Understanding Dendrites: Key to Neural Function
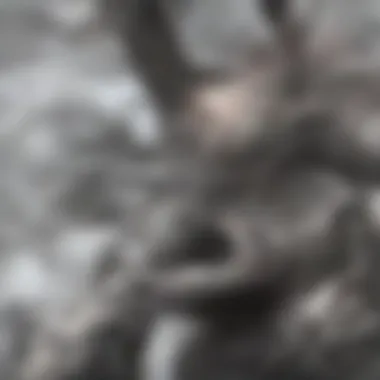
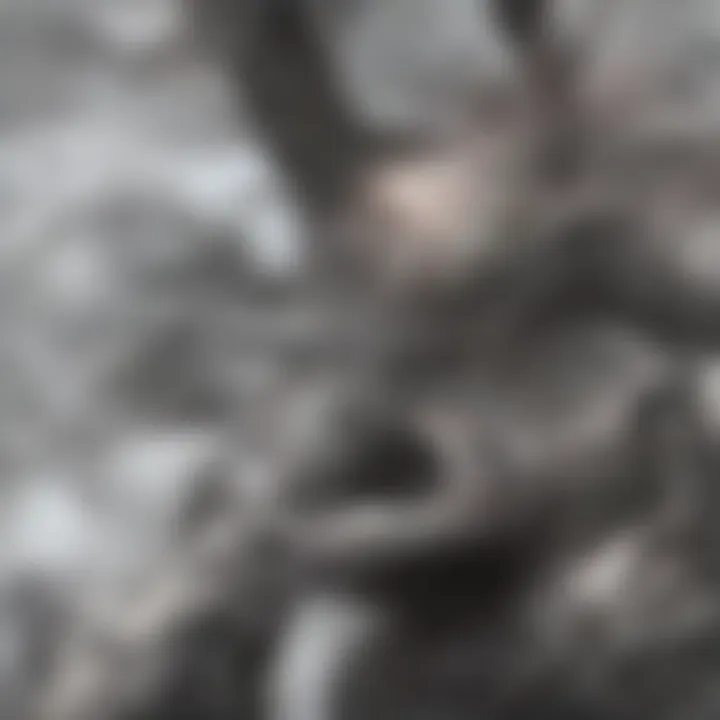
Intro
Dendrites play a pivotal role in the functioning of neurons. These branched extensions are crucial for receiving synaptic inputs, and they significantly influence how neural signals are processed. Understanding dendrites offers insights into the fundamental operations of the nervous system, including their impact on learning, memory, and various neurological disorders. This article aims to provide a comprehensive examination of dendrites, their structure, their developmental processes, and their functional implications.
Research Methodology
Description of Research Design and Approach
The study of dendrites requires a multifaceted research approach. Various methodologies are utilized to explore their structure and function. For instance, advanced imaging techniques like two-photon microscopy allow researchers to visualize dendritic spines in live neurons.
Optogenetics is also used to manipulate neuronal activity with light, enabling a deeper understanding of dendritic function in real-time. This allows researchers to observe how dendrites respond to synaptic inputs and how these responses underpin cellular communication.
Materials and Methods Used in the Study
Researchers often use a combination of electrophysiological recordings and live imaging techniques.
- Morphological Analysis: Techniques such as Golgi staining or fluorescent labeling clarify dendritic structure and morphology.
- Electrophysiology: This involves measuring the electrical activity of dendrites to understand their role in synaptic integration and signaling.
- Genetic Manipulation: Utilizing specific gene editing tools helps to study the effects of genetic variations on dendritic development and function.
Discussion and Interpretation
Interpretation of Results in the Context of Existing Literature
Current findings suggest that dendrites are not merely passive receivers of inputs. They actively participate in processing synaptic signals. Recent studies have highlighted how the dynamics of dendritic spines correlate with cognitive functions. This aligns with existing literature that proposes a link between dendritic changes and learning processes.
Additionally, researchers noted that structural changes in dendrites could lead to dysfunction in neural circuits, potentially contributing to neurological disorders. This reinforces earlier theories about the importance of dendritic health in overall brain function.
Implications for Future Research or Practical Applications
Understanding dendrites offers several implications for future research. There are potential therapeutic targets in neurological diseases such as Alzheimer's and schizophrenia, where dendritic degradation occurs.
Future studies focusing on:
- Dendritic Plasticity: Investigating how environmental factors influence dendritic morphology and function.
- Pathological Changes: Exploring how specific diseases alter dendritic behavior.
- Interventional Strategies: Developing approaches to restore normal dendritic function in affected individuals.
Such research could significantly enhance therapeutic strategies in treating cognitive impairments related to dendritic dysfunction.
Prelims to Dendrites
Dendrites are essential components of the neuronal structure, acting as the primary sites for receiving signals from other neurons. Understanding the role of dendrites is crucial, as they greatly influence neuronal communication, which underpins all aspects of brain function. This article delves into various aspects of dendrites, including their definitions, historical significance, anatomical features, and functional roles. By examining these elements, we can appreciate the complexity of neural networks and the foundational importance of dendrites in cellular function.
Definition and Importance
Dendrites are branched projections of neurons that receive synaptic inputs from other neurons. They play a crucial role in the integrative processes of the neuron, allowing for the convergence of multiple signals. Dendrites can vary significantly in size and shape, influencing how effectively they can receive stimuli.
The importance of dendrites arises from their critical function in signal transmission. When a neurotransmitter binds to a receptor on a dendrite, it can lead to a series of electrical changes within the neuron, ultimately resulting in the generation of an action potential. This impact on neuronal excitability is vital for learning, memory, and overall brain functionality. Moreover, any alteration in dendritic structure or functionality can have significant implications, potentially leading to neurological disorders such as schizophrenia or autism.
Historical Perspectives
The study of dendrites began in the early explorations of the nervous system, with notable contributions from scientists like Santiago Ramón y Cajal, who is often referred to as the father of modern neuroscience. Cajal's pioneering work in the late nineteenth century provided foundational insights into the structure and function of neurons. His detailed drawings revealed that dendrites are not simple, uniform extensions but instead exhibit a multitude of shapes and branching patterns.
Over time, advances in imaging techniques have allowed researchers to visualize dendrites with greater precision. The introduction of fluorescent microscopy and confocal imaging has significantly changed our understanding of dendritic complexity. These developments enable scientists to explore not only the physical properties of dendrites but also their dynamic nature during synaptic activity.
The historical context surrounding dendritic research illustrates an ongoing evolution of thought, one that continues to expand our knowledge and emphasizes the importance of these structures in overall cellular function. As we progress through this article, a clearer picture of dendrites' roles will be developed, highlighting how critical they are in neuroscience.
Anatomy of Dendrites
The anatomy of dendrites is fundamental to understanding their roles within the nervous system. Dendrites are specialized structures of neurons responsible for receiving synaptic inputs from other neurons. Their architecture enables them to effectively integrate signals. In this section, we will explore the basic structure of dendrites, the function of dendritic spines, and the variability in dendritic morphology. Each aspect plays a significant role in how these cellular components contribute to neuronal function.
Basic Structure
Dendrites possess a unique structural design that maximizes their ability to communicate with other neurons. They typically branch out from the soma (cell body) of the neuron. The surface of the dendrites is covered in tiny protrusions called dendritic spines. These spines serve as the sites for synapses, where neurotransmitters are received from presynaptic neurons. The main function of dendrites involves handling a large volume of information from various sources.
The diameter of dendrites is not uniform; they can vary greatly, which influences the electrical properties of the neuron. Thinner branches tend to be more resistant, while thicker ones can transmit signals more efficiently. This characteristic is crucial in determining how neurons process and transmit information.
Dendritic Spines
Dendritic spines are critical structures found on the surfaces of dendrites. They are small, mushroom-shaped projections that increase the surface area for synapses, thereby enhancing the neuron's ability to form connections with other cells. Each spine acts like a mini compartment, where receptors for neurotransmitters are concentrated. This spatial arrangement is vital for effective signal transduction.
The spines can change shape and size in response to synaptic activity. Such plasticity is important for learning and memory. Studies show that spines grow in response to increased neural activity, indicating that dendritic spines not only facilitate communication but also adapt based on experience.
Dendritic Morphology
Dendritic morphology refers to the shape and branching patterns of dendrites, which can be remarkably diverse among different types of neurons. This diversity is a direct influence on a neuron's computational capabilities. The varied forms, from simple to highly branched structures, can determine how neurons integrate synaptic inputs.
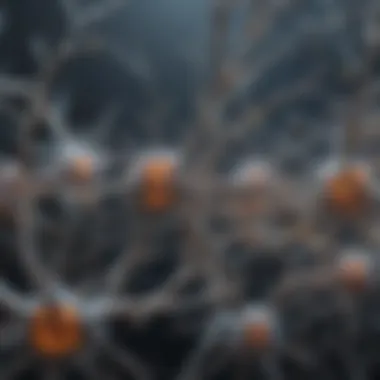
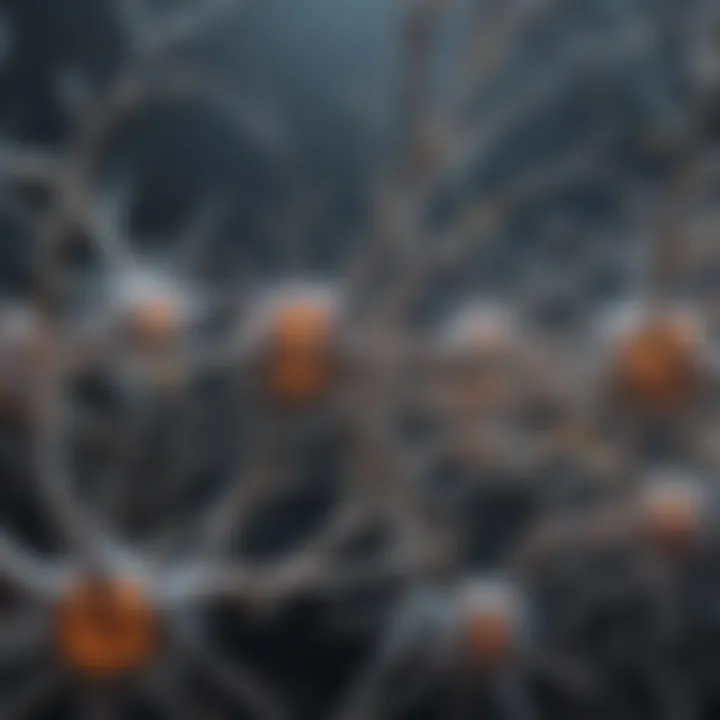
For example, pyramidal neurons in the cortex have long, apical dendrites with numerous branches. In contrast, granule cells in the hippocampus have shorter, more compact dendrites. Such distinctions are tied to the specific roles these neurons play in brain function.
Overall, dendritic morphology is responsive to both genetic programming and environmental factors. This adaptability allows for dynamic adjustments in neuronal networks, shaping overall brain function as learning occurs.
The complexity of dendritic structure and spines underlines the intricate computational functions of neurons in the brain. Understanding these aspects is crucial for grasping how information is processed at the cellular level.
In summary, the anatomy of dendrites is integral to their functionality. The basic structure, role of dendritic spines, and the diversity in morphology all contribute to their importance in neuronal signaling, information integration, and ultimately, neural communication.
Dendrite Development
Understanding dendrite development is crucial for comprehending how neurons establish and maintain connectivity within the neural network. Dendritic growth, branching, and maturation directly impact a neuron’s ability to integrate synaptic inputs. The capacity for effective synaptic integration depends on the morphological characteristics of dendrites, which evolve through distinct developmental stages. These stages influence how a neuron will connect with other neurons, affecting overall neural function and behavior.
Neuronal Differentiation
Neuronal differentiation is the initiation of specialized neuron formation from neural precursor cells. This process is significant in shaping the dendritic structure, which in turn influences synaptic activity. During differentiation, various genetic and environmental factors come into play to direct the specific patterns of dendrite formation. As neurons mature, they develop unique dendritic trees that are integral to their functions, such as receiving inputs and relaying information. The patterns that emerge during these early stages are often preserved throughout the life of the neuron, which can impact learning and adaptability.
One key aspect of neuronal differentiation is the role of intrinsic genetic programs. Specific genes regulate the expression of proteins that are essential for dendrite formation. These proteins help guide the growth and branching of dendrites, ultimately determining their shape and size. Additionally, extrinsic factors like neurotransmitters and glial cell interactions are essential modulators. For instance, neurotrophic factors such as BDNF (Brain-Derived Neurotrophic Factor) play a vital role in promoting dendritic growth and maintenance, highlighting the interdependence of genetic and environmental influences.
Influencing Factors
Several factors influence dendrite development. These can be classified into extrinsic and intrinsic variables that can either promote or inhibit dendritic growth. Understanding these factors is key to unveiling the complexity of neuronal architecture and its implications.
- Extrinsic factors: Environmental elements can significantly impact dendrite development. These include:
- Intrinsic factors: Genetic programming and molecular pathways also govern dendrite formation. Key aspects include:
- Neurotransmitter signaling: The presence and type of neurotransmitters contribute to dendritic branching and spines formation.
- Activity-dependent mechanisms: Neurons that are regularly activated develop more complex dendritic trees, reinforcing the concept that synaptic activity shapes dendritic morphology.
- Glial cell interactions: Glial cells provide essential support for neurons, influencing their growth and the formation of dendrites.
- Gene expression: Specific genes regulate neuronal identity, resulting in varied dendritic structures across different neuron types.
- Cellular signaling pathways: Several pathways, such as the mTOR signaling pathway, play essential roles in dendrite growth and organization.
Overall, the interplay between these factors determines the efficiency of synaptic integration, adding layers of complexity to the neural network. Dendrite development is a dynamic process, continuously influenced by both genetic predispositions and the surrounding environment. Recognizing these influences is essential for understanding neural plasticity and its implications in health and disease.
Dendritic Functionality
Dendritic functionality serves as a cornerstone in understanding how neurons process information. The complex network of dendrites allows neurons to receive and integrate signals from multiple sources. This functionality is not just a passive role; it actively shapes how neurons communicate with each other, influencing overall brain function. Understanding dendritic functionality is pivotal for grasping how information is relayed through neural pathways and how disruptions may lead to various neurological disorders.
Synaptic Integration
Synaptic integration pertains to how dendrites receive synaptic inputs and combine these signals to generate a neuronal response. Dendrites act as a critical junction, where excitatory and inhibitory signals converge. The spatial and temporal aspects of this integration are key to determining whether a neuron will fire an action potential.
Dendritic structures are equipped with various receptors that respond to neurotransmitters. These receptors govern how synaptic inputs are processed modelled on both the same neuron’s synapses and those from neighboring neurons.
- Excitatory Inputs: Generally, these inputs increase the likelihood of action potential firing by depolarizing the dendrite. For instance, glutamate is a common neurotransmitter that binds to these receptors.
- Inhibitory Inputs: These inputs, such as those mediated by GABA, serve to reduce the likelihood of firing, providing a necessary balance in neuronal signaling.
The integration of these signals is heavily influenced by the local dendritic architecture. Dendritic spines often hold synapses, aiding in the specificity of synaptic inputs from particular afferents. The entire process is complex, as it reflects the dynamic nature of synaptic activity and can be shaped by experience.
"The integration of synaptic inputs within dendrites showcases the neuron's remarkable ability to process multiple signals and determine its overall response."
Signal Processing
Signal processing in dendrites refers to how they manage, amplify, and transform synaptic inputs before relaying the information to the soma. This processing is vital for various cognitive functions. Dendrites are not merely passive conduits for electrical signals; they actively modify the signals based on their structure and biochemical environment. For instance, certain types of dendritic calcium channels can amplify depolarizations, influencing neuronal excitability.
Different dendritic regions may show distinct processing capabilities, indicating that the topographical arrangement of dendrites contributes to the nuanced responses of neurons. Factors such as dendritic morphology and the presence of certain ion channels determine how signals are interpreted. By doing so, dendrites contribute to processes like:
- Temporal Summation: Closely spaced inputs may combine effectively, leading to a stronger overall signal.
- Spatial Summation: Inputs from different parts of the dendrite can be integrated to determine the overall output.
Dendritic signal processing is susceptible to modulation through various factors, including experience and environmental changes. This capacity is fundamental to learning and adaptations within neural circuits.
Dendrites and Neural Communication
Dendrites are not merely passive receivers of electrical impulses; they play a crucial role in the complex network of neural communication. Their intricate architecture allows them to integrate synaptic inputs from multiple sources. This capability is essential for the processing of information within the central nervous system.
The significance of dendrites in neural communication lies in their ability to facilitate both local and distant signaling among neurons. They extend far-reaching branches that connect with synapses, enabling the transfer of information between neurons. This interaction is necessary for the proper functioning of neural circuits. Without healthy dendritic communication, the efficiency of these circuits could diminish, leading to various neurophysiological problems.
Role in Information Transmission
Dendrites contribute significantly to the process of information transmission in the brain. When an electrical signal reaches the synapse, neurotransmitters are released into the synaptic cleft. Dendrites have specialized receptors that bind to these neurotransmitters. This binding process converts the chemical signal back into an electrical signal, allowing the neuron to continue propagating the message.
Several aspects of dendrites enhance their role in transmitting information:
- Synaptic Weight: Different synapses have varying strengths. Dendrites can modulate these signals based on their overall structure and activity, a principle that underlies learning and memory.
- Integration of Inputs: Dendrites receive inputs from multiple neurons simultaneously. This convergence allows them to integrate diverse signals, contributing to their complex output.
- Signal Propagation: Dendritic trees can conduct electrical signals. This propagation is not uniform; it depends on the dendrite's geometry and the distribution of ion channels along its length.
In summary, the role of dendrites in information transmission is foundational to neuronal communication. They enhance computational capabilities by integrating inputs and modifying synaptic strengths.
Intercellular Communication
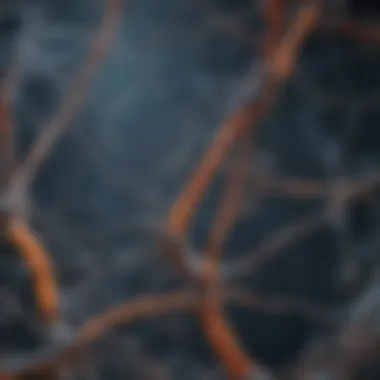
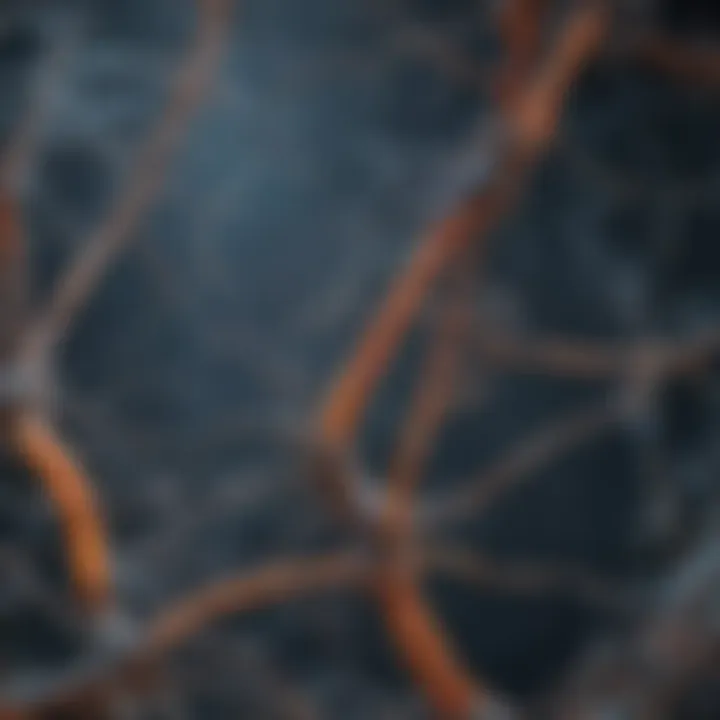
Beyond individual neuron functionality, dendrites also play a vital part in intercellular communication. This process encompasses how neurons communicate with one another as well as with glial cells. Understanding this communication offers insights into how neural networks function as a whole.
Dendrites facilitate intercellular signaling through:
- Chemical Signals: Neurons often release signaling molecules that act on nearby cells. Dendrites can detect these chemicals, influencing the activity of associated neurons or glial cells.
- Direct Contact: Some dendritic extensions make direct physical connections with other cells, allowing for rapid communication. This direct interaction is important for fast signaling processes.
- Modulatory Functions: Dendrites are involved in modulating the activity of neighboring cells. This ability to influence adjacent neurons can lead to synchronized patterns of activity, which is crucial during certain cognitive processes.
The interplay between dendrites and other cellular components enhances the overall communication network in the brain. Dendrites are fundamental in ensuring that information is not only transmitted but also effectively shared among different cell types.
"Dendrites serve as a bridge between individual neuronal signals and the broader circuitry of the brain, making them essential for both local and global communication within neural networks."
Understanding how dendrites function in communication can provide deeper insights into brain function and potential avenues for addressing neurodevelopmental disorders or neurodegenerative diseases.
Dendritic Plasticity
Dendritic plasticity refers to the dynamic changes in the structure and function of dendrites in response to environmental stimuli and neural activity. This process is vital for various aspects of neuronal function, including learning, memory formation, and recovery from injury. Understanding dendritic plasticity allows researchers to unravel the complex mechanisms that govern how neurons adapt and reorganize in response to experiences and challenges.
The ability of dendrites to change is crucial for synaptic inputs to be effectively processed. When a neuron receives frequent stimulation, its dendritic morphology can change, becoming more elaborate or efficient at integrating signals. This adaptability not only enhances the computational capacity of neurons but also contributes to the overall plasticity of the neural circuits.
Mechanisms of Change
Dendritic plasticity can be attributed to several underlying mechanisms. These mechanisms may involve:
- Structural Changes: Dendritic segments can grow or retract based on synaptic activity. This growth often leads to the addition or removal of dendritic spines, tiny protrusions on dendrites where synapses form.
- Biochemical Pathways: Certain signaling cascades, such as those involving calcium ions or protein kinases, play a significant role in mediating changes in dendritic structure and function.
- Gene Expression: Activity-dependent changes can also lead to the transcription of certain genes that encode proteins crucial for dendritic growth or re-organization.
"The capacity for dendrites to remodel themselves is a fundamental characteristic that supports learning and adaptation in neural networks."
Impact on Learning and Memory
Dendritic plasticity is fundamentally tied to learning and memory processes. When an organism learns, the synapses formed on the dendrites of neurons can undergo strengthening or weakening based on the patterns of activity. Such synaptic changes are reflected in altered dendritic morphology.
- Long-Term Potentiation (LTP): This is a persistent strengthening of synapses based on recent patterns of activity. Dendritic spines often increase in size and number during LTP, facilitating enhanced communication between neurons.
- Long-Term Depression (LTD): In contrast, LTD leads to decreases in synaptic strength. This often involves the retraction of dendritic spines, demonstrating how the neural circuits adjust based on experience.
- Memory Consolidation: As memories are formed and stored, dendritic networks become more refined. Structural changes in dendrites support the long-term retention of information, reinforcing the assertion that dendritic plasticity is not just a cellular phenomenon, but also a cornerstone of cognitive processes.
In summary, the study of dendritic plasticity provides insight into how cellular mechanisms underpin learning and memory. These processes have significant ramifications in understanding neurodevelopmental and neurodegenerative disorders, where alterations in dendritic architecture may contribute to cognitive deficits.
Pathological Alterations in Dendrites
Understanding pathological alterations in dendrites is crucial for deciphering the intricacies of various neurological disorders. Dendrites, as primary recipients of synaptic inputs, play a significant role in signal integration and neural communication. When their structure or function is compromised, it may lead to a cascade of physiological changes that contribute to or exacerbate diseases. The exploration of these alterations provides insights into the underlying mechanisms of neurodevelopmental and neurodegenerative conditions.
Neurodevelopmental Disorders
Neurodevelopmental disorders encompass a range of conditions that emerge during early brain development, resulting in impaired functions. These disorders include autism spectrum disorder, schizophrenia, and intellectual disability. Research indicates that dendritic abnormalities play a key role in their pathophysiology. For instance, altered dendritic spine density and morphology can disrupt synaptic connectivity, leading to impaired neural circuits that underlie cognitive and behavioral symptoms.
Genetic and environmental factors significantly influence dendritic development. The failure of proper dendritic growth can result in reduced synaptic activity, directly affecting learning and memory processes. Additionally, changes in the expression of specific proteins involved in dendritic structure, such as GluA1 and PSD-95, have been implicated in the pathology of various neurodevelopmental disorders.
- Dendritic Spine Density: Research suggests that decreased spine density is common in patients with autism and schizophrenia.
- Morphological Changes: Irregularities in spine shape and size can impact synaptic signaling.
Overall, observing how dendritic structures deviate in these disorders may provide not only a better understanding of their origins but also guide potential therapeutic strategies.
Neurodegenerative Diseases
Neurodegenerative diseases, such as Alzheimer's disease, Parkinson's disease, and Huntington's disease, are characterized by progressive loss of neurological function. Dendrites are often severely affected, leading to synaptic dysfunction and subsequent cognitive decline. Studies show that dendritic atrophy is commonly associated with these diseases, which correlates with synaptic loss and cognitive deficits.
In Alzheimer's disease, for example, the accumulation of amyloid-beta peptides has been shown to induce dendritic spine loss and destabilization. This disrupts the complex signaling networks, exacerbating memory impairment. Similarly, in Huntington's disease, pathological changes in dendritic structure correlate with motor and behavioral disturbances.
Key factors contributing to dendritic alterations in neurodegenerative diseases include:
- Toxic Protein Accumulation: Proteins such as tau and alpha-synuclein negatively impact dendritic integrity.
- Oxidative Stress: Increased levels of reactive oxygen species can lead to structural damage in dendrites.
Dissecting these pathological processes not only aids in understanding disease progression but also paves the way for developing targeted therapies aimed at preserving dendritic structure and function.
The intricate relationship between dendritic pathology and neurological function underscores the importance of early diagnosis and intervention in neurodevelopmental and neurodegenerative conditions.
Research Methods for Studying Dendrites
The study of dendrites is vital for understanding neuronal function and pathology. Dendrites are crucial in processing synaptic inputs, influencing how information is integrated within the neuron. Therefore, research methods focusing on dendrites have substantial implications for neuroscience. They help in revealing the complexities of neuronal connectivity and the function of different types of dendritic structures. By employing various research techniques, researchers can discern important properties of dendrites, such as structural adaptations and functional dynamics, which are essential for grasping broader neural network activities.
Understanding dendrites' structure and function through proper research methodologies enhances our comprehension of their contribution to neurological health and diseases. Therefore, selecting the appropriate methods is paramount. This section elaborates on two primary approaches: morphological techniques and functional imaging.
Morphological Techniques
Morphological techniques provide insight into the detailed structure of dendrites. These methods include:
- Electron Microscopy: This technology allows for high-resolution imaging of dendritic structures at the cellular level. It is particularly valuable for studying dendritic spines, which are key sites of synaptic connectivity.
- Confocal Microscopy: This technique enables three-dimensional imaging of dendritic structures. It is beneficial for observing live cells and can be combined with various fluorescent markers to highlight specific proteins or cellular processes.
- Tracing Techniques: By using dyes or genetic modifications, researchers can visualize the entire dendritic arborescence. This helps in studying their connectivity and overall morphology, revealing how different shapes correlate with specific functions in neurons.
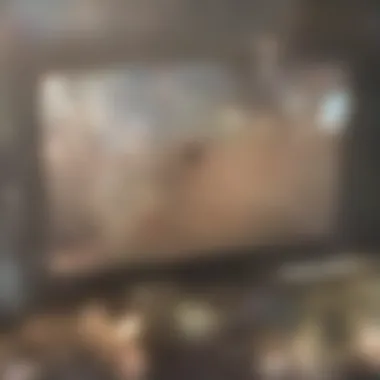
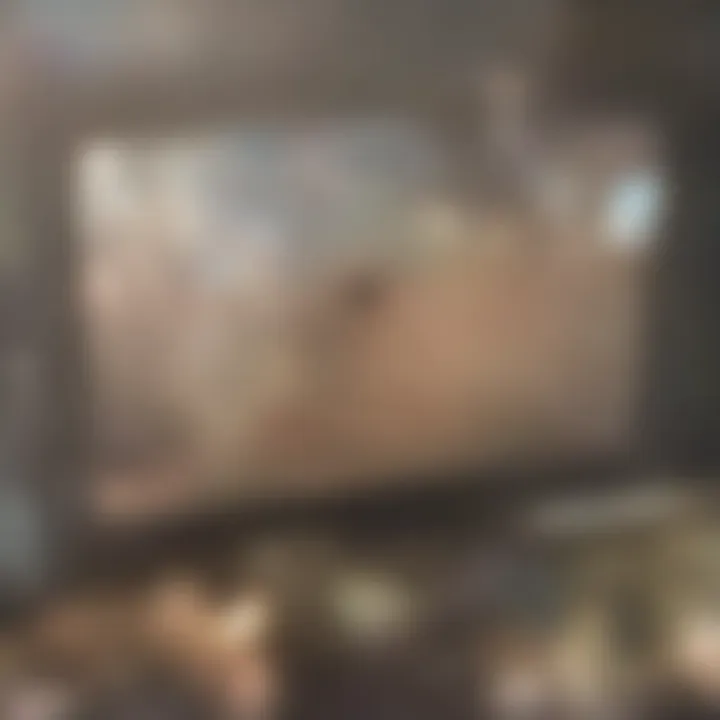
The importance of these morphological techniques cannot be overstated. They lay the groundwork for understanding how dendrites’ structure influences their function in synaptic integration and plasticity. Such insights are crucial in developmental studies and in understanding alterations due to diseases.
Functional Imaging
Functional imaging techniques play a significant role in studying how dendrites operate in real-time under physiological conditions. These methods include:
- Calcium Imaging: This approach measures calcium fluctuations within dendrites, which is crucial for understanding synaptic activity and integration. Changes in calcium levels are direct indicators of neuronal activity, thereby providing insights into functioning of dendrites during synaptic transmission.
- Optogenetics: By using light to control neuron behavior, optogenetics has revolutionized the study of neuronal circuits. Researchers can selectively activate or inhibit dendritic processes, which helps to ascertain their role in specific behaviors and synaptic functions.
- Two-Photon Microscopy: This technique allows for deep tissue imaging of live neurons, monitoring their activity while maintaining their physiological environment. It enables observation of dendritic dynamics during synaptic transmission, which is key for understanding the mechanisms of learning and memory.
Functional imaging expands our knowledge on how dendrites communicate and process signals. It allows for a better understanding of the timing and coordination of synaptic signaling. Therefore, integrating both morphological and functional techniques provides a holistic view of dendrites’ role in cellular function.
"The investigation of dendritic structure and functionality via advanced research methods enhances our understanding of neural circuits and lays the foundation for addressing neurological disorders.”
These methodologies are essential for future breakthroughs in dendritic research, offering insights that can lead to the development of therapeutic strategies for various brain disorders. Overall, the integration of morphological and functional studies will continue to shape our understanding of neuronal dynamics, paving the way for future discoveries in neuroscience.
Comparative Dendritic Studies
Comparative dendritic studies serve as a vital focal point in understanding the diverse roles of dendrites across different organisms. This exploration goes beyond mere anatomical observations; it investigates the functional implications of dendritic variations, offering insight into how neurons adapt based on species-specific neural demands.
One significant element of comparative dendritic studies is the recognition of how environmental factors influence dendritic morphology and connectivity. By analyzing dendritic structures in various species, researchers can draw correlations between ecological niches and neural adaptations. Such comparisons highlight the flexibility of dendritic designs. Dendrites are not static structures. Their adaptability allows neurons to adjust to specific functional requirements, effectively tuning synaptic efficiency.
Moreover, these studies reveal evolutionary trends. Researchers often notice that dendritic structures have evolved to meet the increasing complexity of cognitive processes in higher organisms. Most importantly, studying simple organisms alongside complex mammals can illuminate basic principles of neural function that apply broadly across species.
"Understanding dendritic systems through comparative studies enhances our grasp of neural evolution and complexity. "
Some key points include:
- Investigation of morphological diversity: Shapes and sizes of dendrites vary widely. These variations reflect differing functional demands across species.
- Implications for neurobiological research: Discoveries from these studies can inform models of human brain function and disorders.
- Ecological influences on dendritic evolution: Environmental challenges can sculpt dendritic architecture, fostering unique adaptations.
Across Species
Dendrites exhibit significant variations in structure and function across species. For instance, in primates, the dendritic spines are often more complex than those found in rodents. This complexity suggests a greater capacity for synaptic integration and adaptability in response to experience. In contrast, simpler organisms may possess fewer spines, indicating different modes of information processing.
Studying these differences allows researchers to infer how specific environmental pressures or social behaviors influence neural architecture. For example, animal species that rely on advanced social interactions tend to exhibit more sophisticated dendritic formations. This suggests that dendritic morphology is closely linked to the ecological and social challenges faced by each species.
Evolutionary Perspectives
From an evolutionary standpoint, comparative dendritic studies offer critical insights into how neuronal structures have adapted over time. Dendrites are influenced by both genetic and environmental factors, making their study a window into evolutionary processes. Examining how dendritic structures have emerged across phylogenetic lines can provide evidence of shared ancestry and functional specialization.
Specifically, these studies can indicate trends in increasing dendritic complexity corresponding with the emergence of higher cognitive functions. For example, significant changes in dendritic architecture have been observed in the lineages of mammals as compared to simpler vertebrates. This suggests that as cognitive demands increase, dendritic systems evolve to facilitate enhanced synaptic flexibility and learning capabilities.
Future Directions in Dendritic Research
Research into dendrites is pivotal for advancing our understanding of neuronal function and pathology. The complexity of dendritic structures and their dynamic properties warrant a focused effort on future studies, especially given their connection to fundamental processes such as synaptic integration and plasticity.
Dendritic research is vital for several reasons:
- Understanding Neural Function: Dendrites are the key sites for synaptic inputs. By continuing to explore their roles, we can gain insight into how neurons communicate and process information.
- Pathophysiological Insights: The alterations in dendritic morphology and functionality are associated with numerous neurological conditions. By understanding these changes, we can better pinpoint therapeutic targets.
- Technological Advancements: With emerging technologies, researchers have new tools to examine dendrites in unprecedented detail, unlocking further knowledge about their adaptive nature and role in learning and memory.
"The future of dendritic research lies in enhancing our techniques to investigate their diverse functions in the nervous system."
Emerging Technologies
The advent of various technologies offers profound opportunities for dendritic research.
- High-Resolution Imaging: Techniques like two-photon microscopy enable detailed visualization of dendritic spines in live neurons, allowing researchers to study dendritic changes over time.
- Electrophysiological Methods: Patch-clamp techniques allow for the recording of electrical activity from dendrites. This data helps elucidate the functional roles of dendrites in synaptic transmission.
- Molecular Techniques: Advances in genetic tools, such as CRISPR-Cas9, permit manipulation of dendritic properties and their pathways. This leads to a more profound understanding of associated proteins and their contributions to dendritic functions.
These technologies collectively enhance our ability to analyze and comprehend dendritic behavior in both health and disease.
Potential Therapeutic Applications
Understanding dendrites opens pathways for novel therapeutic strategies. Potential applications include:
- Targeted Drug Delivery: If specific dendritic receptors or pathways involved in a disease can be identified, drugs can be designed to target these pathways, potentially easing symptoms.
- Neuroregenerative Treatments: Research may lead to therapies that promote the regeneration of dendrites, helping recover function after injury or in neurodegenerative diseases.
- Mental Health Interventions: Insight into dendritic changes in mental health conditions could foster the development of new treatments, targeting synaptic connectivity and plasticity.
Future exploration of dendrites signifies an important intersection of technology and therapeutic development, where the insights gained will enrich our understanding of cellular function at a fundamental level.
Summary
This section emphasizes the significance of understanding dendrites in cellular function. Dendrites are not merely passive structures; they are essential for integrating information in neurons. Their complex morphology allows them to receive synaptic inputs from many sources, making them crucial for neurotransmission. The exploration of dendritic roles is vital for comprehending how neurons communicate, process information, and adapt to changes in their environment.
By studying dendrites, researchers can unlock insights into various neuronal functions, such as learning and memory. Moreover, understanding dendrites can guide therapeutic approaches for neurological disorders. Recognizing their structural and functional diversity provides a foundation for biological research and contributes to a broader understanding of brain health.
Key Takeaways
- Dendrites play a central role in neuronal communication, influencing how signal input is processed.
- Their structural diversity is linked to functional variability, impacting various physiological processes.
- Enhancing knowledge about dendritic function can inform the development of treatments for brain disorders.
- Dendrites are pivotal for synaptic plasticity, a mechanism underlying learning and memory.
Implications for Future Studies
- Continued research on dendritic architecture may reveal how specific shapes correlate with unique functions.
- There is a need for advanced imaging techniques to visualize dendritic changes in real-time, facilitating the understanding of dynamic processes.
- Investigating dendritic alterations in disease models can shed light on the mechanisms of neurodevelopmental and neurodegenerative disorders.
- Emerging technologies can be leveraged to explore therapeutic targets along dendritic pathways, aiming to restore normal function in affected neurons.
"Dendrites are not just extensions of neurons; they are fundamental contributors to neural circuitry and behavior."