Understanding Double Vibration: Mechanisms and Applications
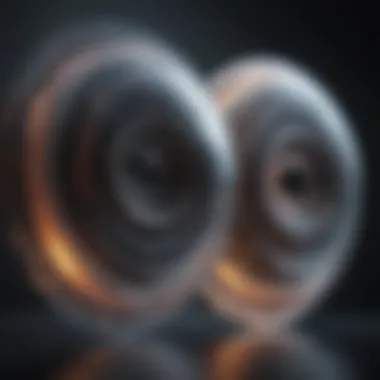
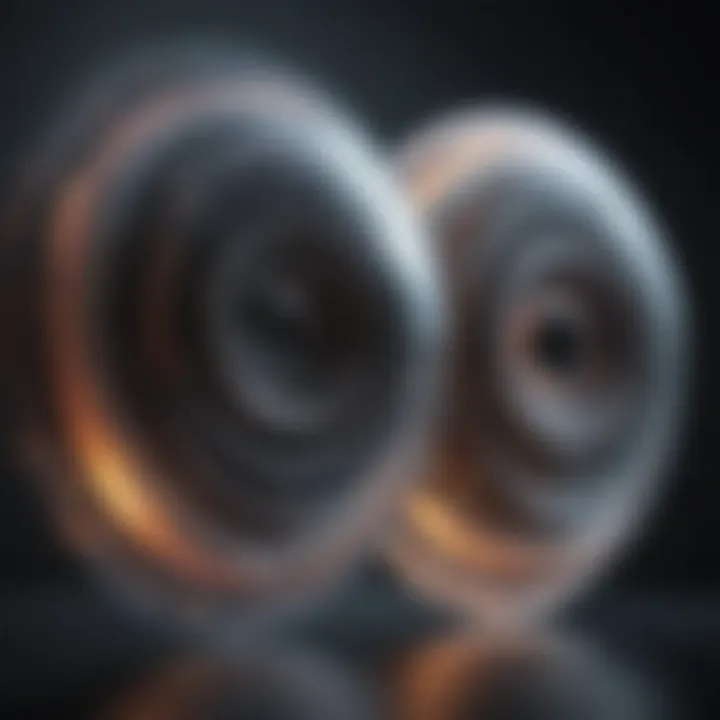
Intro
Double vibration is a complex and intriguing phenomenon that appears across various scientific domains, especially in physics and engineering. This topic is becoming increasingly relevant as technology progresses. Understanding the mechanisms of double vibration can guide innovations in design and functionality across multiple fields. It is pertinent to dissect this subject, not just for academic curiosity but for its potential practical applications.
This article will explore the core mechanisms, applications, and possible implications of double vibration. It seeks to provide readers—ranging from students to seasoned professionals—with valuable insights into how double vibration operates and affects contemporary science and technology.
By considering real-world applications and implications, we can foster a deeper appreciation of how this phenomenon shapes our understanding of dynamic systems. Let's dive deeper into the research methodologies that underpin the exploration of double vibration.
Prelims to Double Vibration
Double vibration is a topic of critical importance in various scientific and engineering fields. Understanding this concept is essential for researchers and industry professionals who rely on accurate vibration analysis in their work. The phenomenon of double vibration extends beyond just theoretical study; it has tangible implications in practical applications, such as structural engineering, mechanical systems, and even biological systems.
In this section, we will explore what double vibration entails and why it merits in-depth examination. By dissecting the foundational elements that constitute double vibration, readers can appreciate its complexities. All stakeholders—from students to seasoned professionals—stand to gain valuable insights by engaging with this subject. The discussion will also lay the groundwork for subsequent sections of the article, ensuring readers have a context as they dive deeper into the subject.
Definition and Conceptual Framework
Double vibration refers to a specific type of vibrational behavior that arises when two or more interacting systems resonate together. The conceptual framework encompasses various principles, including linear and nonlinear dynamics. In essence, these systems exhibit vibrational characteristics that are distinct from single-system vibrations. The interaction between the vibrations can result in various outcomes, such as amplifications or dampening.
This duality can be critical in designing technologies where vibration management is essential. Knowing how to manipulate these systems allows for innovations in engineering and better understanding in fields like geophysics or biology.
Historical Background of Vibration Studies
The study of vibrations can be traced back to early scientific inquiries into harmonic motion. While foundational concepts were established by physicists like Galileo and Newton, the modern understanding of double vibration emerged as researchers began to explore complex systems. In the 19th century, the development of wave mechanics by scientists such as Hermann von Helmholtz laid an early foundation for appreciating vibrational phenomena in a variety of contexts.
As technology evolved, so did the methods for studying vibrations. The advent of computational techniques in the 20th century allowed for more sophisticated modeling. The field has seen advancements in instrumentation, enabling researchers to measure and analyze vibrations with improved accuracy. Today, double vibration remains a topic of active research and application, with implications spanning multiple disciplines.
By understanding both the definition and historical context, readers can appreciate the depth and significance of double vibration. This sets the stage for exploring the physical principles that underpin the phenomenon.
Physical Principles Underpinning Double Vibration
Understanding the physical principles behind double vibration is crucial. It lays the foundation for appreciating how vibrations operate across various systems. These principles inform not only the theoretical aspects of double vibration but also its practical applications in fields such as engineering, acoustics, and geophysics. Emphasizing the mechanisms involved aids in grasping the dynamics that govern vibrational phenomena.
Basic Mechanical Vibration Theory
Mechanical vibration theory provides essential insights into vibrating systems. It details how forces interact to create oscillations and establishes a framework for analyzing them. Vibrations can be classified into free and forced types. Free vibrations occur when a system is displaced and allowed to oscillate on its own, while forced vibrations happen when an external force is applied.
The most significant component of this theory is the concept of natural frequency. Each system has a specific frequency at which it tends to vibrate. If an external force matches this frequency, resonance occurs, leading to increased amplitude. This situation can be both beneficial and detrimental, depending on the context of application.
Wave Mechanics and Resonance
Wave mechanics is pivotal in understanding how double vibration manifests in different contexts. It explores how waves propagate through various mediums. The principles of wave mechanics allow us to comprehend how energy and information are transmitted through vibrations.
Resonance plays a key role here, as it relates to how systems respond to external frequencies. When an external frequency aligns with a system’s natural frequency, the result is amplified oscillations. This principle is foundational in numerous applications, from musical instruments to structural engineering. Being aware of resonance’s effects helps engineers design structures that can withstand or utilize vibrational energy effectively.
Mathematical Modeling of Double Vibration
Mathematical modeling is integral for quantifying vibrational systems. It employs differential equations to describe motion and predict behavior. The modeling process involves defining system parameters such as mass, damping, and stiffness. By establishing these variables, researchers can simulate how double vibrations behave under different conditions.
Using tools such as MATLAB or Python, engineers can solve complex equations that represent double vibrations. This computational approach facilitates a deeper understanding of vibrational phenomena in real-world applications. Moreover, models can help identify potential issues before implementing design solutions in practical scenarios.
The exploration of these principles offers a comprehensive perspective on double vibration, guiding researchers and professionals in effectively leveraging this phenomenon for technological advancements. To broaden the understanding further, readers may explore resources from Wikipedia, Britannica, and platforms like Reddit for community insights.
Types of Double Vibration
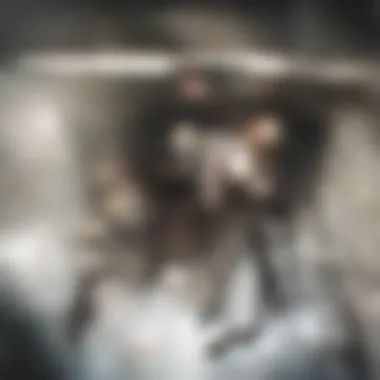
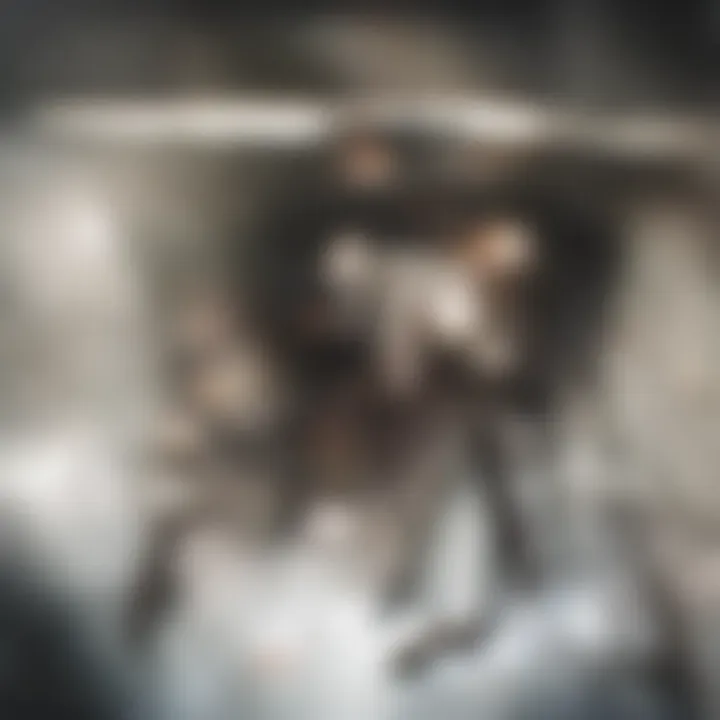
Understanding the various types of double vibration is essential for grasping the broader implications of this phenomenon. Each type presents unique properties and behaviors that can be crucial for applications across different scientific and engineering fields. This section explores specific elements such as linear and non-linear vibrations, coupled mechanisms, and the differences between damped and undamped vibrations. The knowledge gained here allows researchers and practitioners to tailor their approaches depending on the situation and desired outcomes.
Linear and Non-linear Vibrations
Linear vibrations refer to periodic motions that follow Hooke's law. These vibrations maintain a direct proportionality between the displacement of a system and the restoring force acting upon it. Systems exhibiting linear vibrations tend to be easier to model and predict, often displaying greater stability due to their predictable behavior.
In contrast, non-linear vibrations involve systems where displacement and restoring forces do not maintain a straight relationship. Non-linearity can lead to complex dynamics, including bifurcations and chaotic behavior. Understanding these differences is crucial for applications that require precision, such as in aerospace engineering or advanced robotics. A rigorous analysis of both linear and non-linear vibrations allows engineers to choose the right models for specific scenarios.
Coupled Vibrations and Their Mechanisms
Coupled vibrations occur when two or more systems interact, leading to energy exchange between them. This phenomenon is particularly relevant in environments where multiple components of a structure respond simultaneously to external forces. For example, a bridge experiencing wind forces may exhibit coupled vibrations in its beams and trusses.
The mechanisms behind these coupled vibrations often involve shared mass or stiffness properties. Mechanically, they can take the form of modes of vibration that affect one another. Understanding these interactions helps engineers in structural design, where the integrity and durability of systems are paramount. Designing effective dampening or isolation methods to mitigate unwanted coupled vibrations is a vital aspect of modern engineering.
Damped vs. Undamped Double Vibrations
Damped vibrations are those where energy is lost to the environment through mechanisms like friction or air resistance. This loss leads to a decrease in amplitude over time, which may be desirable in specific applications to prevent excessive movement that could be harmful.
Undamped vibrations, on the other hand, do not experience energy loss in such a manner. They often thrive in theoretical models or ideal conditions but may not be practical in real-world applications where damping is unavoidable. The distinction between these two types is significant. Engineers need to carefully assess if their systems will encounter damping, as it will influence the design and longevity of mechanical systems.
"Understanding the specific type of double vibration present in a system can greatly influence its design and function. A tailored approach considers the unique properties and requirements of each application."
Applications of Double Vibration in Technology
Double vibration plays a crucial role in technology across multiple fields. Understanding its applications can enhance innovations and improve systems' efficiencies. It is vital to explore various areas where double vibration manifests its effectiveness, such as structural engineering, mechanical systems optimization, and acoustic engineering.
Vibration Analysis in Structural Engineering
In structural engineering, vibration analysis is paramount in ensuring safety and reliability. Engineers utilize sophisticated tools to measure vibration responses of structures subjected to dynamic forces. Structures like bridges, buildings, and dams experience various loads that can induce vibrations. By analyzing these vibrations, engineers can assess the structural integrity and predict failure modes.
- Structural monitoring systems often integrate sensors like accelerometers to gather data about vibrations.
- This data allows for real-time assessment and enables preventative maintenance practices.
- Vibration analysis helps engineers design structures that can withstand unpredictable forces such as earthquakes or high winds.
Effective vibration analysis not only enhances safety but also prolongs the lifespan of structures, ultimately saving money and resources.
Role in Mechanical Systems Optimization
Optimization of mechanical systems is another critical application of double vibration. Engineers focus on minimizing vibrations in machinery to improve performance and efficiency. Excessive vibrations can lead to wear and tear and can negatively impact operational efficiency. It is essential to identify the sources of vibrations and utilize methods to mitigate them.
- Various techniques, such as damping materials and balanced rotors, help reduce unnecessary vibrations in equipment.
- Simulation software can model vibrations and predict system behavior, guiding engineers toward optimal design solutions.
By effectively managing vibrations, organizations can enhance machinery longevity and improve overall productivity.
Impact on Acoustic Engineering and Sound Design
In acoustic engineering and sound design, double vibration influences sound quality and performance. The behavior of vibrating systems directly relates to sound generation and propagation. An understanding of vibration mechanics allows sound engineers to design spaces and devices that optimize acoustic performance.
- For instance, speakers and musical instruments utilize vibration principles to produce sound. The quality of sound produced is significantly affected by how vibrations interact within these systems.
- Studio acoustics relies on calculation of vibrational modes to create environments that minimize unwanted reverberation or resonance.
The intricate relationship between vibration and sound underscores the importance of double vibration in shaping auditory experiences. From concert halls to home theaters, the application of double vibration improves sound clarity, depth, and richness.
"Double vibration is at the heart of modern technology, impacting everything from our buildings to our sound systems."
In summary, the applications of double vibration in technology are vast and varied. Each sector benefits from a deeper understanding and innovative approaches to vibration analysis, mechanical optimization, and acoustic engineering. These insights lead to safer, more efficient systems that enhance our day-to-day experiences.
Double Vibration in Natural Systems
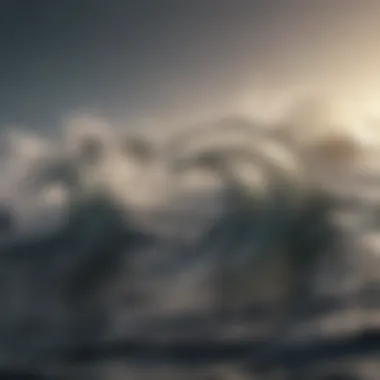
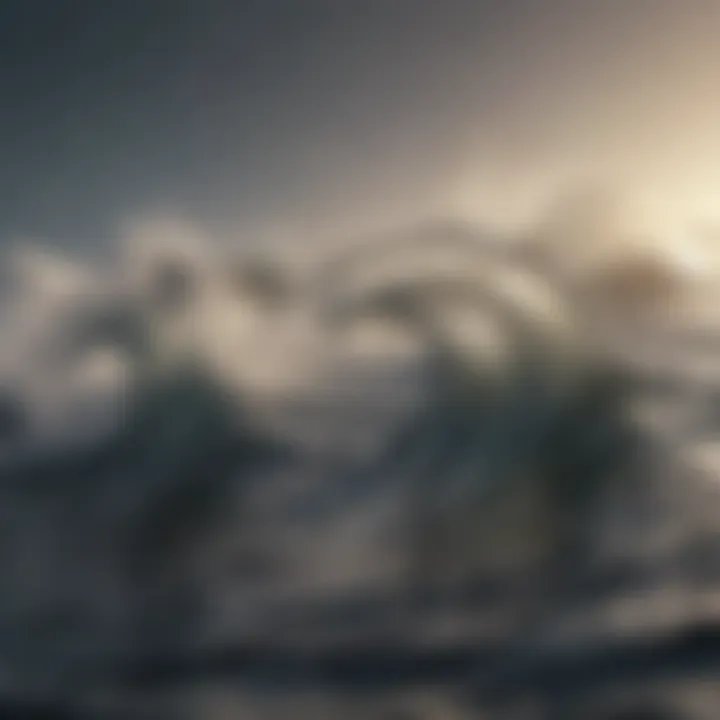
Double vibration plays a crucial role in natural systems, presenting fascinating insights into various phenomena. The study of double vibration in this context helps reveal underlying principles that govern both the physical and biological worlds. Understanding it leads to significant benefits, such as improved predictive models for geophysical events or enhanced insights into biological functions. By investigating this phenomenon in natural systems, we can gather key information that feeds into both academic and practical applications.
Vibrational Phenomena in Geophysics
In geophysics, double vibration manifests through various phenomena such as seismic waves and tectonic movements. When an earthquake occurs, the energy released propagates through the Earth. This energy travels in waves, often exhibiting characteristics of double vibrations. These waves can be P-waves (primary waves) and S-waves (secondary waves), which interact in complex ways.
Geophysicists study these waves to understand earthquake patterns and predict potential hazards. By analyzing vibrational data, scientists can create models that predict how waves will travel through different geological materials. This understanding aids in designing structures that can withstand seismic activity.
The significance of double vibration in this field cannot be understated. It allows researchers to:
- Enhance earthquake prediction: Modeling double vibrations improves the reliability of earthquake forecasts.
- Understand Earth’s internal structure: Studies of wave propagation provide a glimpse into the Earth's composition.
- Design better infrastructure: Knowledge of vibrational responses leads to safer engineering practices for buildings and bridges.
Biological Applications and Implications
In biology, double vibration highlights some fascinating phenomena such as cell signaling and organ functionality. In living organisms, vibrations can occur at molecular levels, impacting how cells communicate and respond to their environment. For example, protein folding processes may involve vibrational movements that influence the protein's function.
Additionally, double vibrations play a critical role in sensory systems. The inner ear processes sound through a delicate system of vibrating structures. Understanding how these vibrations propagate can lead to better treatments for hearing loss or balance disorders.
Some key aspects of biological implications include:
- Cellular Communication: Vibrational signals can influence cellular interactions, affecting growth and development.
- Hearing and Balance: Insights into vibrational mechanisms help in addressing auditory issues.
- Biomimicry in Design: Understanding biological vibrations can inspire the design of new technologies that mimic natural processes.
In summary, studying double vibration in natural systems enriches our comprehension across various scientific fields. Its implications reach far beyond simple observations, extending into practical applications and enhancing our ability to predict, design, and innovate.
"Understanding the vibrational dynamics of both natural and artificial systems bridges gaps in knowledge that could lead to groundbreaking advancements in technology and science."
This focus on natural systems showcases the interconnectedness of the vibrational phenomenon, illustrating its relevance in both geophysical and biological contexts.
Measurement and Analysis Techniques
The measurement and analysis of double vibration are crucial to understanding its underlying mechanisms and implications across various fields. This section addresses the significance of precise measurement techniques and robust data analysis methods in capturing the nuances of double vibration phenomena. Accurate instrumentation and thoughtful data interpretation lead to better applications in technology and science, allowing researchers and practitioners to derive meaningful insights and improvements.
Instrumentation for Vibration Measurement
Instrumentation serves as the foundation for effective vibration measurement. Various tools and devices exist to capture vibrational data accurately. Strain gauges, accelerometers, and piezoelectric sensors are commonly used in capturing vibrational signals. Each type has its advantages and limitations, influenced by factors such as the range of vibration frequencies, environmental conditions, and the specific characteristics of the system being analyzed.
- Strain gauges: Often used in structural applications, they measure the deformation of materials, providing insights into internal forces and vibrations.
- Accelerometers: These sensors measure acceleration and are beneficial for measuring high-frequency vibrations.
- Piezoelectric sensors: These convert mechanical stress into electrical signals, making them useful for both low and high-frequency vibrations.
In selecting instrumentation, one must consider the desired accuracy and sensitivity. Environmental factors can also affect measurements. Therefore, calibration and careful placement of sensors are essential. Ensuring precise measurement setups leads to reliable data, forming the basis for effective analysis.
Data Processing and Interpretation Methods
Once vibrations are measured, data processing is the next step. This involves analyzing raw data to extract meaningful patterns and insights. Effective data processing includes filtering noise, applying transformations, and utilizing mathematical models to interpret the vibrational signals comprehensively.
- Fourier Transform: Often employed to convert time-domain data into frequency-domain representations, making it easier to analyze the frequency components of vibrations.
- Signal Filtering: Removes unwanted noise, which is essential for accurate analysis. Low-pass or high-pass filters are commonly used depending on the application.
- Statistical Analysis: This helps in understanding variabilities and drawing correlations between different variables involved in double vibration.
Data interpretation plays a fundamental role in advancing the understanding of double vibration. The results obtained through analysis can uncover patterns related to system behavior, resonance phenomena, and potential optimization strategies for various applications.
"Understanding the limitations and strengths of your measurement techniques can lead to profound insights in vibration studies."
Challenges in Studying Double Vibration
In the exploration of double vibration, researchers encounter several notable challenges that complicate the study of this intricate phenomenon. Understanding these challenges is essential for advancing knowledge and improving applications across various fields.
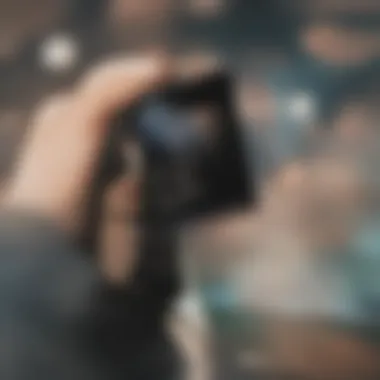
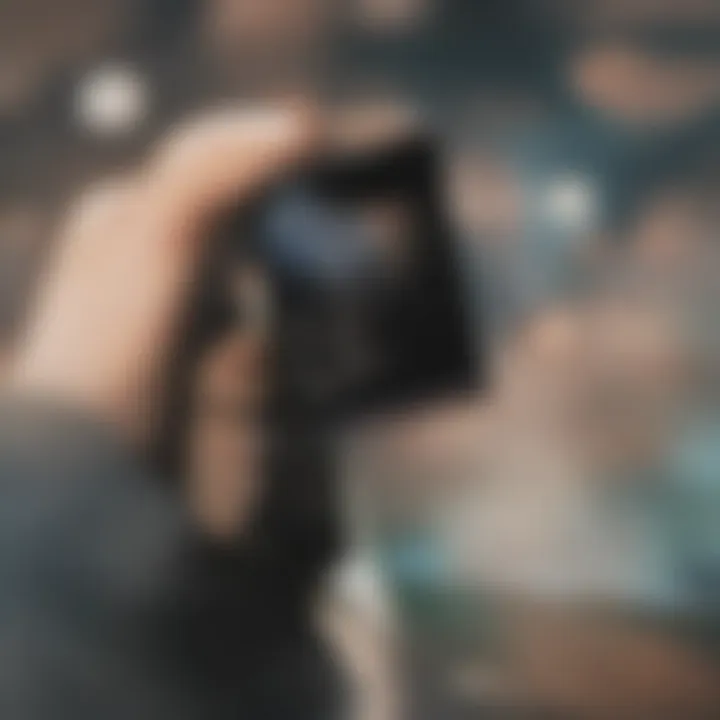
Complexity of Real-world Systems
The first major challenge stems from the complexity of real-world systems. Unlike controlled laboratory settings, many systems in nature and industry exhibit a multitude of variables and interactions that affect their vibrational behavior. For instance, structures like bridges and buildings must account for external factors such as wind, seismic activity, and material properties. This creates a complicated landscape where double vibrations may manifest in unpredictable ways.
Moreover, real-life applications often involve nonlinear dynamics, which can lead to unexpected responses during vibrational events. The interplay between multiple vibrating components adds another layer of complexity, often making it difficult to isolate specific effects. Researchers may find it challenging to derive conclusions that are universally applicable due to these variabilities.
Limitations of Current Theoretical Models
Another significant challenge is the limitations of current theoretical models. While numerous models exist to describe vibrational phenomena, they often simplify or overlook critical aspects of double vibration. Traditional vibrational analysis methods, such as simple harmonic motion, do not adequately capture the nuanced behavior of more complex systems.
Moreover, many models rely on assumptions that may not hold true in practical situations. For example, damping and coupled interactions are frequently not represented accurately. This gap between theory and real-world application can hinder the effectiveness of predictive analytics in engineering and scientific studies. Researchers continuously strive to develop more accurate models, yet this remains a persistent issue in the field.
Effective research must incorporate both experimental observations and refined theoretical considerations.
By addressing these challenges, future research can lead to a deeper understanding of double vibration and its applications in various technologies. Through innovative methodologies and a commitment to refining theoretical perspectives, scholars can push the boundaries of what is known about double vibration, fostering advancements that resonate across multiple disciplines.
Future Directions in Double Vibration Research
As the exploration of double vibration progresses, identifying future directions is crucial. This section highlights potential pathways for research. Understanding how double vibration can evolve may provide solutions to current challenges in various fields. The integration of emerging technologies alongside advanced computational methods represents significant avenues for advancing research.
Emerging Technologies and Their Impact
Emerging technologies have the power to revolutionize the study of double vibration. For example, advancements in sensor technology allow for more precise vibration measurement. Increased sensitivity and range contribute to deeper insights into complex vibrational interactions.
- Nanotechnology: This enables the creation of materials designed with specific vibrational properties. These materials can lead to innovations in industries such as aerospace and automotive.
- Smart Materials: Integration of smart materials capable of self-assessment of vibrational behavior can lead to improvements in structural health monitoring systems.
- Wearable Devices: The rise of wearable technology capable of monitoring vibrational data opens new avenues for research, particularly in the medical field.
The impact of these technologies extends beyond improved measurement. They allow for the collection of vast amounts of data, which in turn can inform theoretical models and practical applications.
Integration of Computational Methods
The role of computational methods in double vibration research cannot be overstated. Innovations in computational modeling contribute to a better understanding of complex systems.
- Finite Element Analysis: This technique provides detailed insights into vibrational behavior within structures, predicting potential failure points.
- Machine Learning: Incorporating machine learning algorithms enables researchers to analyze patterns in vibration data quickly. This can enhance the predictive power of models.
- Simulation Software: Tools like ANSYS and COMSOL Multiphysics allow for the simulation of various vibrational scenarios, improving design processes in engineering and beyond.
Through computational methods, researchers can explore scenarios that are impossible to recreate in a laboratory setting. This helps build a robust theoretical framework for double vibration principles.
"The future of double vibration research hinges on our ability to leverage new technologies and computational power to unravel complexity in vibrational phenomena."
As the field progresses, collaboration between disciplines will enhance understanding and spur innovation. These future directions in double vibration research underscore its importance in advancing technology and our grasp of complex systems.
The End
In concluding our exploration of double vibration, it is crucial to highlight its significance across various fields. This phenomenon forms an essential link between theoretical concepts and practical applications. Understanding the mechanisms behind double vibration aids in refining engineering practices, enhancing designs, and improving the durability of structures.
Summary of Key Points
The discussion throughout this article has pointed out several key elements:
- Definition and Mechanisms: Double vibration involves complex oscillatory behaviors influenced by multiple interacting systems.
- Types and Applications: Different forms of double vibration exist, each with unique properties and implications, especially in engineering and acoustics.
- Challenges: Numerous obstacles challenge researchers, including the complexity of real-world systems and limitations in theoretical modeling.
- Future Directions: Advancements in technology and computational methods hold promise for deeper understanding of double vibrations, leading to novel applications.
Each of these points serves to illustrate how double vibration is not just an abstract concept. Rather, it plays a vital role in contributing to our understanding and application of physical laws in various domains of science and engineering.
Implications for Future Research
Looking forward, the study of double vibration offers valuable avenues for research:
- Technological Integration: By merging traditional theories with cutting-edge technologies, researchers can develop innovative solutions.
- Cross-Disciplinary Approaches: The interdisciplinary nature of double vibration encourages collaboration between fields such as physics, engineering, and biology.
- Modeling Improvements: Future work can focus on refining models to better capture the real-world complexities of double vibration phenomena.
The importance of studying double vibration cannot be understated. As technologies continue to evolve, the lessons learned from this research will have implications not only in academic settings but also in practical, real-world applications.
As we advance our understanding of double vibration, we pave the way for more resilient structures, improved safety protocols, and enhanced technological innovations.