Understanding Battery Discharge Mechanisms and Effects
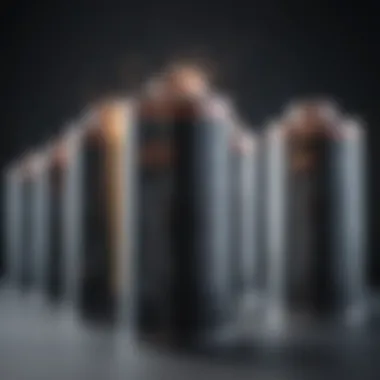
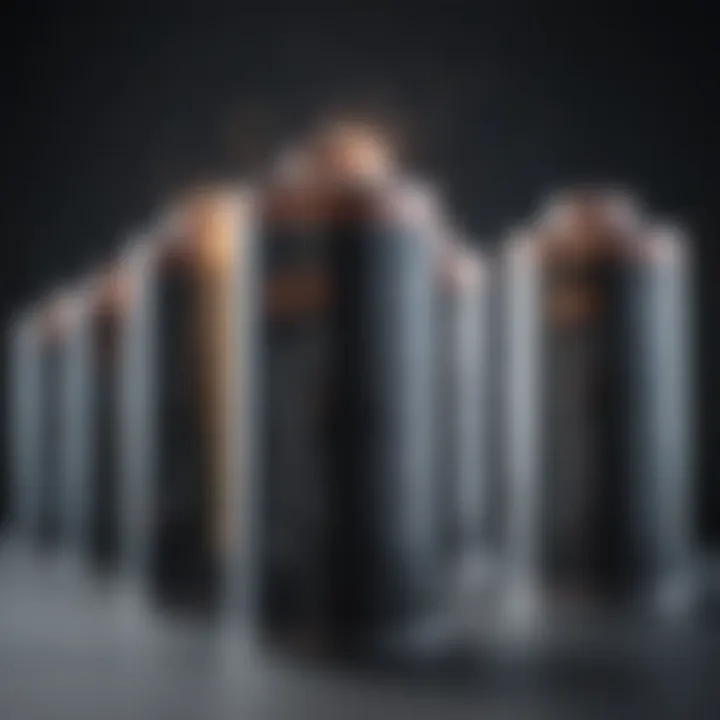
Intro
Battery discharge is a critical phenomenon that occurs in various devices, from smartphones to electric vehicles. Understanding how this process works can greatly inform both practical applications and theoretical research. Discharge is not just about how long a battery lasts; it involves numerous factors that influence efficiency, lifespan, and performance.
This piece will explore the fundamental principles behind battery discharge. It will provide detail on the mechanisms at play, effects that discharge levels have on battery performance, and ways in which management strategies can be optimized for better sustainability. The discussion will also touch on different battery chemistries, highlighting their unique characteristics and real-world implications. By unpacking these essentials, readers can gain a deeper appreciation of battery technology and its relevance in today's digital age.
Research Methodology
Description of Research Design and Approach
The exploration of battery discharge mechanisms requires a robust research approach. This article synthesizes existing literature, case studies, and empirical research to provide a comprehensive overview. The goal is to harmonize theoretical frameworks with practical data, ultimately empowering readers to draw informed conclusions about battery performance.
Materials and Methods Used in the Study
A variety of materials were reviewed to support this research. These include scientific journals, technical manuals, and industry reports focusing on battery technologies. Specific attention was paid to published experiments that evaluated discharge characteristics across different battery chemistries, such as lithium-ion, nickel-cadmium, and lead-acid. Incorporating data from a range of sources allows for a more nuanced understanding of how environmental factors, temperature variations, and discharge depth influence performance.
Discussion and Interpretation
Interpretation of Results in the Context of Existing Literature
Research reveals that factors such as temperature, discharge depth, and battery composition drastically affect performance and lifespan. For instance, a study found that lithium-ion batteries, when subjected to extreme temperatures, demonstrated decreased cycle life compared to those maintained within optimal temperature ranges. This aligns with findings from previous research, emphasizing the importance of temperature management in enhancing battery efficacy.
Implications for Future Research or Practical Applications
Future research could delve deeper into developing new battery materials that resist performance degradation. There is also a pressing need for practical applications that enhance user behavior towards battery management. For example, integrating smart technologies that monitor battery health in real-time could mitigate discharge-related issues for consumers and industries alike.
The ongoing evolution of battery technology necessitates a careful consideration of discharge mechanisms to fully leverage their potential.
As this article unfolds, it will guide the reader through the complexities of battery discharge, ensuring a comprehensive understanding of its mechanisms and management.
Intro to Battery Discharge
Battery discharge is a fundamental process that underpins the functionality of numerous electronic devices, from smartphones to electric vehicles. As reliance on battery power increases, comprehending the mechanisms and implications of battery discharge becomes essential. Understanding this topic is crucial for both the design of more effective energy systems and the management of existing battery technologies.
This section aims to establish a solid foundation in the principles of battery discharge. It will define the concept clearly and highlight its significance across various energy systems.
Definition of Battery Discharge
Battery discharge refers to the process in which stored chemical energy in a battery is converted into electrical energy. This occurs when a battery is connected to a load, allowing electrons to flow from the anode to the cathode through an external circuit. In essence, discharge signifies the battery providing energy to power devices. As the battery discharges, the chemical reactions that generate electrical energy commence, and specific materials within the battery undergo changes.
Understanding the definition of battery discharge is vital because it informs how energy is consumed and the efficiency of the process. Notably, different battery chemistries—such as lithium-ion, lead-acid, and nickel-cadmium—exhibit distinct discharge characteristics.
Importance in Energy Systems
The importance of battery discharge cannot be overstated, especially in the context of today’s energy systems. Batteries play a pivotal role in energy storage, serving as buffering mechanisms that allow for the balancing of supply and demand. Their performance directly influences the effectiveness of renewable energy sources, such as solar and wind, which are inherently intermittent.
Several key considerations arise regarding the significance of battery discharge in energy systems:
- Energy Management: Proper management of battery discharge affects the overall energy efficiency. An understanding of discharge mechanisms is essential for optimizing energy utilization.
- Capacity Evaluation: Knowing how to assess discharge rates and capacity can enhance one’s ability to make informed decisions about battery use in applications.
- Longevity and Sustainability: Effective management to mitigate degradation during discharge cycles extends battery life, contributing to sustainability efforts in technology infrastructures.
Understanding battery discharge is not merely an academic pursuit, but a critical necessity for advancing our energy systems and ensuring efficient technology use.
As we delve into the subsequent sections, we will explore the fundamental principles of discharge, the various battery types and their characteristics, and the factors that affect battery performance. This comprehensive exploration illustrates the relevance of battery discharge in today’s technology-driven society.
Fundamental Principles of Battery Discharge
Understanding the fundamental principles of battery discharge is crucial in grasping how batteries work in various applications. This section outlines the thermodynamics and electrochemical reactions involved in discharge processes. By delving into these core concepts, we can appreciate the complexities and subtleties of battery performance, tailoring our approach to different situations and requirements.
Thermodynamics of Discharge
The thermodynamics of battery discharge refers to the energy changes that occur as a battery releases power. When a battery discharges, chemical energy is converted into electrical energy, following the first law of thermodynamics. This principle states that energy cannot be created or destroyed, only transformed. Therefore, the energy output during discharge must equal the energy input during charging, minus any losses.
Key factors influencing thermodynamic efficiency include:
- Internal Resistance: This is the resistance within a battery that causes energy loss in the form of heat during discharge. A higher internal resistance leads to lower efficiency and may result in overheating.
- Ambient Temperature: Temperature significantly affects the thermodynamic properties of battery materials. Low temperatures can increase internal resistance, while high temperatures may accelerate degradation processes.
- State of Charge (SoC): The energy level in a battery impacts its performance and efficiency. As a battery discharges, its SoC decreases, influencing voltage and current output capabilities.
In optimal conditions, batteries should work close to their theoretical capacity. However, losses always occur, thus real-world applications necessitate factoring in these thermodynamic considerations to enhance overall performance.
Electrochemical Reactions
Electrochemical reactions are at the heart of battery functionality. During discharge, the reactions between the electrodes and the electrolyte produce electrons, which flow through the external circuit, powering devices.
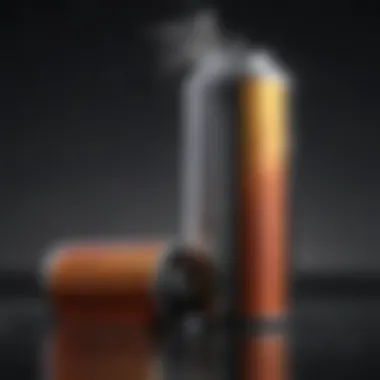
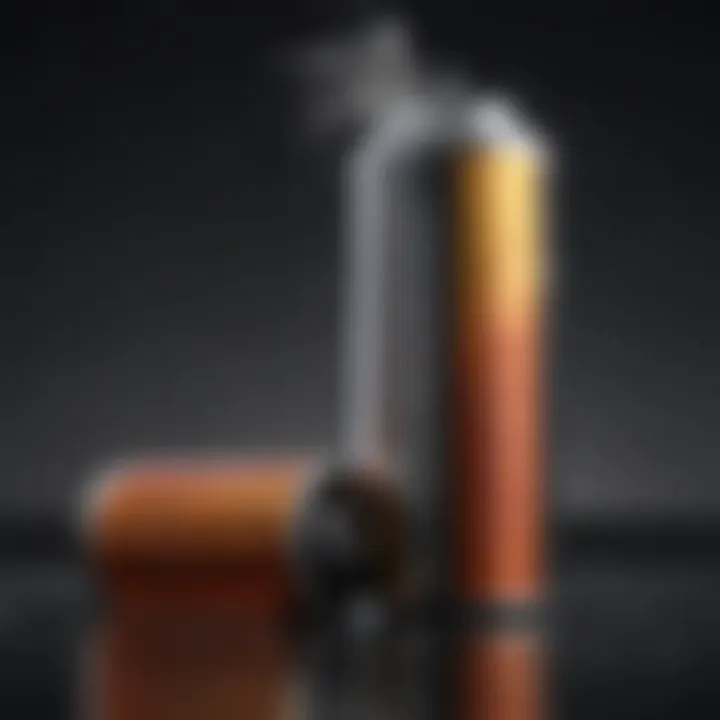
Two main reactions occur in most batteries:
- Oxidation Reaction at the Anode: Electrons are released from the anode material, typically a form of a metal or a metal oxide. This process generates positive ions that migrate toward the cathode.
- Reduction Reaction at the Cathode: At the other end, the cathode material gains these electrons. The reduction process takes place as the positive ions from the electrolyte combine with electrons to form neutral atoms or molecules, recharging the cathode.
The efficiency of these reactions dictates the performance of the battery. Factors affecting electrochemical reactions include:
- Electrode Material: Different materials exhibit varying levels of conductivity and reactivity. The choice of electrode significantly influences discharge rates and capacity.
- Electrolyte Composition: The electrolyte's properties, including its ionic conductivity and viscosity, impact the speed of ion transfer between electrodes, affecting overall performance.
- Surface Area: Increasing the active surface area of electrodes enhances reaction rates, allowing for higher discharge rates.
Types of Batteries and Their Discharge Characteristics
In understanding battery discharge, it is vital to explore the variety of battery types and their unique discharge characteristics. Each battery chemistry exhibits distinct behaviors under discharge conditions. This knowledge is essential for selecting the suitable battery for specific applications, impacting performance, longevity, and efficiency. Recognizing these differences allows engineers and consumers alike to make informed choices, directly influencing the effectiveness of energy utilization.
Lead-Acid Batteries
Lead-acid batteries are among the oldest types of rechargeable batteries. They are particularly favored in applications requiring high surge currents, such as in vehicles. A significant characteristic is their robust discharge capability, which enables them to deliver a large amount of current quickly. However, they have limitations regarding depth of discharge; deeper discharges can lead to reduced cycle life.
The chemical reaction in lead-acid batteries involves the conversion of lead dioxide and sponge lead into lead sulfate. This process releases energy efficiently when discharging. Typical applications include automobile starting, uninterruptible power supplies, and renewable energy storage, due to their relatively low cost and ability to supply high currents on demand.
Advantages of Lead-Acid Batteries:
- Cost-effective compared to many modern batteries
- Proven technology with widespread availability
- Capable of high discharge rates
Considerations:
- Heavier and bulkier than newer alternatives
- Sensitive to deep discharge scenarios
Lithium-Ion Batteries
Lithium-ion batteries have revolutionized the energy storage landscape due to their high energy density and efficiency. These batteries are lighter and have a higher voltage per cell compared to lead-acid. The mechanisms of lithium-ion discharge are based on the movement of lithium ions between the anode and cathode during charging and discharging cycles.
The significant benefit is the ability to sustain higher depths of discharge without significantly impairing battery life. This feature makes lithium-ion batteries suitable for portable electronic devices and electric vehicles, where both weight and performance are critical. They also exhibit consistent performance across a wide temperature range, contributing to their utility in various environments.
Advantages of Lithium-Ion Batteries:
- High energy density and longer lifespan
- Lightweight and compact size
- Minimal memory effect compared to nickel-cadmium batteries
Considerations:
- Higher initial investment compared to lead-acid batteries
- Requires sophisticated management systems to ensure safety and performance
Nickel-Cadmium Batteries
Nickel-cadmium batteries, or NiCd batteries, are another reliable option due to their resilience and ability to function in extreme conditions. They are well-known for their capability to deliver high discharge rates and are commonly used in applications like power tools and emergency lighting.
However, one of the concerns is the memory effect, which can occur if the battery is repeatedly recharged before being fully discharged. This effect reduces the effective capacity of the battery over time. Despite this drawback, NiCd batteries provide dependable performance and durability, particularly in settings where reliability and robustness are paramount.
Advantages of Nickel-Cadmium Batteries:
- Excellent cycle life and durability
- Can operate in extreme temperatures
- High discharge capabilities useful for power-intensive applications
Considerations:
- Environmental and health concerns related to cadmium
- Memory effect needs to be managed to maintain capacity
In summary, understanding the characteristics of these different battery types is crucial for optimizing their use in practical applications. Each battery chemistry has its strengths and limitations, underlining the importance of discerning suitable choices for specific energy storage needs.
Factors Affecting Battery Discharge
Understanding the factors that influence battery discharge is essential for optimizing performance and extending lifespan. This section dives into three critical elements: depth of discharge, temperature effects, and load conditions. Each of these factors plays a significant role in how batteries operate and how effectively they can deliver energy for their intended applications.
Depth of Discharge
Depth of discharge (DoD) refers to the extent to which a battery has been discharged relative to its total capacity. For instance, a DoD of 50% means that half of the battery’s total energy has been used. This factor is crucial for several reasons. Firstly, different battery chemistries exhibit varying tolerances to DoD, which can affect overall performance and durability. For lithium-ion batteries, shallow discharges often lead to longer battery life, while lead-acid batteries can handle deeper discharges without immediate failure.
The relationship between DoD and battery cycle life is especially significant. As the depth increases, the number of cycles a battery can endure before degradation diminishes. Therefore, managing how deeply batteries discharge can enhance efficiency and decrease costs in applications ranging from electric vehicles to renewable energy systems.
Temperature Effects
Temperature plays a vital role in battery discharge efficiency and safety. Higher temperatures can increase the rate of electrochemical reactions, which can lead to improved short-term performance. However, prolonged exposure can cause overheating, risking thermal runaway in lithium-ion systems. In contrast, lower temperatures can impede chemical reactions, leading to reduced capacity and power output.
This variance underlines the necessity of maintaining optimal operating temperatures. For instance, lithium-ion batteries typically perform best around 20 to 25 degrees Celsius. To mitigate temperature-related issues, effective cooling systems or insulation strategies need implementation, especially in high-demand environments.
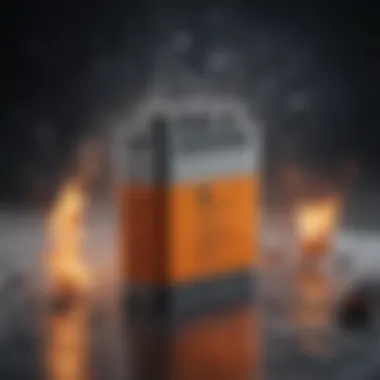
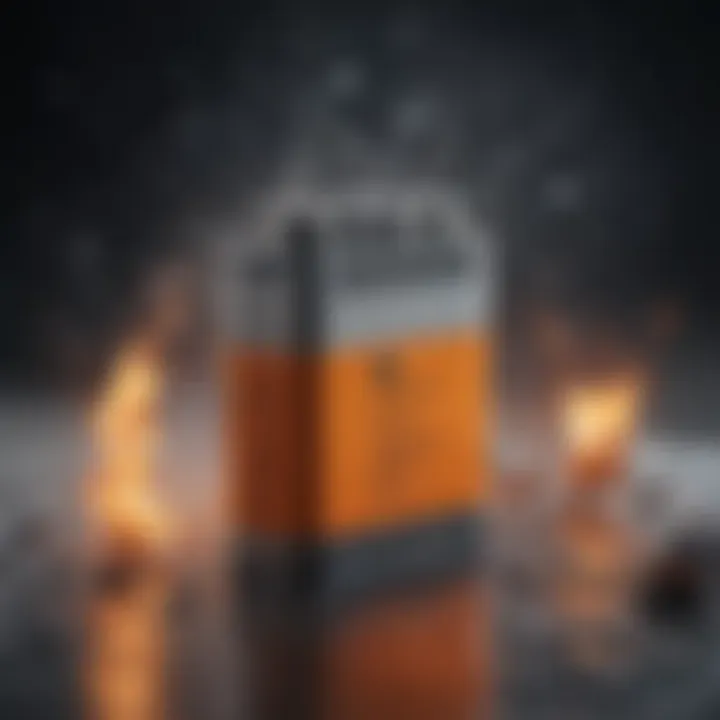
Maintaining the optimal temperature range significantly influences battery life and performance efficiency.
Load Conditions
Load conditions refer to the demand placed on a battery during discharge. The load can vary based on the application, be it a stationary energy storage system or an electric vehicle. Higher load conditions tend to increase discharge rates, impacting both efficiency and longevity. Essentially, higher current draws lead to significant stress on the battery, which can result in increased heat generation and decreased usable capacity over time.
Additionally, varying loads can affect the voltage output, ultimately influencing device performance. Understanding the relationship between load and discharge characteristics is paramount in designing systems that rely on batteries for power.
In summary, attention to depth of discharge, temperature effects, and load conditions can dramatically improve battery management strategies, enhance safety, and prolong battery lifespan. Such insights make it possible to navigate the complexities of energy storage technologies effectively.
Discharge Rate and Capacity
The discharge rate and capacity of a battery are paramount in understanding its performance and longevity. These factors directly influence how batteries operate under various conditions and are pivotal for optimizing their use in different applications. By comprehending the discharge rate and capacity, users can refine management strategies, improve efficiency, and ultimately extend the life of the battery.
Understanding C-Rates
C-rates are a crucial concept when discussing battery discharge rates. They represent the speed at which a battery is charged or discharged relative to its capacity. For example, a battery rated at 1C can discharge its entire capacity in one hour. Conversely, a 2C rating means the battery can discharge its full capacity in half an hour. Understanding these rates helps in determining the appropriate use of batteries based on performance needs.
Here are some essential points regarding C-rates:
- Capacity Measurement: C-rates allow users to quantify battery performance in terms of current output and duration of action.
- Impact on Lifespan: Higher C-rates often correlate with increased stress on the battery, potentially leading to quicker degradation over time.
- Application Specificity: Different applications have varying C-rate requirements. A high-performance electric vehicle may need a higher discharge rate compared to a simple battery-operated light.
As C-rate increases, it often results in faster energy delivery and quicker power availability but can jeopardize the integrity of the battery if not managed correctly.
Influence of Capacity on Performance
Capacity is a measure of how much energy a battery can store and deliver. It is generally expressed in ampere-hours (Ah). Understanding capacity is critical for predicting how long a battery will last under specific loads. When determining battery performance, several factors are influenced by capacity:
- Energy Availability: A larger capacity means the battery can sustain longer usage before needing a recharge. This is vital for applications requiring extended operational time.
- Load Management: When load conditions fluctuate, a battery's capacity can restrict or extend operational time. Equipment that demands high energy over short durations will deplete a lower capacity battery more quickly than a higher capacity counterpart.
- Temperature Sensitivity: Battery capacity can also vary with temperature. For example, at low temperatures, a battery's effective capacity may decrease, lowering its overall performance.
"Optimal battery performance hinges not only on capacity but also how that capacity resonates with the discharge rate, determining the usability under different conditions."
Real-World Implications of Battery Discharge
Battery discharge is not merely a technical concept. It has profound real-world implications that affect countless technologies and everyday devices. Understanding these implications is critical for students, researchers, and professionals. This section deciphers several significant aspects of battery discharge in practical terms.
Battery Degradation Over Time
One of the most important aspects of battery discharge is how it leads to degradation over time. Batteries are finite resources. Each cycle of charging and discharging affects their chemical composition and functionality. For instance, Lithium-ion batteries, which are widely used, experience a gradual decline in capacity with every partial cycle. This phenomenon is termed cyclic aging.
Factors that influence this degradation include:
- Depth of Discharge (DoD): A higher DoD can lead to faster degradation.
- Temperature: Elevated temperatures can accelerate chemical reactions that harm battery components.
Over time, the effective capacity of a battery reduces, affecting performance in end-use applications. Users often face a dilemma between performance and longevity. In critical applications, like electric vehicles and renewable energy systems, a careful balance must be maintained. Mitigation strategies, such as optimizing charging practices, can prolong battery life. Ultimately, understanding degradation helps in developing better protocols for battery usage.
Impacts on Device Performance
The relationship between battery discharge and device performance is clear. As battery health declines, so does the performance of the associated device. Users might notice that their smartphones do not last as long on a single charge as they once did. Additionally, in applications such as drones or medical devices, battery performance can mean the difference between functionality and failure.
Some specific impacts include:
- Reduced Operating Time: Devices experience shorter operational periods as batteries lose capacity.
- Voltage Sag: A non-ideal discharge can result in lower voltages, affecting device performance.
- Increased Load Times: Devices may take longer to perform tasks if batteries cannot provide sufficient current.
"A battery's ability to deliver power at the required levels directly equates to the efficiency of the device it powers."
When a battery is unable to sustain necessary discharge rates, devices may enter power-saving modes or shut down unexpectedly. This behavior is particularly detrimental in mission-critical applications. Thus, understanding reliable battery discharge can enhance device design and application reliability.
Strategies for Battery Management
Battery management is essential for optimizing the performance and lifespan of batteries. As batteries become integral to our lives, strategies for their management take on increased significance. The purpose of effective battery management is to ensure safety, efficiency, and longevity of battery systems. Some key benefits include enhanced performance, reduction in degradation, and cost-effectiveness over time.
Moreover, contemporary devices require batteries to be managed intelligently. Inadequate management can lead to several problems, including thermal runaway, irreversible chemical reaction, and reduced usable capacity. A proactive approach to battery management must consider the nuances of battery chemistry, usage patterns, and environmental conditions.
Managing battery health requires a comprehensive understanding of its discharge and charging cycles, regular monitoring, and timely intervention.
Optimal Charging Practices
Charging practices play a crucial role in battery management. Proper methods not only extend battery life but also ensure safety during operation. Utilizing chargers designed for specific battery types is one of the fundamental practices. For example, lithium-ion batteries should not be overcharged, as this can lead to overheating and potential failures.
Furthermore, it is recommended to charge batteries at moderate rates. High charging rates generate excess heat, leading to degradation of the materials within the battery. In contrast, low charging rates are gentler on the battery, resulting in less stress and prolonged life. Many lithium-ion chargers now come with smart technology that adjusts the charge rate based on the battery state, which helps in optimizing the process.
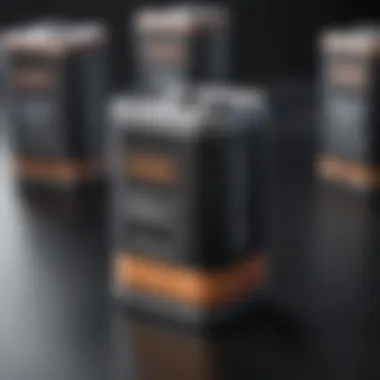
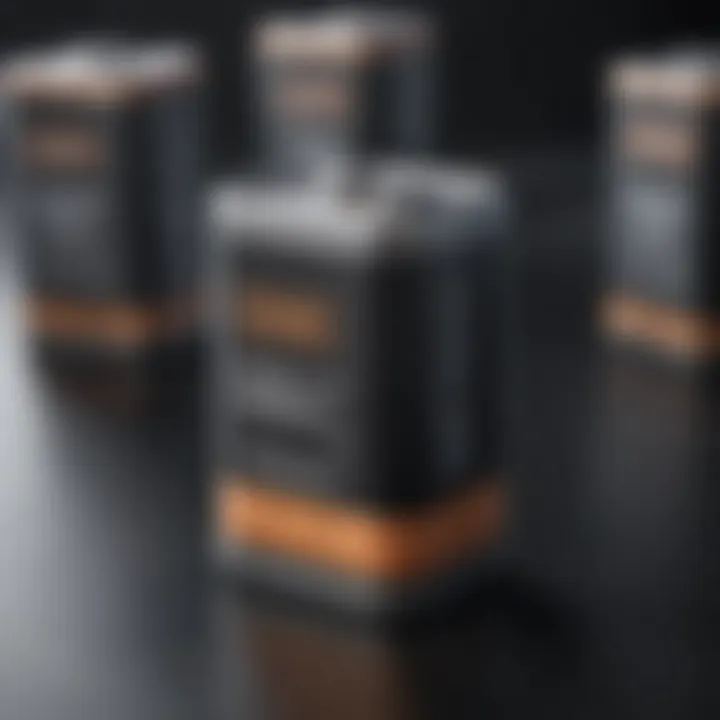
Additionally, prioritizing partial charges rather than deep discharges can vastly improve battery health. Keeping the battery between 20% and 80% charged is often ideal. This prevents the voltage from dropping too low, which would significantly stress the battery’s components.
Temperature Control Techniques
Temperature control is a critical factor in the management of batteries. Extreme temperatures can adversely impact both performance and safety. High temperatures can accelerate the chemical reactions inside batteries, leading to faster degradation. Conversely, cold temperatures can impede reactions, causing inefficient power delivery.
Implementing temperature control mechanisms can mitigate these effects. This may involve:
- Utilizing thermal management systems that maintain optimal operating temperatures.
- Designing battery enclosures that offer insulation, protecting against external temperature fluctuations.
- Monitoring the thermal performance of batteries regularly to anticipate any issues.
Lastly, battery management systems often incorporate temperature sensors to provide real-time monitoring. By analyzing temperature data, operators can implement corrective measures if the battery approaches critical temperature thresholds.
Innovations in Battery Technology
Innovations in battery technology play a significant role in shaping energy storage solutions for the future. As technology advances and the demand for efficient and sustainable power sources increases, innovation becomes crucial. These advancements aim not only to improve performance and efficiency but also to address environmental concerns. The focus is on creating batteries that have longer lifespans, greater energy densities, and reduced environmental impacts.
Advancements in Lithium-Based Batteries
Lithium-based batteries are among the most widely used batteries due to their high energy density and lightweight properties. One of the key advancements in this area is the development of enhanced electrode materials. For instance, the incorporation of silicon into anodes significantly increases their capacity compared to conventional graphite. Additionally, researchers are exploring novel electrolyte formulations to improve safety and performance. This is vital as overheating and fires are major safety concerns with lithium-ion batteries. Another promising advancement is the solid-state battery, which replaces the liquid electrolyte with a solid. This shift not only boosts energy density but also enhances safety by reducing flammability.
These advancements improve usability in electric vehicles and portable electronics, making them more practical for everyday applications. Moreover, these developments address the critical shortage of raw materials like lithium and cobalt, promoting better energy security and less reliance on mining. Researchers continue to explore ways to recycle these materials effectively.
Exploration of Solid State Batteries
Solid-state batteries represent a paradigm shift in battery technology. Unlike traditional lithium-ion batteries that use liquid electrolytes, solid-state batteries employ solid electrolytes. This transition can lead to safer and more efficient batteries. The solid-state design reduces risks associated with electrolyte leakage and flammability that are present in liquid-based systems.
The benefits of solid-state batteries extend to energy density as well. They can potentially store more energy within a smaller volume compared to their predecessors, making them suitable for a variety of applications, ranging from electric vehicles to large-scale grid storage. However, challenges remain in manufacturing processes and scalability. Finding materials that are both conductive and abundant is critical.
Researchers are focusing on various materials, such as sulfide and oxide electrolytes, to overcome these challenges. The promise of solid-state batteries underscores a significant step toward more sustainable energy solutions. As these technologies continue to evolve, they offer a glimpse into a more energy-efficient future.
The evolution of battery technology, especially lithium-based and solid-state batteries, is essential for the transition to sustainable energy solutions. Improved performance, safety, and recyclability will shape the future of energy storage.
In summary, innovations in battery technology are foundational for addressing the escalating energy crisis and environmental concerns. Continuous research into advancements in lithium-based and solid-state batteries highlights a proactive approach in the scientific community to meet global energy needs.
Environmental and Societal Considerations
Understanding how battery discharge impacts our environment and society is crucial in today's world. As reliance on batteries grows in various applications, so does the responsibility to address the ecological footprint associated with their production and disposal. This section highlights the importance of considering sustainability and recycling efforts to mitigate adverse effects on our planet and social systems.
Sustainability of Battery Production
Battery production involves resource extraction, chemical processes, and energy consumption, all of which can contribute to environmental degradation. Raw materials, such as lithium, cobalt, and nickel, are often mined in regions where practices may not be environmentally friendly. For instance, lithium extraction from brine can impact local water supplies, and cobalt mining has raised significant ethical concerns over labor practices.
To enhance sustainability, manufacturers are exploring methods to reduce energy consumption during battery production. This includes utilizing renewable energy sources and developing more efficient recycling methods. Additionally, research is underway to create batteries with less reliance on scarce materials. For example, sodium-ion batteries are being studied as a potential substitute, as sodium is more abundant and widely available.
The shift towards sustainable practices offers several benefits. It can lead to a decrease in the environmental impact associated with raw material extraction and improve social conditions in mining regions through better labor practices. Furthermore, the move towards greener production methods may enhance the public perception of battery technologies, which is crucial for wide-scale adoption.
Recycling Implications
Battery recycling is an essential component of responsible battery use and management. The recycling process not only reduces the need for new raw materials but also lessens the environmental burden caused by discarded batteries. As batteries are thrown away, they can release harmful substances into soil and water, presenting a significant environmental risk.
Recycling technologies have advanced, enabling the recovery of valuable materials. For instance, lithium, cobalt, and nickel can be extracted from used batteries and repurposed for new units.
Some key points to consider about battery recycling include:
- Economic Value: Recycling programs can generate significant economic value by recapturing materials that are otherwise lost.
- Environmental Benefits: By reducing waste, recycling minimizes pollution associated with landfilling hazardous materials.
- Community Engagement: Encouraging local recycling initiatives raises awareness and promotes responsible battery disposal among consumers.
"Effective recycling not only preserves resources but also fosters a circular economy, reducing the lifecycle impact of batteries."
As society moves forward, the environmental and societal considerations surrounding batteries cannot be overlooked. A commitment to sustainability in production alongside innovative recycling efforts will shape the future of battery technology. It is essential to foster discussions and actions that promote responsible practices, ensuring that advancements contribute positively to our world.
Future Directions in Battery Discharge Research
Battery discharge is a critical factor in the performance and longevity of power storage technologies. As we delve deeper into Future Directions in Battery Discharge Research, it becomes evident that exploring new avenues can lead to significant advancements in energy efficiency and sustainability. This area of research is becoming increasingly important due to escalating energy demands and the shift towards renewable energy sources.
Emerging Technologies
Emerging technologies represent a frontier where battery performance can be significantly enhanced. New materials and designs are being investigated to improve charge capacity, discharge rates, and overall lifespan. One key area of focus is the development of solid-state batteries. Unlike conventional lithium-ion batteries, which use liquid electrolytes, solid-state batteries use solid electrolytes. This change can result in higher energy densities and improved safety. Researchers are exploring materials like ceramics and polymers that could make solid-state batteries a practical reality.
Other innovations include lithium-sulfur and lithium-air batteries, which promise greater energy storage efficiency. These technologies aim to replace or complement the current lithium-ion technology, offering higher capacities at lower costs. Each of these emerging technologies can reshape the landscape of energy storage, making batteries not only more efficient but also environmentally friendly.
Impact of AI on Battery Management
The application of artificial intelligence (AI) in battery management systems is another promising direction in research. AI can analyze large datasets from battery usage in real-time, allowing for smart management of energy resources. With predictive analytics, AI can optimize charging and discharging cycles. This reduces wear on batteries and extends their operational life.
Additionally, AI can help identify patterns in battery performance under various conditions. Such insights are critical for users who need to maintain optimal performance, particularly in electric vehicles or energy storage systems for renewable energy sources.
Incorporating AI into battery management systems also enhances safety. Early detection of issues such as overheating or unusual discharge rates can prevent catastrophic failures.
"The integration of AI into battery systems not only improves their life span but also enhances efficiency and safety for extensive usage scenarios."