Understanding DNA Synthesis in Cell Cycle Dynamics
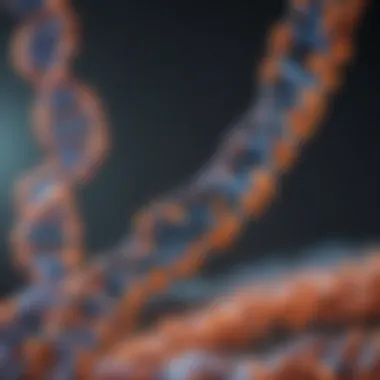
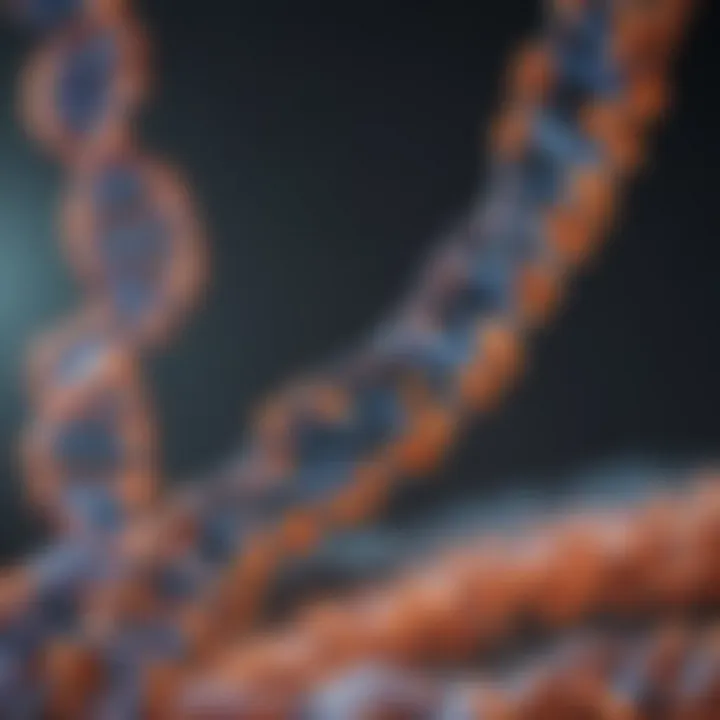
Intro
DNA synthesis is a crucial process that occurs in all living organisms, serving as the foundation for cellular functions and inheritance. Understanding this process is essential for anyone delving into cell biology, genetics, or related sciences. This section provides an overview of DNA synthesis during two pivotal phases of the cell cycle: interphase and mitosis. Each of these stages plays a distinctive role in ensuring accurate duplication and distribution of genetic material.
In interphase, DNA replication primarily takes place. This phase is divided into three sub-stages: G1 (gap 1), S (synthesis), and G2 (gap 2). Each stage has unique functions but collectively prepares the cell for successful division. Mitosis, on the other hand, is where the replicated genetic material is separated into two daughter cells.
This article aims to present a comprehensive guide on the role of DNA synthesis during interphase and mitosis, highlighting its significance in maintaining genetic stability and ensuring proper cellular function. By exploring current research findings and understanding regulatory mechanisms, readers can gain deeper insights into the broader implications of DNA synthesis in cellular biology.
Research Methodology
Research methodology in the context of studying DNA synthesis during interphase and mitosis involves both experimental and observational studies. The following steps outline the approach:
- Experimental Design: This includes controlled laboratory experiments that manipulate variables affecting DNA synthesis.
- Observation Techniques: Researchers use techniques such as flow cytometry and fluorescence microscopy to observe and analyze DNA synthesis.
The primary materials used in these studies include:
- Cell Cultures: Immortalized cell lines are often used for controlled studies. Examples such as HeLa or NIH 3T3 are common choices.
- Biochemical Reagents: Enzymes like DNA polymerases and nucleotides are essential for replicating DNA in vitro.
These methodologies allow researchers to gather significant data and interpret the mechanisms of DNA synthesis, leading to better understanding and potentially new applications in genetics and cell division.
Discussion and Interpretation
The interpretation of findings in DNA synthesis research has profound implications for understanding genetic stability. Studies have shown that errors during replication can lead to mutations, which may result in diseases such as cancer. Understanding the mechanisms regulating this process can inform therapeutic strategies.
Furthermore, ongoing research may reveal more about the regulatory factors guiding DNA synthesis. For instance, checkpoints in interphase can prevent cell division prior to complete DNA replication. This regulation is crucial for maintaining cellular integrity.
In future research, exploring the connection between DNA synthesis and epigenetic changes could provide further insights into gene expression and cellular differentiation. These considerations are essential for both basic science and medical applications.
"Errors in DNA replication can accumulate. Understanding and mitigating these errors is critical for preventing genetic diseases."
Through this discourse, the article aspires to not only engage students and professionals in the subject matter but also contribute to the growing body of literature on cellular biology and genetics.
Foreword to DNA Synthesis
DNA synthesis is a pivotal process in cell division and is critical for the continuity of life. Understanding DNA synthesis reveals how cells maintain genetic stability and integrity. This section will elaborate on the mechanisms of DNA synthesis, emphasizing its relevance in both interphase and mitosis. The insights derived from this discussion are essential for students, researchers, and professionals involved in genetics, molecular biology, and related fields.
Defining DNA Synthesis
DNA synthesis refers to the natural process by which cells replicate their DNA. This process is crucial during the cell cycle, primarily occurring in the S-phase of interphase. During DNA synthesis, the double-stranded DNA molecule unwinds. Each single strand then serves as a template for creating a new complementary strand. The enzyme DNA polymerase plays a vital role in this process, facilitating the addition of nucleotides to form the new strands. DNA synthesis is not a solitary event; it requires a complex interplay of enzymes, proteins, and regulatory mechanisms to ensure accuracy and efficiency.
Importance in Cellular Function
The functionality of cells heavily relies on precise DNA replication. Errors during this process can lead to mutations, which can disrupt normal cellular activities or contribute to diseases such as cancer. Furthermore, DNA synthesis is involved in cellular responses to environmental factors and stress. Repair mechanisms are activated if DNA is damaged, demonstrating how synthesis is closely linked to cellular health. The timely and accurate replication of DNA is foundational for growth, development, and repair processes within organisms. In summary, understanding DNA synthesis provides invaluable insights into how cells function and adapt, highlighting its essential role in the broader context of biological systems.
The Cell Cycle Overview
The cell cycle is a fundamental process that enables cell growth, replication, and division. Understanding its phases is crucial for comprehending how genetic material is accurately replicated and distributed during cellular division. The coordination of DNA synthesis and mitosis is vital for maintaining genetic stability in both individual cells and the organism as a whole. Any disruptions in this cycle can lead to serious consequences, including developmental abnormalities and diseases such as cancer.
Phases of the Cell Cycle
The cell cycle consists of distinct phases, primarily categorized into interphase and mitosis. Interphase accounts for the majority of the cell cycle and can be further split into three subphases: G1 (Gap 1), S (Synthesis), and G2 (Gap 2). Following interphase, the cell enters mitosis, where nuclear division occurs, leading to the formation of two daughter cells. Each of these phases plays an important role in ensuring that cells function properly.
- G1 Phase: This phase involves cell growth and preparation for DNA synthesis. The cell accumulates nutrients and synthesizes proteins necessary for the upcoming replication of DNA.
- S Phase: This is where DNA replication takes place. Each chromosome is replicated to form sister chromatids, thus ensuring that genetic information is preserved.
- G2 Phase: In this phase, the cell undergoes further growth and prepares for mitosis. The cell checks that DNA replication is complete and repairs any damage that may have occurred.
- M Phase (Mitosis): The final phase where active division occurs. Mitosis itself consists of sub-phases including prophase, metaphase, anaphase, and telophase.
Duration of Each Phase
The duration of each phase within the cell cycle can vary significantly depending on the cell type and environmental conditions. Generally, interphase takes much longer compared to mitosis. Here is a simplified overview of the average duration:
- G1 Phase: Typically lasts from several hours up to a day, depending on the cell type.
- S Phase: Often requires 6 to 8 hours for DNA synthesis to ensure accurate replication.
- G2 Phase: This phase usually takes 3 to 4 hours as the cell prepares for mitosis.
- M Phase: Mitosis is relatively brief, lasting roughly 1 to 2 hours.
Knowing the duration of each phase allows scientists to understand the dynamics of cell proliferation and to study anomalies that may arise during these critical processes.
"Understanding the cell cycle is key to unlocking the foundations of cellular biology and the management of diseases like cancer."
By examining the phases of the cell cycle and their durations, we gain insight into the intricate mechanisms that govern life at a cellular level.
Interphase as a Crucial Stage
Interphase is a pivotal part of the cell cycle and serves as a preparatory phase for mitosis. During this stage, the cell is not simply resting; rather, it is engaged in critical activities necessary for successful cell division. Understanding interphase is vital because it lays the groundwork for the replication and distribution of genetic material.
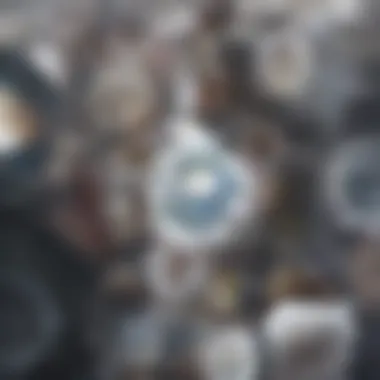
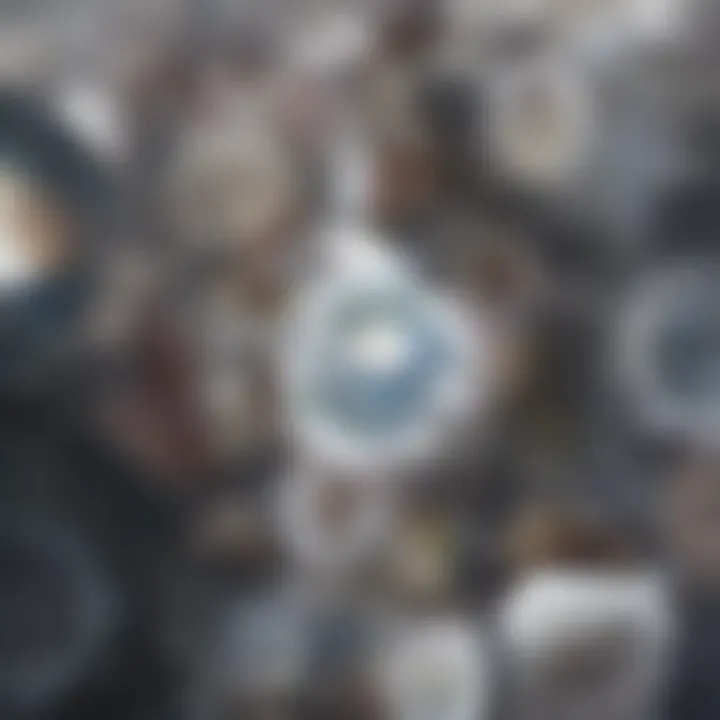
One major consideration about interphase is its role in ensuring that all cellular components are adequately replicated and checked before the cell enters mitosis. This reflection time is essential as it directly impacts the fidelity of DNA synthesis, which is crucial for maintaining genetic integrity. Without proper preparation during interphase, cells risk undergoing faulty division, leading to mutations or chromosomal aberrations.
A further benefit of emphasizing interphase is the opportunity to study various cellular processes that occur within this stage. From growth to DNA repair, interphase is rich with biochemical activities that are important for overall cellular functionality. The duration of this phase also varies significantly among different types of cells, further illustrating its complexity and importance.
Through the following sections, we will explore the definition, duration, and subphases of interphase. This analysis will reveal how interphase contributes fundamentally to DNA synthesis and its subsequent replication during mitosis.
Definition and Duration of Interphase
Interphase can be defined as the phase of the cell cycle where the cell spends the majority of its time, preparing for division. This phase is often divided into three distinct periods: G1 (Gap 1), S (Synthesis), and G2 (Gap 2). The duration of interphase varies among cell types, but it typically takes a significant portion of the total cell cycle time, often exceeding 90% in many somatic cells. The entirety of interphase can last from several hours to many days, depending on factors such as nutrient availability and environmental conditions.
The extended duration of interphase is crucial because it allows the cell to grow and replicate its DNA accurately. Missing the intricate processes that occur in this phase can result in numerous developmental and genetic issues.
Subphases of Interphase
G1 Phase
The G1 phase, or Gap 1 phase, marks the beginning of interphase. During this period, the cell grows and performs its normal functions. One key aspect of the G1 phase is the synthesis of proteins and organelles necessary for the cell's operation. This phase is characterized by intense metabolic activity, which prepares the cell for DNA replication.
Due to its nature, G1 serves as a beneficial choice for this article. Its emphasis on growth not only prepares the cell for the next stages but also impacts future mitotic divisions. However, the G1 phase can also face challenges such as DNA damage from environmental factors. These setbacks might necessitate the activation of checkpoint mechanisms to ensure genomic stability before advancing to the next phase.
S Phase
The S phase, or Synthesis phase, is where DNA replication occurs. It is perhaps the most critical phase in interphase concerning the topic of DNA synthesis. Here, the entire genomic content is duplicated, ensuring that each daughter cell receives an identical set of chromosomes. This phase is marked by the activity of specific enzymes, such as DNA polymerases, which catalyze the synthesis of new DNA strands.
The importance of the S phase in this article cannot be overstated. Its unique feature—complete DNA replication—contributes fundamentally to genetic consistency between generations of cells. Failure during this phase could lead to extensive genetic disorders, hence reinforcing the necessity of its proper execution and regulation.
G2 Phase
Lastly, the G2 phase, or Gap 2 phase, involves the final preparations before mitosis. In this phase, the cell continues to grow and produces proteins necessary for chromosome manipulation during division. Notably, G2 serves as a checkpoint, ensuring everything is in order before the cell commits to mitosis.
The G2 phase is beneficial for this article as it underscores the importance of quality control in DNA synthesis and prepares the cell for the rigorous demands of mitosis. Any inaccuracies detected in this stage can halt the cell cycle, preventing potential propagation of errors.
Understanding these subphases gives valuable insight into the significance of interphase in the context of DNA synthesis and cellular division. It illustrates the meticulous processes that fortify multicellular life against genetic instability.
DNA Synthesis in Interphase
DNA synthesis during interphase plays a crucial role in ensuring that the genetic material within each cell is accurately duplicated before division. This process is significant because it underpins cellular function and integrity. Without proper DNA synthesis, the accuracy of genetic information would be compromised, leading to detrimental effects on cell viability and organism health. Scientists have learned a lot about how interphase is structured to maximize the success of DNA replication. This understanding is key to advancements in genetics, cellular biology, and medicine.
Initiation of DNA Replication
The initiation of DNA replication marks the beginning of the synthesis process. It involves several steps, starting with the unwinding of the double helix at specific locations known as origins of replication. This phase is critical because it ensures that the entire genome is copied efficiently. The various proteins and enzymes involved in this process help define how and when replication occurs. Key players include specific origins recognition complexes that bind to the DNA, allowing for the appropriate assembly of replication machinery.
Enzymatic Action During Replication
DNA Polymerase
DNA polymerase is a vital enzyme that synthesizes new DNA strands. Its primary function is to add nucleotides to a growing DNA strand complementary to the template strand. A key characteristic of DNA polymerase is its high fidelity, which means it can add nucleotides accurately. This precision is essential to maintaining genetic integrity. The most popular type in many organisms is DNA polymerase III, particularly in prokaryotes, because of its processivity and efficiency.
An important feature of DNA polymerase is its proofreading ability. It can remove incorrectly paired nucleotides, which enhances the accuracy of the DNA replication process. However, the requirement for a primer to initiate synthesis can be seen as a disadvantage because it necessitates the involvement of another enzyme, primase, to create a starting point.
Helicase
Helicase is another critical enzyme that plays a role in DNA replication. Its specific function is to unwind the double helix, separating the two strands of DNA. This unwinding is fundamental for allowing access to the template strands that DNA polymerase needs for synthesis. A significant characteristic of helicase is its ability to use energy from ATP hydrolysis to move along the DNA and separate the strands effectively.
Helicase's unique feature is its rapid unwinding ability, which is crucial for maintaining efficiency during the replication process. However, its activity also puts strain on the DNA strands, leading to potential issues such as overwinding, necessitating other proteins to alleviate this stress.
Primase
Primase synthesizes short RNA primers that provide a starting point for DNA polymerase. This enzyme is essential because DNA polymerase cannot initiate the synthesis without the presence of a primer. Primase's key characteristic is its ability to create these short RNA sequences quickly, facilitating faster replication onset.
One advantage of primase is its flexibility in starting points, enabling replication to initiate at multiple sites along the DNA. However, the RNA primers must be removed and replaced with DNA later in the process, which adds complexity to the overall replication mechanism.
Replication Fork Dynamics
The replication fork is a critical structure that forms during DNA replication. It represents the area where the DNA strands are separated and new strands are synthesized. The dynamics at the replication fork involve the coordination of multiple enzymes and proteins to ensure smooth progression. Factors such as processivity and the synchronized activity of leading and lagging strand synthesis are essential here. Proper regulation of these dynamics is crucial for maintaining genomic stability and preventing errors during DNA replication. The challenges posed by the unwinding, synthesis, and ligation processes must all be addressed for successful completion of DNA replication in interphase.
Mitosis Overview
Mitosis is a fundamental process in cellular biology. It is the method by which a single cell divides into two daughter cells, each containing the same number of chromosomes as the original cell. Understanding mitosis is crucial for several reasons. Firstly, it plays a significant role in growth and repair of tissues. Every time a tissue needs to regenerate or when an organism grows, mitosis is responsible for providing new cells.
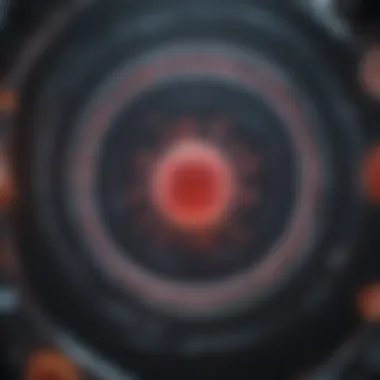
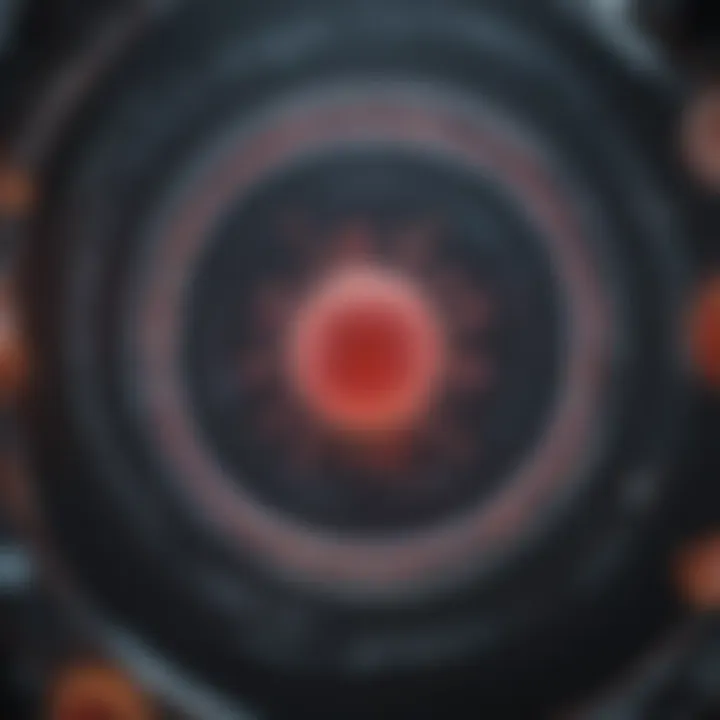
Moreover, mitosis ensures genetic continuity. Each daughter cell receives an identical set of chromosomes, which is critical for maintaining the genetic integrity of an organism. This process is not just essential for multicellular organisms; it also affects unicellular organisms, allowing them to reproduce asexually. Understanding these dynamics can reveal insights into developmental processes and the mechanisms behind cellular function.
In this section, we will delve into the distinct phases of mitosis, detailing how each phase contributes to the overall process of cell division.
Phases of Mitosis
Mitosis occurs in a series of steps, each with its own unique features and functions. The phases of mitosis consist of prophase, metaphase, anaphase, and telophase. Each phase plays a critical role in ensuring that cell division occurs accurately.
Prophase
During prophase, chromatin condenses into visible chromosomes. This is when the mitotic spindle begins to form. The nuclear envelope starts to break down, allowing spindle fibers to interact with the chromosomes. A key characteristic of prophase is chromosome condensation, which ensures that the genetic material is compact and organized for separation. This phase is beneficial for this article as it sets the stage for the ensuing steps that follow in mitosis.
A unique feature of prophase is its duration. While it may seem brief, its preparation is essential for the accuracy of the entire process. Disruptions at this stage can lead to improper segregation of chromosomes, potentially resulting in genetic disorders.
Metaphase
Metaphase is characterized by the alignment of chromosomes along the metaphase plate. This alignment is crucial because it ensures that each daughter cell will receive an equal and accurate set of chromosomes. A significant aspect of metaphase is the role of the spindle apparatus, which helps to organize and pull the chromosomes into position.
The key characteristic of metaphase is that it serves as a checkpoint to ensure that all chromosomes are correctly aligned and attached to spindle fibers. This is a beneficial point in the process as it minimizes the risk of errors that can disrupt genetic stability. Any anomaly during this phase can have serious implications for the cell's future viability.
Anaphase
In anaphase, the sister chromatids are pulled apart and moved toward opposite poles of the cell. This movement is driven by the shortening of spindle fibers. A defining characteristic of anaphase is the separation of chromatids, which symbolizes the cell's commitment to division.
This choice of content is essential as it highlights the dynamics of chromatid movement, which is crucial in maintaining genetic stability. A unique feature of anaphase is the potential for errors in one cell leading to mosaicism, where cells have different genetic compositions, stressing the critical nature of this phase.
Telophase
Telophase marks the near completion of mitosis. The chromosomes arrive at opposite poles and start to de-condense back into chromatin. The nuclear envelope re-forms around each set of chromosomes. This phase is characterized by the re-establishment of the nuclear structure.
Telophase is beneficial to understanding because it emphasizes the conclusion of sister chromatid separation and the re-establishment of two distinct nuclei within a single cell. A unique aspect of telophase is that although mitosis is winding down in this phase, cytokinesis soon follows to physically separate the two daughter cells. Understanding telophase is crucial for grasping how mitosis effectively culminates in cell division.
DNA and Mitosis
Understanding the relationship between DNA and mitosis is crucial in cellular biology. Mitosis is the process through which a single cell divides to produce two identical daughter cells. During mitosis, the integrity and structure of DNA is paramount. The accurate replication and segregation of DNA ensures that each daughter cell receives an exactly identical set of chromosomes. Therefore, the mechanisms governing DNA during mitosis directly influence the viability and functionality of future cells.
In mitosis, several factors come into play that are essential for the successful division of cells. These include the organization of chromosomes, the role of centromeres, and the overall coordination of the mitotic machinery. Each of these elements tether back to how DNA is structured and managed. Multiple checkpoints and regulatory pathways help maintain genetic integrity, minimizing the risk of mutations or abnormalities.
Chromosome Structure during Mitosis
During mitosis, DNA undergoes significant changes in its structure. Initially, DNA exists in a loosely packed form called chromatin during interphase. As the cell enters prophase, DNA condenses into tightly packed structures known as chromosomes. This condensation is crucial for ensuring that DNA is accurately separated during cell division.
Chromosomes are composed of two identical sister chromatids linked at a region called the centromere. Each chromatid represents a complete DNA molecule. As mitosis progresses through its phases, these chromatids must be precisely aligned along the metaphase plate before they are pulled apart into separate daughter cells.
The structure of chromosomes facilitates several key processes:
- Protection of DNA: The compact structure guards against mechanical stress during cell division.
- Alignment and Distribution: The spindle apparatus interacts with the chromosomes to ensure proper alignment and equal distribution into daughter cells.
- Gene Regulation: The chromatin structure can influence gene expression before and after mitosis.
Role of Centromeres
Centromeres serve as pivotal structures during mitosis, anchoring sister chromatids together. They are instrumental in the proper attachment of spindle fibers during cell division. When a cell divides, the centromere ensures that each sister chromatid is tugged toward opposite poles of the cell. This action is critical for maintaining genetic consistency across daughter cells.
The unique composition of centromeres, which often contain specific histone variants, plays a role in the functionality of these sites. Some important aspects of centromeres include:
- Kinetochore Formation: The kinetochore is a protein structure that forms on the centromere. It serves as the attachment point for spindle fibers during chromosome segregation.
- Spindle Checkpoints: Centromeres are involved in the spindle assembly checkpoint, monitoring whether chromosomes are correctly aligned before signaling the cell to proceed with mitosis.
- Mutations and Anomalies: Abnormalities in centromere function can lead to missegregation, resulting in aneuploidy or other genetic disorders.
By comprehending the intricate roles of DNA and its components during mitosis, researchers can better appreciate how cellular integrity is upheld. The understanding gained from these mechanisms holds significance for fields ranging from genetics to cancer research.
Comparing DNA Synthesis in Interphase and Mitosis
Understanding the differences and similarities in DNA synthesis during interphase and mitosis is important for several reasons. It highlights the dynamic nature of cellular processes and reveals how DNA replication is integral to maintaining genetic integrity.
DNA synthesis primarily occurs in the interphase stage of the cell cycle. However, the mechanisms, timing, and location of DNA replication differ significantly from those during mitosis. Knowing these differences can help researchers and students comprehend how failure in these processes can lead to genetic disorders or malignancies.
Timing and Location of DNA Replication
DNA replication is limited to certain phases of the cell cycle. The S phase of interphase is where DNA synthesis takes place. During this stage, the entire genome is replicated, ensuring that each daughter cell receives an identical copy of the DNA. In contrast, mitosis is primarily focused on the separation of already duplicated chromosomes into daughter cells. Therefore, no DNA replication occurs during mitosis.
The specific timing is crucial: in interphase, DNA synthesis prepares the cell for division, while mitosis ensures that the replicated chromosomes are organized and divided correctly.
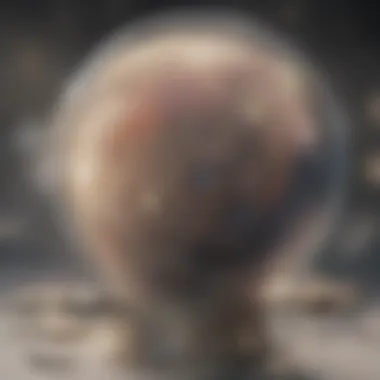
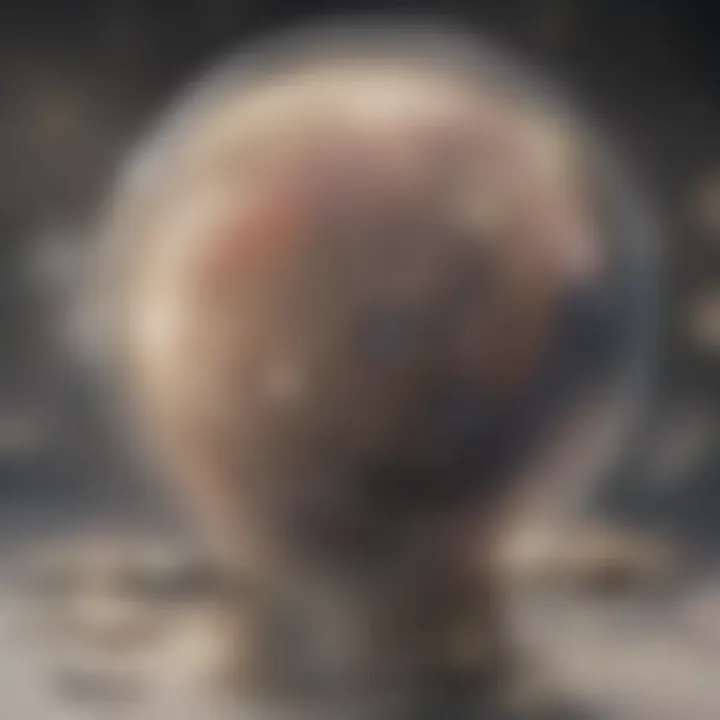
The location of DNA synthesis is also noteworthy. In eukaryotic cells, DNA replication occurs in the nucleus. In contrast, mitosis involves the organization and alignment of chromosomes in the mitotic spindle apparatus, which is a different phase that contributes to the segregation of genetic material.
Impact on Genetic Integrity
The processes of DNA synthesis during interphase and mitosis directly impact genetic integrity. Errors that occur during DNA replication in interphase can lead to mutations, which are then passed on to daughter cells. These mutations can cause genetic disorders if they disrupt critical genes or regulatory pathways. Moreover, they may also contribute to tumorigenesis, where uncontrolled cell division occurs.
Mitosis provides a framework for distributing the replicated chromosomes to daughter cells, ensuring that each cell inherits a complete and accurate set of genetic instructions. Failures during this phase, such as nondisjunction, can lead to aneuploidy, where the daughter cells have an abnormal number of chromosomes.
"A precise and orderly process of DNA synthesis is essential for maintaining genetic stability across generations of cells."
Regulatory Mechanisms in DNA Synthesis
Regulatory mechanisms play a significant role in DNA synthesis, ensuring that the process occurs accurately and efficiently. These mechanisms are vital for maintaining the integrity of the genome during cell division in both interphase and mitosis. Any disruption in these regulatory factors can lead to severe consequences, including mutations and cancer. Understanding how these mechanisms function can provide insights into both fundamental cellular biology and potential therapeutic targets.
Cyclins and Cyclin-Dependent Kinases
Cyclins are a family of proteins that are crucial in regulating the cell cycle. They work in tandem with cyclin-dependent kinases (CDKs), which are enzymes that, when activated by binding to cyclins, initiate various processes in the cell cycle.
- Role: Cyclins accumulate in response to specific signals in the cell, promoting transition through different phases of the cell cycle. Activated CDKs then phosphorylate target proteins, leading to a cascade of cellular responses necessary for DNA synthesis.
- Types of Cyclins: Different cyclins govern various checkpoints and phases. For instance, Cyclin D is associated with the G1 phase, while Cyclin E is critical for the transition from G1 to S phase, where DNA synthesis occurs.
The precise regulation of cyclins and their corresponding CDKs ensures that DNA replication proceeds only when the conditions are appropriate, reinforcing genetic stability.
Checkpoint Proteins
Checkpoint proteins serve as crucial guardians of the cell cycle, monitoring various conditions before allowing the progression of DNA synthesis. They ensure that cells do not replicate damaged DNA or divide prematurely.
- Key Functions:
- Importance: These checkpoints operate at critical transitions in the cell cycle, including the G1/S checkpoint and the G2/M checkpoint, effectively protecting the cell from propagating errors.
- Detection of DNA Damage: Proteins like p53 can halt the cell cycle in response to DNA damage, giving the cell time to repair itself before proceeding.
- Monitor Environmental Conditions: Some checkpoint proteins assess whether essential nutrients and growth factors are present.
"The role of regulatory mechanisms in DNA synthesis transcends mere cellular function; it is pivotal to our understanding of cancer biology and genetic diseases."
Overall, regulatory mechanisms in DNA synthesis are indispensable for the health of a cell and organism. By coordinating with various proteins and checkpoints, they guarantee that DNA replication occurs accurately and efficiently, reinforcing the importance of a well-regulated cell cycle.
The Consequences of Disrupted DNA Synthesis
Disruption in DNA synthesis can lead to significant ramifications for cellular health and function. When DNA synthesis does not occur correctly, the consequences extend beyond the individual cell; they can impact the entire organism. Understanding these effects is crucial for grasping how cellular processes maintain genetic stability and prevent diseases.
A key consequence of disrupted DNA synthesis is mutations, which are permanent alterations in the DNA sequence. These mutations can arise from various factors, such as environmental stressors, replication errors, or failures in the repair mechanisms that usually rectify these mistakes. Some mutations are benign, but others can disrupt normal cellular function and lead to genetic disorders.
Mutations and Genetic Disorders
Mutations can manifest in many forms, such as point mutations, insertions, or deletions. The impact of these alterations depends on their nature and location within the genome. For example, a mutation in a coding region of a gene may disrupt the protein's structure and function, leading to functional deficits. Consider the case of cystic fibrosis, caused by mutations in the CFTR gene. This genetic disorder results in severe respiratory and digestive problems due to the production of thick, sticky mucus in the lungs and pancreas.
Furthermore, mutations can be heritable. If they occur in germ cells, they can be passed on to subsequent generations, potentially leading to hereditary disorders. Genetic counseling and testing can help identify such risk factors. The relevance of mutations in the context of disrupted DNA synthesis cannot be overstated, as they form the basis for many genetic diseases, which substantially affect individuals and families alike.
Cancer: A Result of Failed Regulation
Another severe outcome of disrupted DNA synthesis is the development of cancer. Cancer arises when the regulatory mechanisms that control cell division and DNA repair fail. Normally, checkpoints within the cell cycle ensure that cells only divide when conditions are optimal and that any DNA errors are repaired. However, if DNA synthesis is compromised, errors can accumulate beyond the ability of the repair systems to cope, leading to uncontrolled cell growth.
Specific mutations in genes that regulate cell cycle progression, such as oncogenes and tumor suppressor genes, can propel a cell toward cancer. For instance, mutations in the TP53 gene, a crucial tumor suppressor, can lead to the loss of function necessary for cell cycle regulation and apoptosis. Failure of these checks results in cells dividing excessively, escaping normal growth constraints. Hence, understanding the interplay between disrupted DNA synthesis and cancer is essential for developing targeted therapies and preventive strategies.
In summary, the consequences of disrupted DNA synthesis can lead to profound issues, notably mutations that contribute to genetic disorders and the onset of cancer. Both require significant attention in research and treatment, underscoring the importance of maintaining genomic integrity in cellular processes.
Future Directions in DNA Synthesis Research
Research in DNA synthesis remains at the forefront of molecular biology. Understanding the intricacies of this process paves the way for advancements in various fields. As the knowledge base expands, several critical elements come into play. These include improved methodologies, innovative technologies, and the potential benefits these advancements could bring.
Technological Advances in Study Methods
Modern techniques continue to evolve, enhancing our comprehension of DNA synthesis. Some notable advancements include:
- Next-Generation Sequencing (NGS): This technology allows for rapid sequencing of vast amounts of DNA, enabling researchers to pinpoint minute changes in the genetic code.
- CRISPR-Cas9: This gene-editing tool facilitates targeted alterations in DNA, allowing for the study of specific genes in detail.
- Single-Cell Sequencing: This method investigates genomic variations at the cellular level. It sheds light on the diversity within cell populations that was previously obscured in bulk sequencing.
These techniques facilitate deeper insights into the timing and mechanisms of DNA synthesis and enable the study of its role in various biological contexts. Such advancements are crucial for tackling significant challenges in cellular biology and genetics.
Implications for Medicine and Therapy
The ramifications of ongoing research in DNA synthesis affect various medical domains. Understanding these mechanisms can lead to significant predictions and interventions in healthcare.
- Personalized Medicine: Advances in DNA synthesis research enable tailored therapies based on an individual's genetic information. This promises more effective treatment strategies with fewer side effects.
- Gene Therapy: Insights gained from studying DNA synthesis help refine gene therapy approaches, potentially curing genetic disorders by correcting or replacing faulty genes.
- Oncology: Discovering the role of DNA synthesis in cancer can lead to targeted treatments that inhibit uncontrolled cell division, offering more effective cancer management strategies.
"The future of medicine is in understanding and manipulating the very code of life itself."
In summary, the future directions in DNA synthesis research hold great promise. By integrating technological innovations and enhancing our understanding of DNA synthesis, we can unlock new potentials in the realms of medicine and genetics. The research landscape will continue to evolve, providing valuable insights that contribute to the betterment of human health.