Understanding Chromatography: Principles and Applications
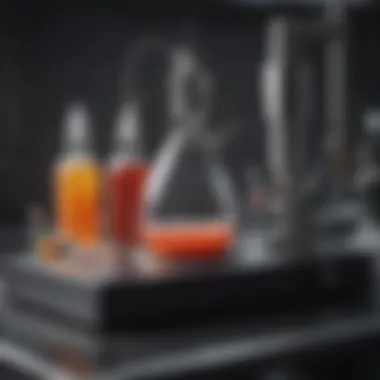
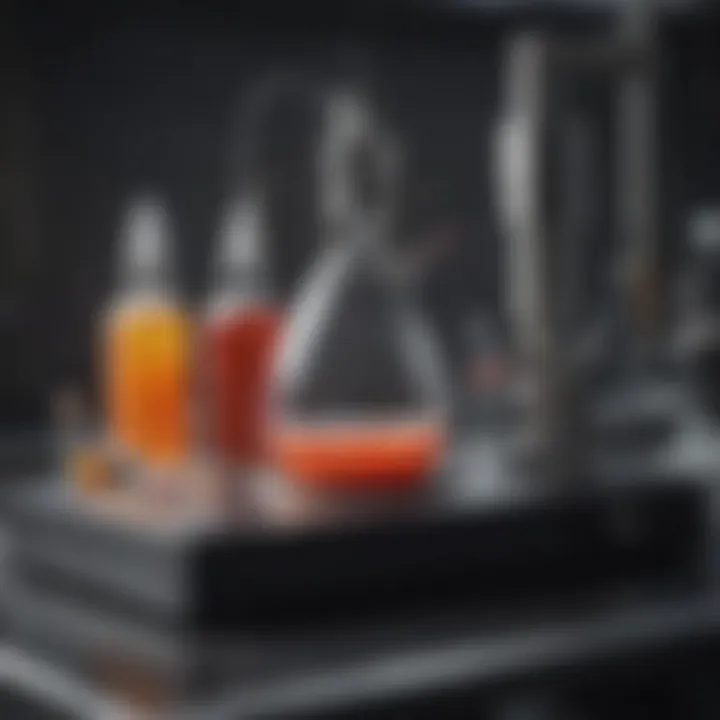
Intro
Chromatography stands as a pivotal technique in analytical chemistry, playing a central role across various scientific disciplines including biology and materials science. Its primary function revolves around the separation of complex mixtures into simpler components, which can subsequently be analyzed for qualitative and quantitative purposes. This article aims to unpack the intricate principles of chromatography, explore its diverse types, and illustrate its real-world applications.
The relevance of chromatography goes beyond mere academic interest. As industries focus on quality control and environmental monitoring, understanding this analytical technique becomes increasingly important. Knowledge of chromatography is essential for students, researchers, and professionals aiming to deepen their expertise in these fields.
In this exploration, we will cover the foundational aspects of chromatography, details of various methods, and the significance of this technique in contemporary research and industry settings.
Research Methodology
Description of research design and approach
The study of chromatography involves a systematic approach that emphasizes both theoretical and practical dimensions. Research in this area often begins with a thorough literature review to understand existing methods, applications, and theories. This step ensures a well-rounded comprehension of the subject.
Researchers typically employ both qualitative and quantitative analysis. Qualitative analysis helps in identifying the components present in a mixture, while quantitative analysis determines the concentration of these substances. This dual approach provides a comprehensive insight into chromatography's effectiveness as an analytical tool.
Materials and methods used in the study
In conducting chromatography research, several materials and methods are essential:
- Stationary Phase: The material through which the sample mixture travels, typically a solid or a liquid that is immobilized on a solid.
- Mobile Phase: The solvent used to carry the sample mixture. This can be a liquid or gas, depending on the type of chromatography employed.
- Detector: Devices like UV-Vis spectrophotometers or mass spectrometers used to identify and quantify the separated components.
Common methods include:
- High-Performance Liquid Chromatography (HPLC)
- Gas Chromatography (GC)
- Thin-Layer Chromatography (TLC)
These techniques employ different mechanisms of separation, such as partition or adsorption, influencing their applications and results.
Discussion and Interpretation
Interpretation of results in the context of existing literature
Results obtained through chromatography often align with existing data presented in previous studies. For instance, HPLC routinely demonstrates its efficiency in separating complex mixtures in pharmaceutical formulations. Studies have shown that it enhances the resolution of components, thereby facilitating better identification and quantification.
A comparative analysis with similar methods, like GC, highlights the specific advantages of each technique under various conditions. Understanding these differences can aid researchers in selecting the appropriate method for specific applications.
Implications for future research or practical applications
As science continues to evolve, so does the role of chromatography. Future research may delve into improving existing techniques, reducing analysis time, or enhancing sensitivity. Developments in miniaturization and automation could make chromatography more accessible and efficient.
Moreover, the ongoing emphasis on environmental monitoring underlines the necessity for robust analytical techniques. Chromatography's capacity to detect trace contaminants can support sustainability efforts across multiple industries.
"Chromatography is more than just a technique. It is a bridge between complex mixtures and clarity, helping us navigate the intricacies of chemical compositions."
In summary, understanding chromatography's principles and applications is crucial for both academic and industrial advancements. This technique offers profound insights into the composition of materials critical for quality control, research, and environmental safety.
Prelims to Chromatography
Chromatography serves as a pivotal method within analytical science, enabling the systematic separation and analysis of complex mixtures. This section aims to elucidate the foundational knowledge necessary to appreciate chromatography's multifaceted applications. Understanding this technique is crucial for students, researchers, educators, and professionals engaged in various fields, including chemistry, biology, and environmental science.
Definition and Historical Context
Chromatography originates from the Greek words "chroma," meaning color, and "grapho," meaning to write. The technique was first employed in the early 20th century by the Russian chemist Mikhail Tsvet, who used it to separate colored pigments in plant extracts. Tsvet’s method laid the groundwork for the chromatography we know today, evolving into a sophisticated range of techniques that allow for the separation and analysis of compounds based on their physical and chemical properties.
Over the decades, chromatography has transformed remarkably. From its initial application in separating plant pigments, it has become indispensable in various scientific disciplines. Modern chromatography encompasses numerous types, such as gas chromatography and high-performance liquid chromatography, adapted for diverse substances. As industries and research fields grow increasingly complex, the evolution of chromatography remains vital to achieving precise and reliable analytical results.
Importance in Analytical Chemistry
Chromatography holds a central position in analytical chemistry due to its precision and versatility. The technique allows for the separation of components in mixtures, facilitating the identification and quantification of individual substances. This becomes especially significant in situations where accuracy is paramount, such as determining drug dosages or assessing environmental contaminants.
The benefits of chromatography extend far beyond mere separation;
- It provides insights into chemical composition and structure.
- It aids researchers in drug development and metabolic studies.
- It ensures compliance with regulatory standards in industries like food and pharmaceuticals.
For instance, in quality control, chromatography is often employed to test the purity of raw materials and finished products. Techniques such as high-performance liquid chromatography can detect impurities at trace levels, ensuring consumer safety and product efficacy.
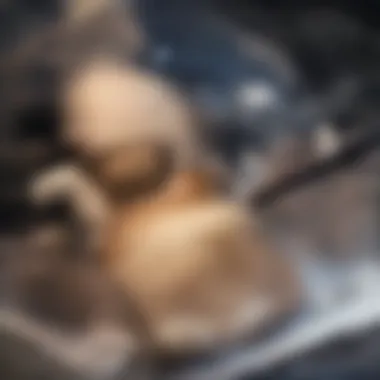
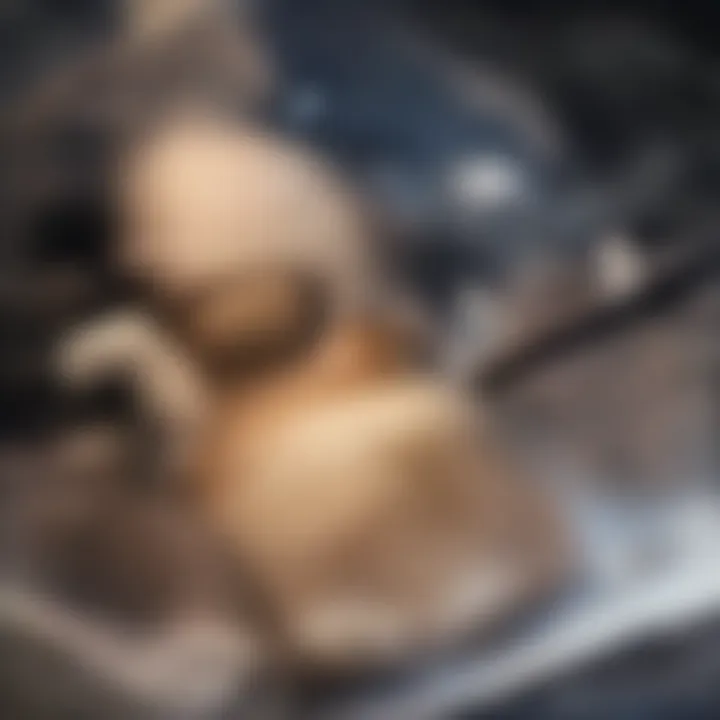
As this article progresses, it will detail the fundamental principles, various types, and applications of chromatography. Understanding these elements contributes significantly to a comprehensive grasp of the technique's role in both research and industry.
Fundamental Principles of Chromatography
Chromatography is a pivotal analytical method that hinges on fundamental principles governing its operation. Understanding these principles is crucial, as they ensure the efficacy and efficiency of the chromatography process. This section will delve into three core concepts: partitioning and elution, retention time and resolution, as well as the roles of the mobile and stationary phases.
Partitioning and Elution
Partitioning is the core principle that explains how components separate in chromatography. In this context, partitioning refers to the distribution of compounds between two phases—the stationary phase that remains fixed and the mobile phase that moves. When a sample mixture is applied, its various components interact differently with these phases. Some components will adhere to the stationary phase more than others, while others will prefer the mobile phase.
The process of elution involves the mobile phase carrying the sample through the chromatography system, gradually separating the components as they travel at different speeds. This separation happens because of the different affinities of each component for the two phases. In practical terms, higher affinity for the stationary phase results in slower movement, while greater solubility in the mobile phase leads to faster elution.
Retention Time and Resolution
Retention time is a critical measure in chromatography, indicating how long a component stays in the system before being detected. Each compound in the mixture has a unique retention time based on its interaction with the stationary and mobile phases. This characteristic helps in identifying substances in a mixture.
Resolution, on the other hand, quantifies the ability to distinguish between closely spaced components. A high resolution indicates clear separation, while low resolution can result in overlapping peaks in a chromatogram, leading to ambiguous results. Factors affecting resolution include the column length, particle size, and flow rate of the mobile phase.
Mobile Phase vs. Stationary Phase
In chromatography, understanding the differences between the mobile and stationary phases is vital. The stationary phase, usually a solid or liquid that is coated on a solid support, provides a surface for the separation process. Conversely, the mobile phase, which can be a gas or liquid, serves to transport the sample through the chromatography system.
The choice of these phases significantly impacts the separation efficiency. For example, in Gas Chromatography, the mobile phase is an inert gas while in Liquid Chromatography, it's a liquid solvent. Additionally, the interactions between sample molecules and these phases dictate the chromatography method's selectivity and sensitivity.
Effective understanding of these fundamental principles enables researchers to optimize chromatographic conditions for specific applications.
In summary, the fundamental principles of chromatography—including partitioning and elution, retention time and resolution, as well as the interactions between mobile and stationary phases—are indispensable for anyone looking to employ this technique effectively in scientific research and applications.
Types of Chromatography
The variety of chromatographic techniques forms the backbone of its applicability across diverse scientific fields. Each type possesses specific characteristics, advantages, and limitations that cater to different analytical needs. Understanding these types is essential for researchers and practitioners aiming to select the most suitable method for their work.
Gas Chromatography
Principle and Mechanism
Gas chromatography relies on separating volatile substances as they vaporize and pass through a stationary phase, often contained in a column. The mechanism primarily utilizes the differing affinities of compounds to the stationary phase, leading to varying retention times. This principled approach allows for efficient analysis of complex mixtures. Key characteristics such as high sensitivity and rapid analysis contribute to its popularity in both research and industry. However, its limitation lies in analyzing only volatile compounds, restricting its use in non-volatile substances.
Applications in Industry
In the industrial realm, gas chromatography plays a vital role in quality assurance and control. It is widely used in the petrochemical industry for analyzing hydrocarbon mixtures and in the food industry to ensure safety and compliance with regulations. Its specific applications enable precise detection of contaminants and the ascertainment of product consistency. This method's ability to provide timely results enhances its appeal, despite the operational requirements that may pose challenges for some facilities.
Limitations and Considerations
Gas chromatography has notable limitations. Its dependency on volatility means it cannot analyze non-volatile compounds effectively. Additionally, sample preparation must be meticulous to prevent degradation or loss of analytes during the process. Though effective, the need for specialized equipment and initial setup costs can be prohibitive for some smaller laboratories.
Liquid Chromatography
Liquid chromatography encompasses several sub-types, including High-Performance Liquid Chromatography (HPLC) and Ultra-High Performance Liquid Chromatography (UHPLC). This method is critical in many fields due to its versatility in handling various sample types.
High-Performance Liquid Chromatography (HPLC)
High-Performance Liquid Chromatography uses high pressure to push solvent through a packed column filled with solid particles. This technique facilitates high resolution and great separation power. Its popularity stems from its ability to handle a broad range of substances, making it a go-to choice in pharmaceutical chemistry. A distinct advantage is its capacity to analyze complex mixtures with precision, but the necessity for regular maintenance of high-pressure equipment may increase operational costs.
Ultra-High Performance Liquid Chromatography (UHPLC)
Ultra-High Performance Liquid Chromatography builds on the principles of HPLC by utilizing smaller particle sizes in its columns, leading to faster analysis times and increased efficiency. This method is particularly beneficial for high-throughput environments where time and accuracy are paramount. The unique feature of UHPLC is its ability to produce high-resolution results in a fraction of the time required for traditional HPLC. However, the equipment is costlier and may require specialized training for effective operation.
Applications in Clinical Research
In clinical research, liquid chromatography is indispensable for drug development and therapeutic monitoring. It allows precise measurement of drug concentrations and metabolites in biological samples. This analytical capacity is crucial for understanding pharmacokinetics in patients. The versatility in method adjustments aids in adapting to various biological matrices, providing significant advantages. However, the interpretation of complex data can require advanced expertise, presenting a challenge for some researchers.
Thin Layer Chromatography (TLC)
Thin Layer Chromatography uses a thin layer of adsorbent material on a flat substrate as the stationary phase. This straightforward technique is pivotal in many qualitative analyses.
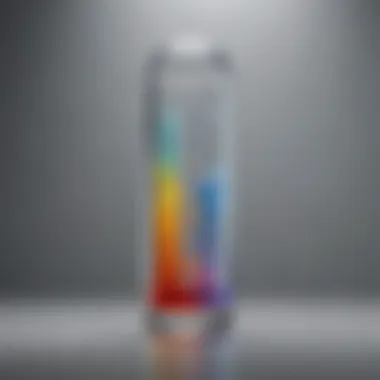
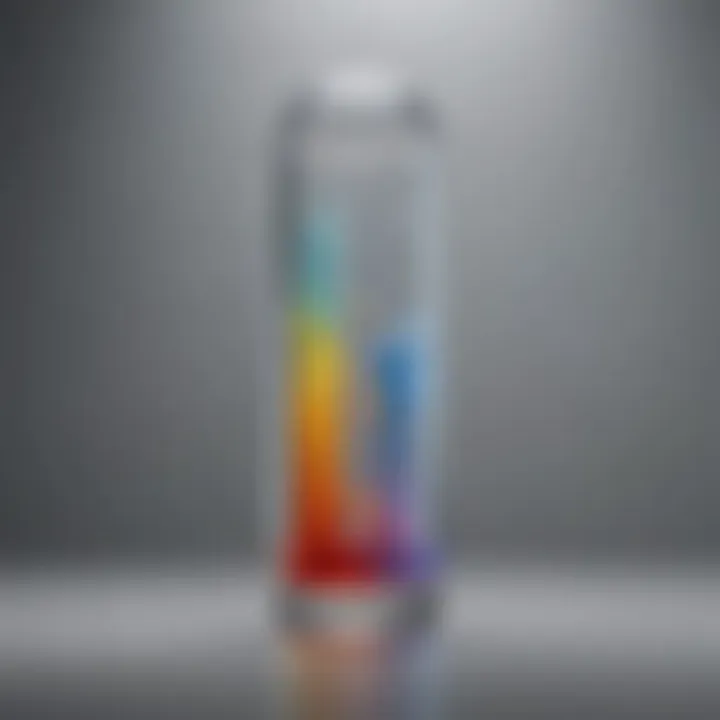
Basic Principles
The basic principles of TLC involve spreading a sample over a thin adsorbent layer and then allowing a solvent to move through capillary action. Compounds in the sample move differently based on their affinity to the stationary phase versus the solvent. This technique is valued for its simplicity and cost-effectiveness, allowing for quick reference and analysis in labs. However, it is less suitable for quantitative results compared to other techniques like HPLC.
Comparison with Other Methods
Compared to its counterparts, TLC is generally more accessible and less expensive. It is an excellent initial screening method that can guide further analyses. However, the lower resolution and reproducibility compared to HPLC or GC means it is usually more suited for qualitative rather than quantitative analysis.
Educational Use Cases
TLC is often favored in educational settings for teaching chromatographic principles. It allows students to visualize separation processes and understand the underlying science without complex instrumentation. While it is an effective educational tool, its simplicity may not prepare students for the intricacies of more advanced forms of chromatography used in professional environments.
Supercritical Fluid Chromatography (SFC)
Supercritical Fluid Chromatography employs supercritical fluids, usually carbon dioxide, as the mobile phase. This method provides unique operational characteristics that can enhance various analytical processes.
Operational Characteristics
SFC combines some advantages of both gas and liquid chromatography, such as fast analysis times and the ability to separate non-volatile compounds. Its operational characteristics include lower viscosity of supercritical fluids, which leads to better mass transfer. This results in sharper peaks and improved resolution. However, the technology requires specialized equipment and understanding, which can limit its adoption.
Advantages over Liquid Chromatography
SFC offers several advantages, particularly in terms of solubility and the ability to handle a range of polarities. It generally uses environmentally-friendly solvents, aligning with green chemistry principles. The faster analysis time can significantly boost productivity in laboratories. However, the limited availability of methods and the need for more extensive training can present barriers for some users.
Experimental Applications
In experimental settings, SFC is effective for chiral separations, which are crucial in pharmaceutical developments. Its versatility in various applications, from natural product analysis to polymer characterization, enhances its appeal. Despite its strengths, it may lack universal acceptance across all analytical platforms, which can inhibit broader application in practice.
Mechanisms of Separation
Understanding the mechanisms of separation in chromatography is crucial for evaluating its effectiveness and applications. Each mechanism addresses how components in a mixture can be differentiated during the chromatographic process. Recognizing these mechanisms aids in selecting the appropriate type of chromatography for specific analyses. The techniques utilized in this domain can be influenced negatively by improper methods or conditions, emphasizing the need for thorough understanding.
Chemical Properties Involved in Separation
The separation of compounds during chromatography is largely governed by their chemical properties. These properties include polarity, charge, and molecular weight. In liquid chromatography, for instance, polar compounds interact differently with the stationary and mobile phases than nonpolar ones.
- Polarity plays a central role; polar substances tend to prefer polar solvents leading to different retention times compared to nonpolar substances.
- Ionization can further complicate dynamics. The presence of acidic or basic functional groups influences interactions with the stationary phase.
- Molecular weight also impacts separation efficiency. Larger molecules might move slower than smaller ones due to size exclusion.
It is important to consider these chemical attributes when designing separation processes. Failing to account for them can lead to inaccurate analysis results.
Physical Interactions in Chromatography
In addition to chemical properties, physical interactions contribute significantly to the separation processes in chromatography. Various interactions can occur between analytes and the stationary phase, including:
- Van der Waals forces: Weak attractions that exist between molecules can influence how substances interact with the stationary phase in liquid chromatography.
- Hydrogen bonding: This strong interaction can greatly affect how well components are retained by the stationary phase, particularly in polar environments.
- Electrostatic interactions: For charged molecules, the repulsion or attraction to the stationary phase can determine their movement during the separation process.
In recognizing these interactions, one can enhance the efficacy of chromatographic techniques. Understanding the balance of chemical properties and physical interactions allows for optimized separation conditions, leading to clearer results and better reproducibility in analyses.
Applications of Chromatography
Chromatography is a cornerstone in analytical chemistry, finding its relevance across many fields such as biochemistry, pharmaceutical sciences, and environmental science. It serves as a fundamental tool for separating and analyzing complex mixtures. Its applications extend from research and development to quality control in industries, underscoring its versatility. The benefits of chromatography include high sensitivity, the ability to analyze small sample sizes, and the capability to separate compounds that are very similar in structure. Its precision and efficiency make it an indispensable technique in laboratories worldwide.
Research and Development
Research and development benefit significantly from chromatography. The ability to isolate and analyze compounds quickly is crucial in various scientific inquiries.
Drug Development and Pharmacokinetics
In drug development, chromatography plays a vital role in the analysis of drug compounds. Its ability to separate substances makes it invaluable for studying pharmacokinetics, which examines how drugs are metabolized in the body. The key characteristic of this application is its precision in quantifying drug concentrations in biological samples. This precision allows scientists to optimize dosages and improve therapeutic efficacy. One unique feature of drug development using chromatography is its adaptability to different types of analyses, including purity tests and stability studies. Advantages include the ability to support the rigorous standards necessary for regulatory approval, while disadvantages may arise in terms of time-consuming methods and the need for highly skilled personnel to conduct analyses accurately.
Environmental Monitoring
Environmental monitoring is another significant application where chromatography shines. This technique is utilized to detect pollutants and analyze samples from water, soil, and air. The key characteristic here is the sensitivity that chromatography offers when identifying trace levels of contaminants. Given the growing concerns about environmental safety, chromatography has become increasingly crucial in monitoring compliance with environmental regulations. The unique feature of this application lies in its ability to analyze complex environmental matrices, where other methods may struggle. Advantages include comprehensive insights into pollution levels, but challenges can occur due to the variability in sample quality and the need for substantial calibration work when switching between different matrices.
Quality Control in Industries
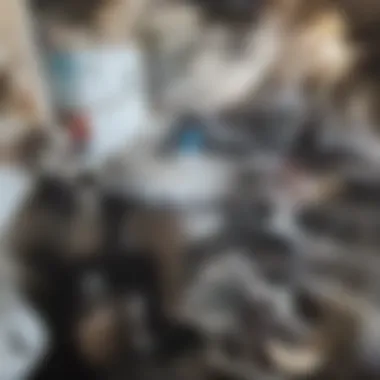
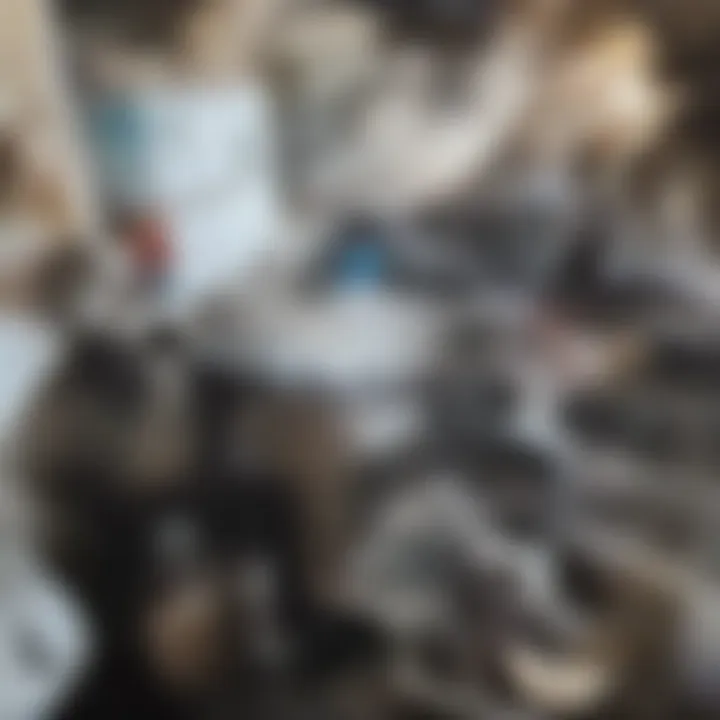
Quality control is essential in maintaining standards across various industries. Chromatography is a dominant method used to ensure product quality and safety.
Food Safety Testing
Food safety testing heavily relies on chromatography to detect harmful substances in food products. This aspect is critical for public health and safety. The key characteristic of food safety testing through chromatography is its capability to identify and quantify additives, contaminants, and natural toxins in food. It is widely regarded as a beneficial choice due to its accuracy and reliability. A unique feature of food safety testing is the use of methods like gas chromatography and high-performance liquid chromatography to assess food constituents. Advantages of this application include the ability to ensure consumer safety and compliance with health regulations, although disadvantages may include lengthy sample preparation times and the necessity for ongoing method validation.
Petrochemical Analysis
Petrochemical analysis illustrates the importance of chromatography in the oil and gas industry. This application absorbs a significant proportion of chromatographic techniques. The key characteristic is its role in analyzing hydrocarbon fractions from crude oil and monitoring fuel quality. Its continuity in research and application signifies why it is crucial within this industry. Unique features include the capability to separate complex mixtures into individual components for further analysis. The advantages of petrochemical analysis through chromatography are wide-ranging; however, disadvantages might involve high operational costs and the requirement for sophisticated instrumentation that may not be accessible to all facilities.
Chromatography remains vital in research, ensuring safety and compliance while enabling advancements in numerous scientific fields.
Future Trends in Chromatography
With the continuous evolution of scientific research and industrial applications, the field of chromatography is undergoing significant transformations. The future trends in chromatography highlight how advancements in technology can enhance analytical capabilities, improve efficiency, and open new avenues for application. These trends are essential not only for researchers and industries but also for advancing overall scientific knowledge.
Advancements in Instrumentation
Recent years have witnessed remarkable innovations in chromatographic instrumentation. These advancements aim to increase sensitivity and resolution while reducing analysis time. For instance, the development of faster detectors, such as mass spectrometers and fluorescence detectors, has improved the capability of chromatographic systems. These detectors allow for the analysis of smaller sample sizes with higher accuracy.
Moreover, automation in chromatography is becoming more prevalent. Automated systems, such as autosamplers, enhance reproducibility and reduce human error. Additionally, the integration of artificial intelligence in data analysis allows for better interpretation of results, while minimizing the time spent on routine tasks. This enables scientists to focus on more complex analyses without being bogged down by procedural requirements.
Benefits of Advancements
- Increased Sensitivity: Detection of compounds at lower concentrations.
- Shorter Run Times: Faster methods lead to increased throughput.
- Enhanced Reproducibility: Automation minimizes human error.
- Robust Data Analysis: AI improves the quality of results.
Integration with Mass Spectrometry
One of the most prominent future trends in chromatography is its integration with mass spectrometry (MS). This combination, often referred to as LC-MS (Liquid Chromatography-Mass Spectrometry) or GC-MS (Gas Chromatography-Mass Spectrometry), has become a cornerstone in analytical chemistry. The integration allows for detailed analysis following separation, providing both qualitative and quantitative data.
Mass spectrometry effectively identifies and quantifies complex mixtures at very low levels, making it invaluable for applications in pharmaceuticals, environmental monitoring, and food safety. The future is likely to see even tighter integration between these technologies, streamlining workflows and enhancing analytical power.
The synergy between chromatography and mass spectrometry reshapes the horizons of analytical chemistry, paving lanes for novel discoveries.
Considerations for Integration
- Cost Implications: High-end equipment can represent a significant investment.
- Expertise Requirement: Users need specialized training to operate and interpret results properly.
- Method Development: Optimizing protocols for combined use may require extensive research and adaptation.
Challenges and Limitations
In the field of chromatography, understanding the challenges and limitations is crucial for both practitioners and researchers. Recognizing these factors helps to refine techniques and improve results. While chromatography is an essential analytical tool, it is not without its difficulties. Factors like method selection, instrument calibration, and sample condition can significantly impact the performance of the chromatographic technique.
Common Pitfalls in Chromatographic Techniques
Chromatographic techniques can often lead to unexpected outcomes due to certain pitfalls. One such common issue is incomplete separation. This can happen when the solvent system or stationary phase is not suitable for the compounds being analyzed. The choice of mobile phase plays a crucial role in ensuring optimal performance.
Another significant challenge is overloading the column. When too much sample is loaded, it can cause peak distortion or tailing, affecting the resolution of the analysis. Additionally, temperature fluctuations can also lead to variations in retention times, making it essential to maintain consistent laboratory conditions. Experimenters must also be cautious of contamination, which can skew results and lead to misinterpretations. Ensuring thorough cleaning of apparatus and employing suitable controls can mitigate these issues.
Cost and Access to Technology
The cost associated with chromatography can often be a significant barrier. Advanced chromatographic systems, like High-Performance Liquid Chromatography (HPLC) and Mass Spectrometry integration, come with high price tags that may restrict access for smaller labs or academic institutions. This limitation can hinder research due to inadequate resources.
Furthermore, maintenance fees and operational costs for these systems can accumulate over time, making regular usage economically challenging. Another consideration is the availability of qualified personnel. Adequate training is required to use these instruments effectively. Without trained professionals, labs may not utilize the technology to its full potential, which in turn can limit research capabilities.
"Access to advanced chromatography technology is often uneven, leading to disparities in research advancements across institutions."
Inclusion of affordable but effective alternatives may help in some cases. Nonetheless, the gap in access can result in a stagnation of knowledge in certain areas of research. Looking ahead, the chromatography community must work towards making these technologies more accessible, ensuring that innovative research continues to thrive.
Ending
The conclusion of this article serves a pivotal role in consolidating the information presented regarding chromatography, emphasizing its complexity and importance in scientific inquiry. It centers on the significance of understanding chromatography as a fundamental analytical technique widely utilized across various disciplines, from chemistry to environmental science. Readers gain an understanding of its applications, benefits, and the intricacies that come with its usage.
Summary of Key Points
Chromatography stands as a cornerstone in analytical science, crucial for both qualitative and quantitative analysis. Here are the essential aspects:
- Fundamental Principles: The basic mechanisms involve partitioning, retention time, and the interaction between mobile and stationary phases, which are essential for effective separation and analysis.
- Types of Chromatography: Various chromatographic methods, such as gas chromatography and high-performance liquid chromatography, serve different purposes and industries. Each type carries its own advantages and limitations.
- Applications: The applications of chromatography extend across research domains. They include drug development, environmental monitoring, and food quality control, showcasing its versatility and importance in scientific practices.
- Future Trends and Challenges: As technology evolves, new instrumentation and integration with methods like mass spectrometry are emerging. However, challenges such as cost accessibility and common pitfalls remain relevant considerations for practitioners.
The Future of Chromatography
Looking ahead, the field of chromatography is poised for transformative growth. With the ever-increasing demand for accurate analytical techniques, advancements in technology are crucial. Innovations in instrumentation could lead to more efficient, rapid, and precise methods. Furthermore, the integration of chromatography with mass spectrometry promises enhanced sensitivity and specificity in analyses. As researchers and industries attempt to tackle global challenges, chromatography will maintain its vital role in ensuring quality and safety in numerous applications.
To effectively navigate this evolving landscape, professionals need to stay abreast of current trends and technological improvements. Understanding these dynamics will foster more effective uses of chromatographic techniques in both academia and industry, ultimately contributing to advancements in scientific research and applied sciences.