequently, healthcare providers must account for these variables when prescribing medication to ensure optimal therapeutic outcomes for each patient. Medication adherence and consistency in administration conditions can also mitigate absorption variability and enhance efficacy.
Distribution: How Drugs Move Within the Body
Distribution refers to the process by which a drug disperses throughout the body's tissues and fluids after absorption. This phase is crucial for determining the drug's efficacy and safety. Understanding distribution helps in predicting drug concentrations in various organs, thereby influencing both therapeutic outcomes and potential toxicities.
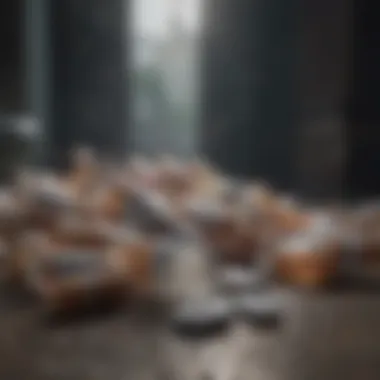
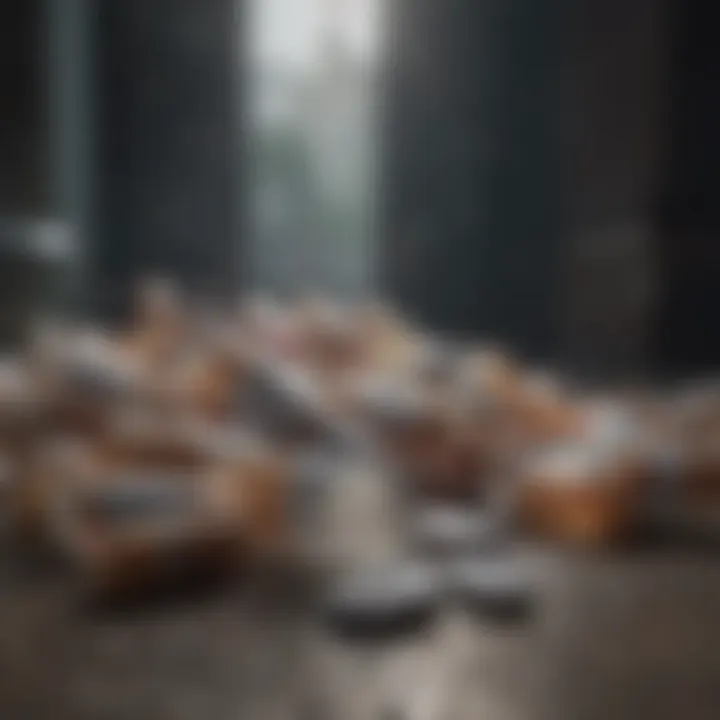
The distribution of drugs can be affected by several pharmacological factors. These might include the drug's lipophilicity, which impacts the ability to cross cell membranes, and its protein binding properties, which can influence the free drug available for action. Additionally, blood flow to tissues plays a vital role. Highly perfused organs, like the liver and kidneys, receive drugs faster than less perfused tissues. These considerations illustrate how distribution can vary significantly between drugs and patient populations.
Pharmacological Factors Affecting Distribution
There are key pharmacological factors that determine how drugs distribute throughout the body. These include:
- Molecular Size and Weight: Smaller molecules generally spread quicker as they can pass through vascular endothelium.
- Lipophilicity: High fat solubility allows drugs to enter fatty tissues more easily, which can lead to prolonged drug action.
- Protein Binding: Drugs that bind extensively to plasma proteins are not readily available for therapeutic effect.
- pH Partition Theory: The ionization of drugs at physiological pH can affect their distribution. Non-ionized drugs usually penetrate membranes better than ionized forms.
Understanding these factors is essential for optimizing the pharmacological use of each drug, ensuring its safety and effectiveness.
The Role of Blood-Brain Barrier
The blood-brain barrier (BBB) is a selective barrier that protects the brain from harmful substances while allowing essential nutrients to pass. It presents a unique challenge for drug delivery, as many therapeutic agents cannot cross this barrier effectively.
- Structure: Tight junctions between endothelial cells in brain capillaries limit paracellular permeability, making it hard for most substances to enter the brain tissue.
- Transport Mechanisms: Specialized transporters exist to facilitate the passage of certain compounds, like glucose and amino acids, but most drugs remain excluded.
This barrier has significant implications in treating neurological conditions. For instance, drugs developed for brain diseases need to possess specific characteristics, such as enhanced lipophilicity or molecular size adjustments to aid penetration.
"Effective drug delivery to the brain hinges on understanding and overcoming the blood-brain barrier."
Tissues as Reservoirs
Tissues can act as reservoirs for drugs, impacting their duration of action and efficacy. Storage in various tissues can prolong the effect of a drug as it is released slowly. For example, fat tissues can store lipophilic drugs, leading to cumulative effects over time.
- Liver: Often referred to as a primary storage and processing site due to its intricate vascular structure.
- Muscle: Can serve as a reservoir for hydrophilic drugs, influencing the duration of effect.
- Adipose Tissue: Significant for lipid-soluble drugs; patients with higher body fat may experience altered drug kinetics.
This distributional behavior emphasizes the need for tailored dosing regimens, particularly in obese or elderly patients who may have different pharmacokinetic profiles.
Understanding drug distribution is essential for optimizing pharmacotherapy and achieving desired health outcomes. Each of these elements plays a crucial role in shaping how a drug performs after it enters the body, guiding both clinical decisions and research directions.
Metabolism: The Body’s Chemical Processing
Metabolism refers to the collection of biochemical processes that transform drugs and other substances in the body. It plays a crucial role in pharmacology, as it determines how effectively a drug can exert its therapeutic effects. Proper understanding of metabolism informs dosing regimens, potential drug interactions, and overall drug safety.
Two main phases characterize metabolism: Phase I and Phase II. Phase I primarily involves chemical modifications of the drug, often through oxidation, reduction, or hydrolysis reactions. This phase is crucial in altering the drug's chemical structure, which may increase or decrease its biological activity. Phase II, on the other hand, typically involves conjugation reactions where the modified drug from Phase I combines with another substance to enhance water solubility. This makes it easier for the body to excrete the compound.
The efficacy of drug metabolism is influenced by various factors, including the drug's chemical properties, the individual's physiological condition, and genetic background. Understanding these phases helps in predicting the behavior of drugs in the body, thereby improving therapeutic outcomes.
Phases of Drug Metabolism
The two primary phases of drug metabolism are essential in transforming drugs into more readily excretable forms.
- Phase I Metabolism:
- Phase II Metabolism:
- Involves the introduction or exposure of functional groups on the drug molecule.
- Catalyzed primarily by the cytochrome P450 enzyme family.
- Resulting metabolites can still be active or inactive, affecting the drug's overall efficacy.
- Involves conjugation, linking the Phase I metabolites to another molecule.
- Common reactions include glucuronidation and sulfation, enhancing water solubility.
- Generally leads to the formation of inactive compounds, facilitating excretion through urine or bile.
Enzymatic Activity and Drug Interactions
Enzymatic activity is central to drug metabolism, mainly driven by cytochrome P450 enzymes. These enzymes vary between individuals due to genetic differences and environmental factors like diet or co-administered medications. This variability can lead to significant differences in drug metabolism rates among patients, which has direct implications for drug efficacy and safety.
Drug interactions often arise when one drug alters the metabolism of another. For example:
- Inhibition: One drug may inhibit the enzyme responsible for metabolizing another, leading to increased plasma concentrations, which enhances the risk of toxicity.
- Induction: Conversely, some drugs may enhance enzyme activity, possibly resulting in lower drug concentrations and decreased therapeutic effects.
This highlights the critical importance of understanding enzymatic pathways for clinical practice.
Genetic Variability in Metabolism
Genetic factors significantly influence individual metabolism. Variants in genes encoding drug-metabolizing enzymes can modulate metabolism rates. For instance, certain genetic polymorphisms in CYP2D6 can classify individuals as poor, intermediate, extensive, or ultra-rapid metabolizers. This classification impacts how individuals respond to medications, necessitating tailored therapeutic approaches.
- Key Points on Genetic Variability:
- Understanding genetic differences allows for personalization in treatment plans.
- Identifying polymorphisms can help avoid adverse drug reactions.
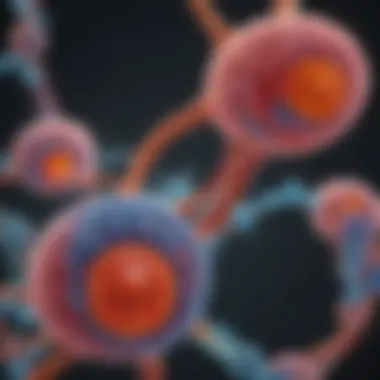
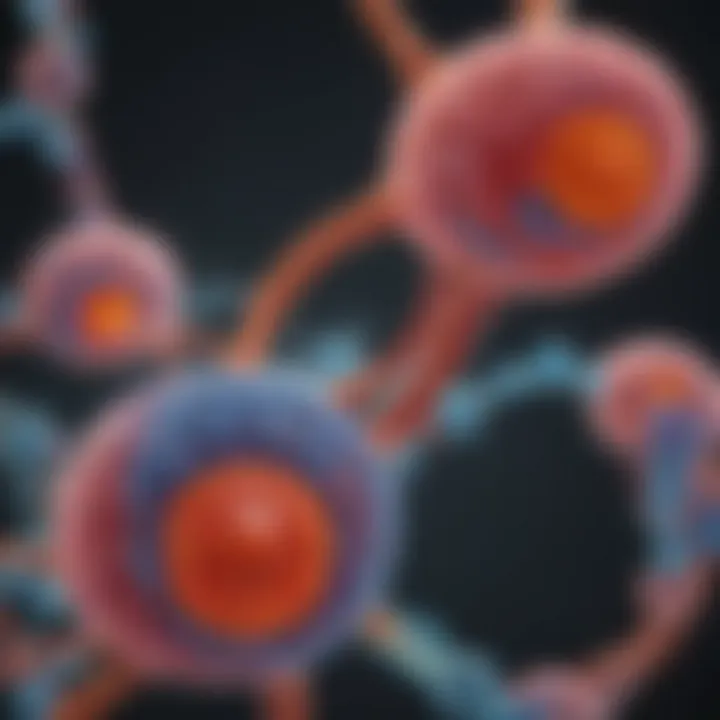
Excretion: The Final Phase of Drug Clearance
Excretion represents the last crucial step in the journey of a drug through the body. It involves the removal of waste products from the metabolic processing of compounds. This phase is essential not only for maintaining homeostasis but also for ensuring that drugs do not accumulate to toxic levels. Understanding the mechanisms and factors influencing excretion can greatly aid in the design of safer and more effective medications.
Routes of Excretion
The human body employs several pathways for the excretion of substances. The primary routes include:
- Renal Excretion: This is the most significant route, where the kidneys filter blood to remove waste products, leading to urine formation.
- Biliary Excretion: The liver packages drugs and their metabolites into bile, which is then excreted into the digestive tract. Some substances can also re-enter into the bloodstream through enterohepatic recycling.
- Respiratory Excretion: Gases, such as anesthetics, can be expelled through the lungs, indicating the body's ability to remove volatile substances.
- Sweat and Saliva: While less significant, these routes can also contribute to the elimination of some drugs. Sweating may even play a role in detoxification during intense physical activities.
Each route serves different purposes and is influenced by the chemical properties of the drug as well as individual physiological factors.
Factors Affecting Excretion Rates
Many variables can impact the efficiency of drug excretion. Key factors include:
- Physiological Conditions: Age, sex, and overall health can alter renal function, leading to variations in how effectively drugs are cleared.
- Hydration Levels: Adequate fluid intake affects urine production, influencing the elimination rate of drugs through the kidney.
- Drug Properties: pH, solubility, and molecular size significantly determine how a compound is processed and expelled. For instance, smaller and more water-soluble molecules are usually eliminated more easily.
- Interactions with Other Drugs: Some medications can inhibit or enhance the excretion of others, resulting in altered drug levels in the body, which may pose risks for toxicity or reduced efficacy.
The interplay of these factors must be carefully monitored to ensure optimal therapeutic outcomes while minimizing adverse effects.
"Understanding excretion is vital for clinicians and researchers. It influences drug dosing, safety, and ultimately, patient compliance."
To sum up, the excretion phase is not merely about waste removal; it is a complex and regulated process that plays a pivotal role in pharmacology. In-depth knowledge of this phase ensures that medications are used effectively and safely in clinical practice.
ADME in Drug Development
The significance of ADME in drug development cannot be overstated. ADME, which stands for Absorption, Distribution, Metabolism, and Excretion, encapsulates the pharmacokinetic processes that determine a drug's behavior in the human body. Understanding these components is vital for predicting how a substance will perform therapeutically.
Pharmaceutical scientists conduct rigorous ADME studies to evaluate how drugs are absorbed into the bloodstream after administration, how they are distributed to various tissues, how they are metabolized by the liver or other tissues, and how they are ultimately excreted through urine or feces. These studies provide insights that guide formulation methods and dosing regimens, allowing for more effective and safer medications.
In the stage of drug development, it is paramount to analyze the ADME properties of candidate compounds. This can indicate their potential efficacy, bioavailability, and therapeutic windows. Any shortcomings in ADME profiles may lead to modifications in the chemical structure, dosage forms, or delivery systems before reaching the market. The overarching goal is to enhance the therapeutic impact while minimizing adverse effects.
Another important point is the financial implications of thorough ADME evaluation. Efforts spent on ADME can save costs in the long run. By identifying problematic compounds early, researchers can prevent later-stage failures that could lead to significant financial losses. Given the increasing costs associated with drug development, incorporating ADME considerations offers a strategic advantage for pharmaceutical companies.
Importance of ADME Studies
ADME studies are crucial for various reasons. They provide essential data that influence the design and optimization of new drugs. Understanding absorption rates aids in determining the most effective administration routes. For instance, some drugs may function best when taken orally, while others might be more effective intravenously.
Additionally, insights into how well a drug distributes in various tissues help predict its action and side effects. Such studies assist researchers in making informed choices when it comes to dosing. The goal is always to find a balance between effectiveness and safety.
The assessment of metabolic pathways enables scientists to identify possible drug interactions that can alter efficacy or safety profiles. Variability in metabolism rates among individuals can impact therapeutic outcomes, underscoring the need for personalized approaches in medicine.
Regulatory Considerations
Regulatory agencies closely scrutinize ADME data as part of the drug approval process. Agencies like the U.S. Food and Drug Administration (FDA) have set clear guidelines for the reporting and evaluation of ADME characteristics in new drug applications.
ADME data must be robust and meet specified criteria to ensure that drugs are safe and effective for public use. Moreover, the integration of ADME data into the early phases of development can streamline the regulatory review process.
Furthermore, translational research is increasingly prioritized by regulatory bodies. This means that the relationship between laboratory findings and clinical outcomes is crucial. Providing strong ADME data strengthens the link between preclinical studies and eventual human use, which is a significant consideration in any regulatory review.
"ADME plays a pivotal role in drug development and regulatory approvals, impacting everything from formulation design to market access."
Through comprehensive ADME studies, pharmaceutical companies can better align their drugs with regulatory expectations. This proactive approach reduces the likelihood of extensive revisions or delays in the approval process, expediting the journey from discovery to patient care.
Challenges in ADME Research
The field of ADME research is critical for understanding how drugs behave in the human body. However, it faces several challenges that can impact the development of safe and effective medications. Addressing these challenges is essential for enhancing drug development processes and ensuring that new therapeutics are both effective and safe for human use.
One significant issue is the limitations of current models used to predict ADME properties. These models vary in accuracy and can lead to unreliable predictions, complicating the decision-making process for drug development. Insufficient data may also arise from a lack of representative biological samples. Moreover, current models often do not account for the complexity of human physiology, which can lead to discrepancies between predicted and actual drug behavior.
Another challenge revolves around ethical considerations in human studies. Conducting ADME studies on humans requires rigorous ethical oversight to ensure that participants' rights and well-being are protected. This limits the types of trials that can be performed, sometimes delaying crucial research.
Limitations of Current Models
The existing ADME models, while useful, have notable limitations. They generally fall into two categories: in vitro studies and in vivo studies. In vitro methods often rely on cell lines or tissues that may not fully represent the reactions occurring in the human body. These simplifications can yield misleading results, particularly in predicting metabolic pathways or interactions with other substances in a complex biological environment.
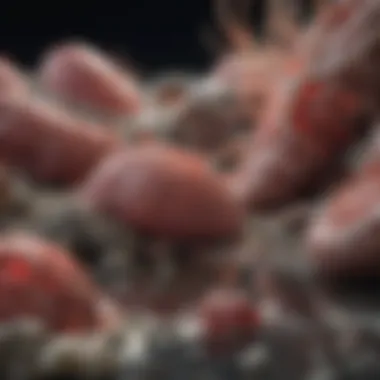
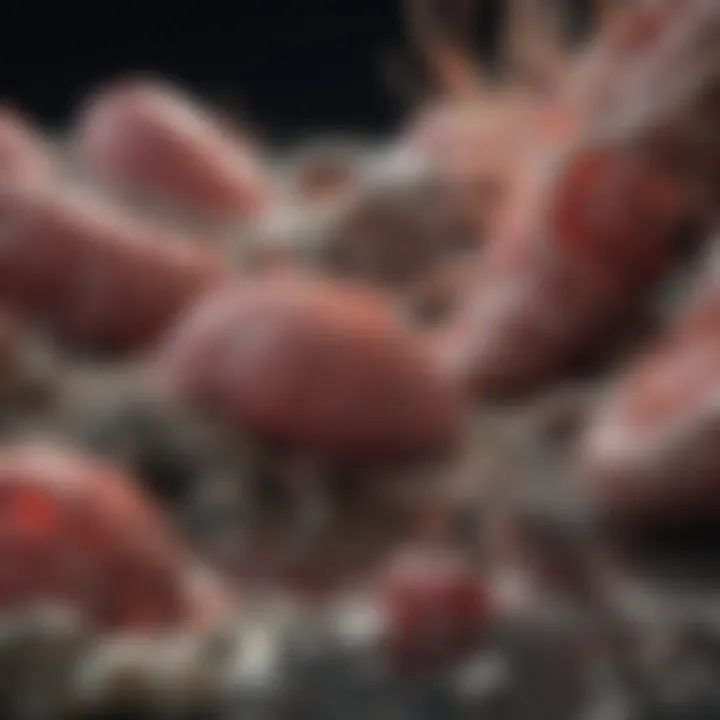
In vivo studies present their own issues, mainly related to ethical constraints and variability among human subjects. Factors such as genetics, age, and general health can influence how drugs are absorbed, distributed, metabolized, and excreted. Consequently, the results from animal studies may not always translate effectively to humans. The limitations in predictive power drive the necessity for improvements in modeling techniques, such as integrating more comprehensive physiological parameters.
"Current ADME predictions may lack accuracy and thus bring risks in drug development."
Ethical Considerations in Human Studies
Ethical considerations are at the forefront of human trials in ADME research. Researchers must navigate a complex landscape of regulations and ethical guidelines aimed at protecting participants. Obtaining informed consent is paramount, ensuring that participants understand the nature of the studies and any potential risks involved. This is particularly crucial in studies involving vulnerable populations or complex drug interactions.
The urgency to gather data on human pharmacokinetics often clashes with the ethical need for thorough oversight and safety measures. This situation can lead to slower progress in understanding how drugs perform in the human population. Balancing ethical considerations with the need for clinical data remains a substantial challenge in ADME research.
In summary, addressing the challenges in ADME research, particularly the limitations of models and ethical concerns in human studies, is vital for advancing drug development. It is through overcoming these hurdles that the industry can improve its efficiency and effectiveness in delivering safe and efficacious therapeutics.
Advancements in ADME Studies
Recent advancements in ADME studies signify a pivotal turning point in pharmacology. Understanding how drugs are absorbed, distributed, metabolized, and excreted is essential for developing effective therapies. These advancements lead to more accurate predictions of drug behavior within the body, optimizing dosage and minimizing adverse effects. Technology infinitely expands the possibilities of research, providing tools that can refine processes and outcomes in drug development. Ultimately, this can lead to better health outcomes for patients.
Technological Innovations
Innovations in technology have dramatically altered the landscape of ADME research. High-throughput screening methods allow scientists to examine thousands of compounds simultaneously, speeding up the discovery of viable drugs. Automated systems ensure more consistent results and reduce human error, making the data more reliable. Moreover, advanced imaging techniques, such as positron emission tomography (PET), enable real-time observation of drug behavior in living organisms. This direct visualization helps researchers to observe drug distribution and metabolism in ways that were not possible before.
Additionally, artificial intelligence and machine learning algorithms are increasingly utilized to predict ADME properties of new compounds. These algorithms analyze big data from previous studies, uncovering patterns that can inform future drug designs. They help in predicting pharmacokinetic properties before extensive lab work begins, saving valuable time and resources.
In Silico Modeling in ADME
In silico modeling plays a crucial role in enhancing the understanding of ADME characteristics. This computational approach allows researchers to simulate how a drug behaves in the body without immediate reliance on animal or human subjects. Models can forecast absorption rates, distribution volumes, or metabolic pathways based on chemical structure and other variables. These predictions help in identifying promising candidates earlier in the development process.
Such methodologies can also facilitate the assessment of genetic variability among individuals, allowing for tailored approaches in drug therapy. Tools like quantitative structure-activity relationship (QSAR) models evaluate potential biological activity based on chemical structure.
As ADME research continues to evolve, integrating these technological advancements ensures a more efficient and informed approach to developing safe and effective medications, enhancing overall patient care.
Future Directions of ADME Research
The exploration of ADME research continues to evolve. It is vital to acknowledge the critical role that ADME components play in pharmacology. As science progresses, so does the need to enhance our understanding of how these processes affect drug therapy and development. Focusing on future directions allows researchers and practitioners to adapt to emerging challenges in the field. By identifying promising areas, it can lead to innovations that improve drug efficacy and patient safety.
Personalized Medicine and ADME
Personalized medicine represents a paradigm shift in the healthcare landscape. In this context, ADME becomes even more significant. Understanding individual variations in absorption, distribution, metabolism, and excretion is key for tailoring treatments. Each patient's genetic makeup can influence how they process medications. For instance, variations in liver enzymes affect metabolism rates, which in turn impacts the drug concentration in the body. Therefore, personalized approaches must be integrated into ADME studies to refine dosage recommendations.
Some potential benefits of personalized medicine in relation to ADME include:
- Improved Efficacy: Customizing treatment plans enhances the likelihood that drugs will be effective.
- Reduced Adverse Effects: By understanding a patient's metabolism, clinicians can avoid prescribing drugs that may cause harm due to abnormal processing.
- Optimized Drug Development: Knowledge about patient variability can guide researchers in developing drugs that are more suitable for diverse populations.
Incorporating personalized medicine into ADME studies allows for a more holistic approach to patient therapy. This integration holds the promise of advancing drug development by focusing on the individual rather than a one-size-fits-all method.
Integration of Pharmacogenomics
Pharmacogenomics plays a crucial role in modern pharmacology. It examines how genes affect a person's response to drugs. Integration of pharmacogenomics with ADME research can lead to significant insights into drug efficacy and safety. This integration is essential for understanding why certain individuals experience better outcomes or more side effects than others.
The following considerations highlight the importance of merging pharmacogenomics with ADME:
- Targeted Drug Design: Knowledge of genetic variations can inform drug design tailored to metabolize specific genotypes effectively.
- Dosing Regimens: Pharmacogenomic data allows for more precise dosing recommendations, providing clarity on how different patients should receive their medications.
- Treatment Decision-Making: Clinicians can make informed treatment decisions based on a patient’s genetic profile, which can increase confidence in the effectiveness of prescribed medications.
"ADME processes combined with pharmacogenomic insights may redefine how practitioners approach medication management, paving the way for safer and more effective therapies."
The convergence of pharmacogenomics and ADME is revolutionary. It addresses the need for more refined strategies in drug therapy. Future research must focus on this integration to fully realize the potential benefits for patients.
Finale
The conclusion serves as a synthesis of the entire discussion on ADME, emphasizing the essential roles these processes play in pharmacology. Understanding ADME is crucial for evaluating how drugs interact with the human body, ultimately improving therapeutic outcomes and minimizing adverse effects. This section will reiterate key components, laying out their significance in the broader context of drug development and patient care.
Recap of Key Insights
The exploration of absorption, distribution, metabolism, and excretion uncovers fundamental aspects of pharmacokinetics. Each area's impact on drug efficacy can’t be overstated. It is vital to remember that:
- Absorption determines how much of a drug enters the bloodstream.
- Distribution affects how a drug is delivered to tissues and organs.
- Metabolism describes the biochemical alteration of the drug, influencing duration and intensity of action.
- Excretion ensures the removal of drug metabolites from the body, impacting clearance rates.
These insights highlight the delicate balance between the desired therapeutic effects and potential toxicities. Integrating this understanding into clinical practice enhances the safety and effectiveness of pharmacotherapy.
The Importance of Continued Research
Continued research in ADME is essential for several reasons. Advances in methodologies and technologies can yield better models for predicting drug behavior. Research can identify new targets for drug therapy, leading to the development of more effective drugs with fewer side effects. Furthermore, understanding the nuances of individual responses to medications is becoming increasingly important, particularly with the rise of personalized medicine.
"Research into ADME profiles not only enhances our grasp of pharmacokinetics but also guides therapeutic applications in diverse populations."