Understanding FACS Sorted Cells: An In-Depth Exploration
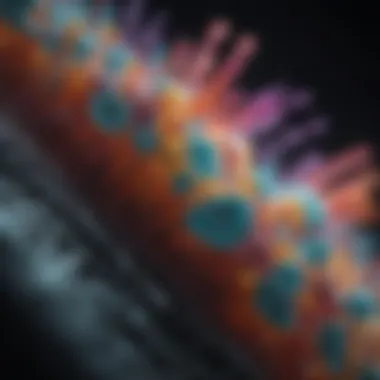
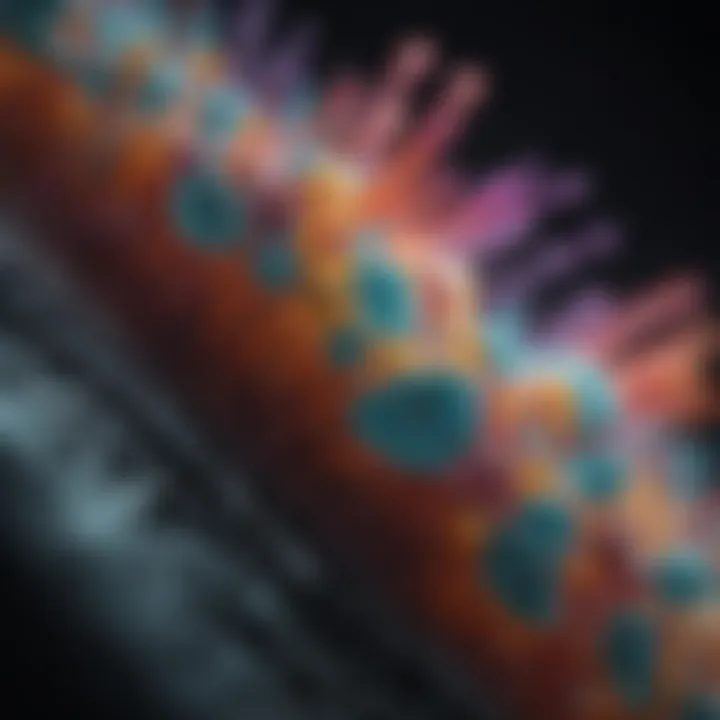
Intro
Fluorescence-activated cell sorting (FACS) is a sophisticated technique widely employed in biological research. It allows for the separation and isolation of specific cell populations based on their unique fluorescent characteristics. This approach has seen vast applications, especially in areas like immunology, cancer research, and stem cell biology. Understanding the intricacies of FACS not only sheds light on its operational principles but also highlights the numerous possibilities it offers researchers in the realm of cell biology.
Research Methodology
Description of Research Design and Approach
To effectively delve into FACS sorted cells, one must appreciate the methodology behind this technique. The research design involves a systematic approach to evaluate how FACS is conducted, determining the types of cells that can be sorted based on their fluorescent markers. Both in vitro and in vivo models are often utilized to explore the efficiency and efficacy of the sorting process.
Materials and Methods Used in the Study
The primary components of FACS include a flow cytometer, specific fluorescently labeled antibodies, and the cell samples being analyzed. The following materials are crucial:
- Flow Cytometer: This is the essential device used to detect and sort cells based on their light-scattering properties and fluorescence.
- Fluorescent Antibodies: These antibodies are tagged with fluorescent dyes to mark specific cell types, allowing for precise sorting during the process.
- Cell Samples: The samples can range from blood to tissue cultures, depending on the research focus.
The FACS process typically involves the following steps:
- Preparation of Cell Samples: Cells are first isolated and resuspended in a suitable buffer.
- Staining with Fluorescent Antibodies: Cells are incubated with antibodies, allowing them to bind to specific markers on the cell surface.
- Running the Samples through Flow Cytometer: The samples are suspended in a single-cell stream before being passed through the laser system of the flow cytometer.
- Sorting of Cells: Based on the fluorescence signal and physical characteristics of cells, the cytometer sorts and collects the target cell populations.
By employing these techniques, researchers can effectively isolate specific cells for further analysis or experimentation.
Discussion and Interpretation
Interpretation of Results in the Context of Existing Literature
The efficacy of FACS sorting has been well-documented in various studies. Research shows a high correlation between the fluorescent intensities of sorted cells and specific cell functions or states. This has significant implications in identifying cancerous cells, immune cell subtypes, or even stem cells. The ability to isolate these populations enhances the understanding of their roles in health and disease.
"FACS allows researchers to dissect and understand the complexity of cell populations in a quantitative manner, fostering discoveries across fields."
Implications for Future Research or Practical Applications
The future of FACS sorted cells looks promising, especially with advancements in technology. Improved fluorescent dyes and enhanced flow cytometry systems may lead to more accurate cell sorting and analysis. Additionally, the integration of artificial intelligence and machine learning could optimize sorting processes, offering quicker and more precise results.
In practical applications, the therapeutic potential is vast. FACS can facilitate targeted cell therapies, vaccine development, and deeper insights into immune responses. As researchers continue to explore FACS technology, the horizon for cell biology research and clinical applications broadens significantly.
Prelude to FACS
Fluorescence-Activated Cell Sorting (FACS) is an essential method in cell biology. This technique allows researchers to separate live cells based on their unique characteristics. The significance of FACS in the realm of scientific research cannot be overstated. It provides insight into the functional attributes of cells, assisting in various studies ranging from immunology to cancer research.
FACS technology is the bridge between basic science and applied clinical research. By isolating specific cell types, scientists can explore their roles in health and disease, making it easier to develop targeted therapies. This introduction will cover crucial aspects of FACS, laying the foundation for understanding its principles and applications.
Definition of FACS
FACS stands for Fluorescence-Activated Cell Sorting. It employs lasers and fluorescent markers to detect and sort cells. Cells are labeled with specific fluorochromes that emit light when excited by laser light. In essence, FACS uses light as the primary tool to discern differences among cells. The process involves passing cells through a focused laser beam. When a cell passes through, the system detects its fluorescence and sorts it based on predefined characteristics, such as size or internal structure. This definition underscores the utility of FACS in precisely isolating cell populations.
Historical Context
The development of FACS technology is rooted in broader advancements in flow cytometry. The first flow cytometer was created in the 1960s, but it was the 1970s that marked the turning point for FACS. Research teams began to utilize fluorescence to enhance cell identification. Notable advancements in lasers and fluorochrome technology contributed to the evolution of FACS. It played a pivotal role during the Human Immunodeficiency Virus studies in the 1980s. Since then, FACS has grown to become a standard technique in laboratories worldwide, expanding its applications in various fields drastically. The historical context illustrates the evolution of this powerful tool and highlights its impact on modern research methodologies.
FACS has transformed how researchers analyze and manipulate cells. Its ability to sort cells effectively has opened up numerous paths in both basic and applied science, underscoring the continued relevance and growth of this technique.
Principles of Fluorescence
Fluorescence is a fundamental aspect of fluorescence-activated cell sorting (FACS). Understanding this principle helps elucidate how specific cell populations are isolated based on their unique fluorescent properties. At its core, fluorescence involves the absorption of light by a molecule, followed by the re-emission of light at a longer wavelength. This property is crucial in FACS, enabling researchers to tag cells with fluorescent markers for accurate sorting. It is essential to grasp this concept to appreciate the potential applications and limitations inherent in FACS methodologies.
The importance of fluorescence in FACS lies not only in its theoretical foundation but also in practical applications. Fluorescent markers act as vital indicators within biological samples. Therefore, accurate knowledge of how fluorescence works provides insight into optimizing cell sorting techniques.
Understanding Fluorescence
Fluorescence occurs when a molecule transitions from a ground state to an excited state after absorbing light or other radiation. As the molecule returns to its ground state, it releases energy in the form of visible light. This light differs in wavelength from the absorbed radiation, allowing for the distinction between different light sources. The intensity and specificity of fluorescence emissions are crucial for the successful differentiation of various cell populations in a sample.
In FACS, specific wavelengths are selected to identify target cells. The efficient design of excitation lasers and detection systems is critical. For example, Argon-ion lasers can excite multiple fluorochromes simultaneously, enhancing the multiplexing capabilities of fluorescence applications.
Fluorochromes and Dyes
Fluorochromes, or fluorescent dyes, are chemical compounds that exhibit fluorescence. Their selection is fundamental in FACS. Various fluorochromes emit light across a spectrum, allowing researchers to label different cell types uniquely. Some common examples include fluorescein isothiocyanate (FITC) and phycoerythrin (PE). Each fluorochrome has unique spectral properties that guide its application in specific experimental contexts.
When designing a FACS experiment, one must consider several factors regarding the choice of fluorochromes:
- Spectral Overlap: Minimize overlap between fluorochrome emissions to prevent detection errors.
- Brightness: Select bright fluorochromes to increase signal detection.
- Photostability: Choose dyes that resist photobleaching, ensuring reliable results over time.
Using a combination of fluorochromes can increase the data obtained from each cell sample, allowing deeper insights into cellular behaviors. Hence, the expert selection and application of fluorochromes are vital for the successful implementation of FACS methodologies.
Understanding the principles of fluorescence is essential for optimizing FACS. The choice of fluorochromes directly affects the quality of the data produced.
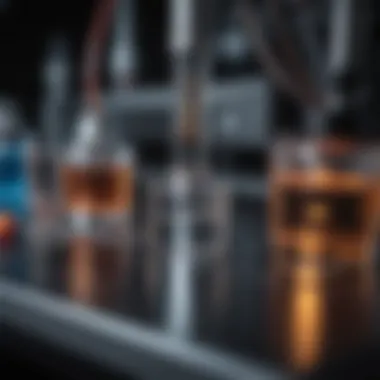
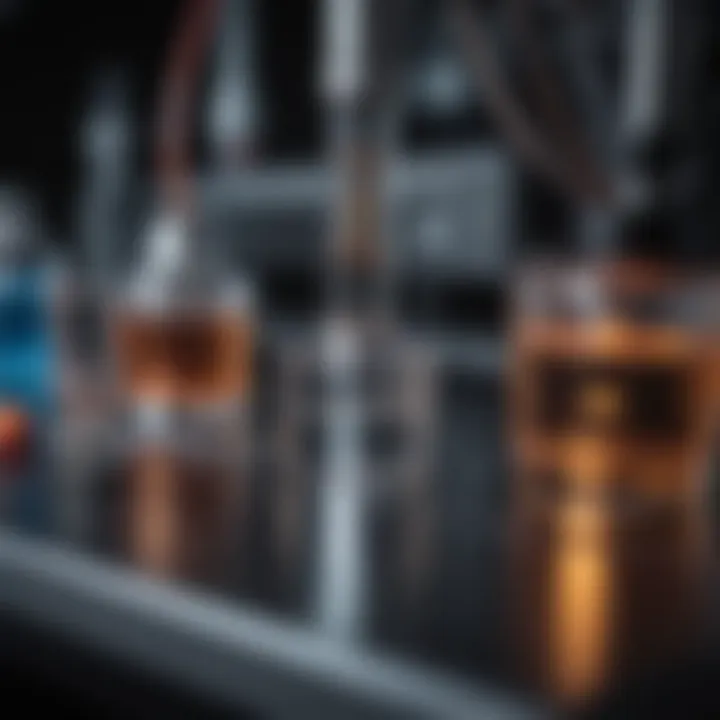
FACS Instrumentation
Fluorescence-activated cell sorting (FACS) relies heavily on instrumentation for its execution. The precise measurements taken by these devices are necessary for achieving reliable results. Multiple aspects of the technology exist that must be understood to fully appreciate its function and significance in cell sorting. Understanding the components, laser systems, and detection systems within FACS machines will clarify how these elements contribute to successful sorting processes.
Components of FACS Machines
FACS machines are composed of several critical components that enable their functionality. Among these parts are the fluidics system, lasers, and optics.
- Fluidics System: This system is responsible for transporting cells in a stream, ensuring each cell aligns precisely as it passes through the laser. A well-designed fluidic system minimizes cell clumping, important for acquiring accurate data.
- Lasers: Lasers excite fluorochromes in the cells, setting off fluorescence that is essential for sorting. The right type and quality of laser are vital.
- Optics System: This includes lenses and filters that collect the emitted light once excited by lasers. The optics system ensures proper detection of fluorescence.
- Data Acquisition System: This collects and processes the signals received from the detection system. It converts the identified fluorescence into valuable data, guiding the sorting procedure based on specific criteria.
Each component’s optimized design is crucial for enhancing the machine’s efficiency and the accuracy of sorting.
Laser Systems
Lasers are at the core of FACS technology. They are essential for the excitation of the fluorescent labels bound to the cells. Without efficient laser systems, the fundamental mechanism of FACS—detecting and sorting cells based on their fluorescence—would not function.
- Types of Lasers: Different types of lasers such as argon, helium-neon, and solid-state are used based on the fluorescence properties required. The selection ultimately influences the spectrum of fluorochromes that can be excited.
- Laser Wavelengths: Each laser operates at specific wavelengths, which must align with the absorption spectra of the chosen fluorochromes. This correspondence is critical for maximizing the emission of fluorescence.
- Stability and Efficiency: The quality of laser systems influences data productivity. A stable and efficient laser enhances fluorescence intensity and, in turn, facilitates better cell sorting.
Detection Systems
Detection systems in FACS determine the success of the entire sorting process. Once the cells are excited and fluorescence is emitted, the detection systems capture this light.
- Photomultiplier Tubes (PMTs): These devices convert light into an electric signal. PMTs are sensitive to low light levels, making them perfect for catching various fluorescence intensities.
- Avalanche Photodiodes (APDs): APDs are increasingly used as an alternative to PMTs due to their improved sensitivity and greater dynamic range. They perform particularly well under variable light levels.
- Digital Signal Processing: This step is vital as it converts and analyzes the electrical signals for accurate data representation. Proper signal processing ensures that the fluorescent data collected leads to meaningful conclusions about cell populations.
In summary, understanding these systems is key to harnessing FACS effectively. Each system has its specific challenges and advantages, requiring a deep knowledge base for successful operation. Moreover, attention to each component fosters innovation in operational techniques, advancing the field of cell sorting.
The FACS Sorting Process
FACS sorting is a pivotal element of cell biology that allows researchers to isolate specific populations of cells based on their unique characteristics. Understanding this process is vital for anyone interested in utilizing FACS technology for research or therapeutic applications. Within this section, we will address several significant components that make up the sorting process: sample preparation, sorting mechanisms, and data acquisition and analysis. Each of these aspects plays a crucial role in ensuring the validity and reliability of the results.
Sample Preparation
Sample preparation is one of the most critical steps in the FACS sorting process. Proper handling and treatment of samples can drastically affect the outcomes. Before sorting, cells must be adequately labeled with appropriate fluorochromes to allow for distinct identification under the flow cytometer.
- Cell Viability: Ensuring that the cells are viable is crucial. Dead cells can lead to inaccurate sorting and analysis. Methods such as trypan blue exclusion can be employed to assess cell health.
- Cell Density: An optimal cell concentration should be maintained. Too many cells may result in clumping, which can obstruct flow, while too few cells may hamper the statistical relevance of results.
- Buffer Selection: The choice of buffer can affect the stability of fluorescent labels and, ultimately, the quality of results. Phosphate-buffered saline (PBS) is commonly used due to its compatibility with various fluorochromes.
A well-prepared sample sets the foundation for an effective sorting process, influencing both efficiency and precision in subsequent steps.
Sorting Mechanisms
The sorting mechanisms utilized in FACS fundamentally determine how cells are isolated. In essence, FACS employs an intricate system that combines fluid dynamics, optics, and electronics to achieve precise sorting.
- Hydrodynamic Focusing: This technique streamlines cells into a single file through the application of fluid dynamics. This ensures that each cell passes through the laser beam one at a time, reducing the likelihood of collisions or misclassification.
- Laser Excitation: When the cells intersect with the laser, the fluorescent dyes emit light at specific wavelengths. This emitted light is detected and analyzed to determine the characteristics of each cell.
- Deflection Plates: Based on the detected fluorescent characteristics, cells are charged and directed into their respective collection tubes. This accurate sorting process enables researchers to isolate populations with minimal cross-contamination.
Sorting mechanisms are essential for effective FACS, as they provide the technological framework for separating cells based on fluorescence intensity and light scattering properties.
Data Acquisition and Analysis
The final aspect of the FACS sorting process involves data acquisition and analysis. This step is crucial for interpreting the sorted populations and understanding the implications of the findings.
- Acquisition Software: Modern FACS instruments come equipped with sophisticated software that manages data collection. This software allows for multi-parameter analysis, enabling the examination of several cellular characteristics simultaneously.
- Data Interpretation: After acquisition, the data must be analyzed statistically. Various parameters, such as mean fluorescence intensity and population frequency, need to be evaluated carefully. Statistical software may be applied to quantify changes and identify cell subsets.
- Visualization Tools: Graphical representations such as histograms and dot plots are often generated to facilitate interpretation. They help in visualizing the distribution of cell populations and the effects of various experimental conditions.
Data acquisition and analysis not only guide the sorting but also provide insights that inform subsequent research directions and hypothesis generation.
"In the realm of FACS, every detail in the sorting process enhances the overall quality and reliability of the research outcomes."
In summary, the FACS sorting process is pivotal for deriving accurate and meaningful research results. Each step, from sample preparation through sorting mechanisms and data analysis, requires meticulous attention to detail to ensure the successful application of FACS technology.
Applications of FACS
The applications of fluorescence-activated cell sorting (FACS) are numerous and impact a variety of fields. As a sophisticated method of isolating specific cell populations, FACS allows researchers to study cells in a more meaningful way. This capability has led to substantial advancements in immunology, cancer research, stem cell research, and gene therapy, among others. Understanding these applications is essential for appreciating the full potential of FACS technology. Each application not only showcases the versatility of this technique but also emphasizes its significance in solving complex biological questions.
Immunology Applications
In immunology, FACS serves as a key tool to analyze and manipulate immune cell populations. Scientists utilize this technique to identify different immune cell types based on surface markers, aiding in the understanding of immune responses. Specifically, the ability to isolate T-cells, B-cells, and antigen-presenting cells has transformed our understanding of various diseases and immune disorders.
Moreover, FACS allows for the evaluation of cytokine production and other functional assays, providing insights into how these cells respond under different conditions. Clinically, FACS is critical in developing immunotherapies, as it helps to identify target cell populations for treatments like monoclonal antibodies and CAR-T cell therapies.
Cancer Research
Cancer research benefits significantly from FACS technology, particularly in the characterization of tumor microenvironments. The ability to sort and analyze heterogeneous cell populations within tumors leads to better understanding of cancer biology. Researchers can isolate cancer stem cells, which may demonstrate resistance to therapies, providing insights into relapse and metastasis.
FACS is also used to assess the expression of biomarkers, facilitating diagnostics and treatment strategies. The technique aids in patient stratification for clinical trials, ensuring tailored therapies based on the specific cell populations present.
Stem Cell Research
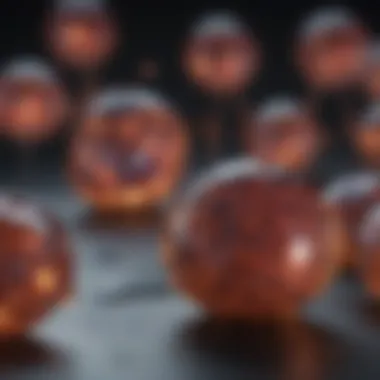
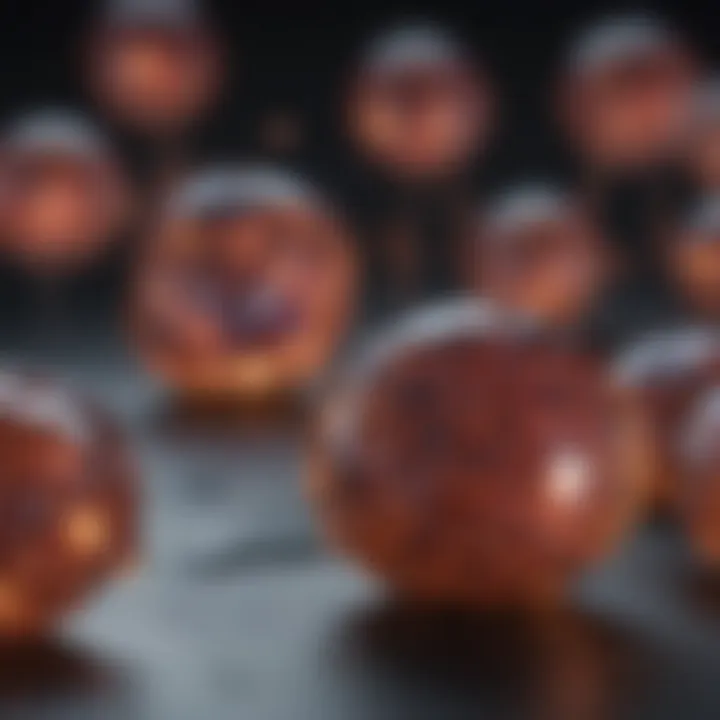
FACS plays a vital role in stem cell research by enabling the isolation of specific stem cell types. It allows researchers to purify populations of embryonic stem cells or adult stem cells based on distinctive surface markers. This isolation is critical for studying differentiation pathways and understanding the mechanisms that govern stem cell behavior.
Additionally, FACS assists in evaluating the potential of stem cells for therapeutic applications. By monitoring cell surface markers during differentiation, scientists can ensure they are generating the desired cell types for regenerative medicine or tissue engineering.
Gene Therapy and Genetic Engineering
In the realm of gene therapy and genetic engineering, FACS is utilized to sort genetically modified cells. This sorting capability is essential for ensuring that only cells with the desired genetic modifications are used in experiments or clinical applications. For example, in gene therapy, FACS can help isolate transduced cells that express a therapeutic gene, enhancing the efficacy of treatments.
Furthermore, FACS supports the assessment of gene expression and the efficiency of genetic modifications. The ability to sort cells based on reporter constructs or surface markers makes it easier to analyze the outcomes of various genetic interventions.
The applications of FACS extend beyond what is currently known, continually evolving with advances in technology and biological understanding.
Challenges and Limitations
Understanding the challenges and limitations of fluorescence-activated cell sorting (FACS) is crucial for researchers and practitioners. While FACS is an extremely valuable tool in cellular biology, it is not without its drawbacks. Addressing technical shortcomings and biological variability allows for improved methodologies and results in research settings. Researchers must be cognizant of these factors to optimize their experiments and interpret data correctly.
Technical Limitations
Technical limitations in FACS primarily relate to the equipment itself and the protocols employed. Some common issues include:
- Sensitivity and Resolution: The performance of FACS may be affected by the sensitivity of detectors. If the detection systems are not calibrated properly, small populations of cells may go unnoticed. This lack of sensitivity can diminish the accuracy of data, particularly when analyzing rare cell types.
- Throughput Limitations: While modern FACS machines can process thousands of cells per second, there remain scenarios where the speed is a bottleneck. For large sample sizes, extended sorting times can lead to sample degradation, posing a risk to viability and functionality of sorted cells.
- Complexity of Protocols: FACS protocols can be intricate. Errors in sample preparation or staining can lead to misinterpretation of data. Ensuring adherence to strict quality control protocols is essential, yet it also increases operational complexity.
It’s essential to consider that advances in technology continually aim to mitigate these limitations. However, awareness of these constraints helps inform better practices and experiment designs in a lab setting.
Biological Variability
Biological variability presents a unique layer of challenges in FACS applications. This variability can arise from multiple sources, including:
- Genetic Diversity: Different cell populations can exhibit significant genetic differences that affect their behavior and fluorescent characteristics. When sorting, phenotypic variations can result in unexpected sorting outcomes, potentially affecting the experimental results.
- Environmental Factors: Conditions in which cells are grown or stored, such as temperature and medium composition, may influence their functionality. Changes in such parameters can lead to variability in sorting performance, making it difficult to reproduce results.
- Cell Cycle Dynamics: Cells in different stages of the cell cycle can exhibit different properties, impacting fluorescence. This variability can skew results if not properly accounted for during sorting.
Overall, understanding these biological factors is key to interpreting results effectively. It necessitates a careful approach to experimental design and execution to minimize their impacts.
"Awareness of challenges and limitations fosters a more profound understanding of experimental outcomes and guides better methodologies."
By recognizing both technical limitations and biological variability, researchers can enhance their application of FACS technology. Continued development in the field aims to address these issues while promoting a more reliable and robust understanding of cellular populations.
Future Directions in FACS Technology
Fluorescence-activated cell sorting (FACS) is a dynamic field that is continually evolving. The future directions in FACS technology will not only enhance the efficiency of sorting but also broaden the scope of applications. Staying abreast of these advancements is essential for researchers, as the implications can directly influence experimental outcomes and overall understanding of cell biology.
Advancements in Fluorochrome Development
The evolution of fluorochromes plays a considerable role in the future of FACS technology. New fluorochromes are being developed with improved photostability, brightness, and specific binding characteristics. These enhancements mean researchers can explore more complex cellular environments with greater precision.
For example, innovative fluorochromes can allow for multicolor labeling. This process gives the ability to analyze multiple cell surface markers concurrently, leading to more detailed cellular profiling. As these compounds become available, they not only increase the information yield from a single experiment but also reduce the sample processing time.
Moreover, ongoing research aims at the creation of fluorochromes that are sensitive to specific cellular events, such as pH changes or binding affinity alterations. These targeted markers could open avenues for studying dynamic processes within living cells, offering insights that were previously impossible to capture.
Integration with Other Technologies
The integration of FACS with other technologies is critical for maximizing its capabilities. For instance, coupling FACS with genomic and proteomic profiling technologies enables a multifaceted approach to cellular analysis. This combination allows for a comprehensive understanding of cellular function and behavior.
One notable example is the integration of FACS with next-generation sequencing (NGS). This pairing provides the opportunity to analyze sorted cells at both the transcriptomic and genomic levels. Researchers can sort specific cell populations and immediately analyze their genetic material, thus linking phenotypic data with genomic insights.
Additionally, combining FACS with imaging technologies, such as confocal microscopy, can offer a synchronized view of cellular morphology and molecular characteristics. This synergy allows for a thorough analysis of the spatial distribution of biomarkers within tissues.
In summary, the future directions in FACS technology are bright, with advancements in fluorochrome development and the integration with other cutting-edge technologies heralding a new era for research in cellular biology. As these improvements take hold, the reach of FACS will expand into new realms, enhancing our capability to dissect the complexity of life at the cellular level.
The evolution of FACS technology signifies a robust pathway for breakthroughs in multiple biological disciplines.
For more insights refer to Wikipedia or Britannica.
Case Studies
Case studies serve as vital references in the exploration of FACS sorted cells. They illustrate real-world applications of the FACS technique and help researchers understand its significance in various fields like immunology and oncology. By examining specific instances, one can observe both the successes and challenges faced during the implementation of FACS. This fosters a deeper understanding of how theoretical principles translate into practical applications.
Case Study in Immunology
In immunology, case studies highlight the utility of FACS in identifying and isolating specific immune cell populations. One notable instance involved researchers examining the role of regulatory T cells in autoimmune diseases. By using FACS, they could isolate these cells based on their unique surface markers and analyze their functionality in vitro.
The process began with the collection of blood samples from patients. The researchers labeled the cells with fluorescent antibodies targeting specific proteins on the surface of regulatory T cells. This allowed the differentiation of these cells from other immune cell types. The FACS machine then sorted out the targeted cells, providing isolated populations necessary for further study.
Benefits of utilizing FACS in such studies include:
- Enhanced precision in identifying rare cell populations
- Ability to perform functional assays on isolated cells
- Facilitation of longitudinal studies by tracking specific cell types over time
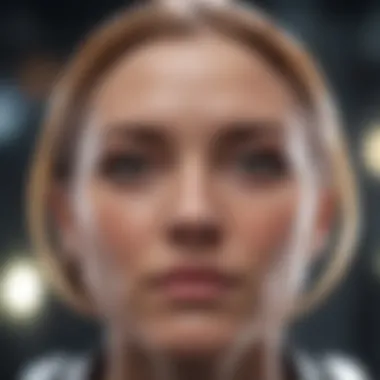
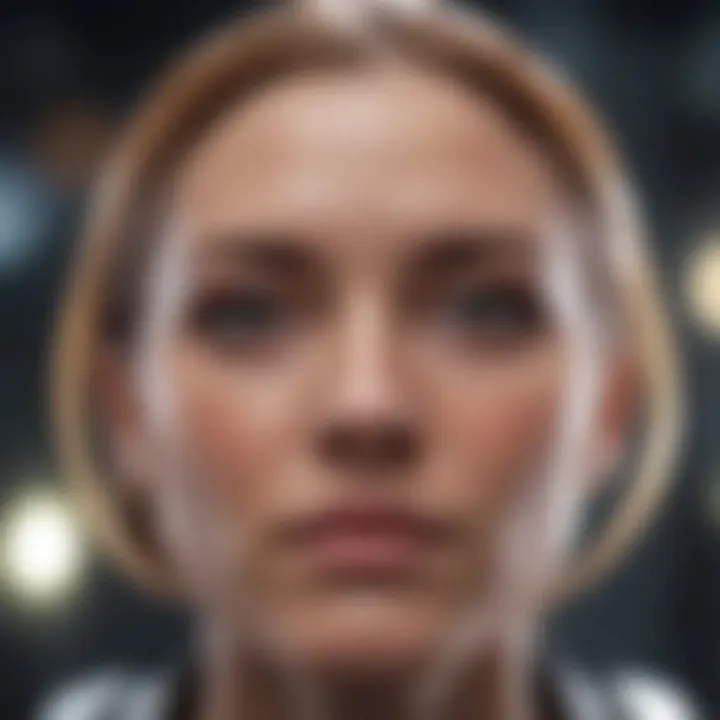
However, there are considerations too. The results must be interpreted with caution due to potential biological variability among samples. Moreover, the technical limitations of FACS equipment can affect the reproducibility of the findings.
Case Study in Cancer Research
In the realm of cancer research, FACS has been pivotal in identifying and isolating cancer stem cells. A significant study focused on breast cancer stem cells and their role in tumorigenesis. Researchers utilized FACS to isolate cells expressing particular markers associated with stemness.
They performed a series of experiments where tumors were dissociated to obtain a single-cell suspension. Specific fluorochrome-labeled antibodies were used for surface marker recognition, allowing for efficient sorting based on expression levels. This enabled the identification of a subpopulation of cells with self-renewal and tumorigenic capabilities.
The advantages of using FACS in this context include:
- The ability to isolate rare and functionally distinct populations
- Insight into cellular mechanisms behind tumor recurrence
- Comparison of stem cell populations from different tumor stages for effective therapeutic strategies
However, researchers in this area must acknowledge several limitations. For instance, not all markers are specific to cancer stem cells, leading to potential misclassification. Additionally, the complexity of cancer biology can challenge the interpretation of sorted populations.
Thus, these case studies illustrate the profound impact of FACS technology in both immunology and cancer research, while also underscoring the need for careful consideration of its limitations.
Best Practices for FACS Sorting
Fluorescence-Activated Cell Sorting (FACS) is a powerful tool in cell biology, enabling researchers to isolate and analyze specific cell populations based on their fluorescent characteristics. Yet, tapping into its full potential requires adherence to best practices, which ensure accuracy and reproducibility in results. This section outlines the key elements that enhance the effectiveness of FACS sorting, discussing the importance of optimizing sample handling and implementing robust quality control measures.
Optimizing Sample Handling
Sample handling can significantly affect the outcome of FACS sorting. Proper techniques in this area increase the chances of obtaining high-quality isolations. There are a few important aspects to consider:
- Cell Preparation: Cells must be prepared in a way that maintains their physiology. This includes minimizing cell death and stress during the preparation process.
- Dilution Factor: Use an appropriate dilution of cells. Highly concentrated samples can lead to clogging and reduced sorting efficiency. Generally, diluting to a concentration of about 1-10 million cells per milliliter is advisable.
- Buffer Selection: Choose a suitable buffer that maintains cell viability and prevents aggregation. Common buffers include phosphate-buffered saline (PBS) or specialized staining buffers.
- Staining Protocols: Follow specific staining protocols to ensure that fluorochrome-conjugated antibodies adequately label the target cell population without excessive background noise.
By optimizing these factors, researchers can improve cell recovery rates, which is critical for downstream applications.
Quality Control Measures
Quality control in FACS sorting safeguards the integrity of the data produced. Several measures help ensure the reliability and validity of FACS results. Some critical components include:
- Regular Calibration: Regular calibration of the FACS machine increases accuracy. This ensures that the lasers and detection systems function at optimal performance.
- Control Samples: Include positive and negative control samples during sorting. These controls allow for assessments of sorting efficiency and help identify potential issues in the sorting process.
- Monitoring Flow Rates: Maintain consistent flow rates to avoid fluctuations in sample delivery. Uneven flow can lead to cell clumping or inadequate sorting of distinct populations.
"Quality control is not just a procedure, but a principle of scientific integrity that underpins reliable research."
- Documentation: Keep precise records of sorting parameters and outcomes. This documentation is invaluable for troubleshooting and for future experiments.
Ethical Considerations
Ethics play a critical role in the realm of scientific research, particularly in areas involving biological materials such as cell sorting. Understanding the ethical considerations linked to fluorescence-activated cell sorting (FACS) is paramount as it directly influences the integrity of research outcomes and societal trust. This section will explore the ethical implications of cell sorting research, providing insight into the standards that govern practice in this field.
Ethics in Cell Sorting Research
When conducting research that involves FACS, several ethical issues must be taken into account. The most prominent of these involves informed consent. Researchers must ensure that biological samples, including human cells, are collected with the explicit permission of the donors. This process requires informing donors about how their cells will be used, potential risks, and benefits associated with the research. Additionally, maintaining the privacy and confidentiality of the data derived from these samples is essential. Failure to adhere to these ethical standards can lead to mistrust in the research community.
Another ethical aspect to consider is the potential misuse of sorted cells. FACS technology can be used to isolate specific cell types that may have implications in gene therapies or stem cell research. Therefore, researchers must contemplate the long-term consequences of their work, ensuring that it is directed toward beneficial applications and does not contribute to harmful practices. Researchers have a responsibility to consider how their discoveries could be misapplied or weaponized against vulnerable populations.
Furthermore, the regular review and update of ethical guidelines are necessary as FACS technology evolves. Staying informed of emerging ethical dilemmas is crucial for sustaining ethical research practices. By discussing these considerations openly within the scientific community, researchers can better navigate the ethical landscape of their work.
Implications for Human Studies
The ethical implications of FACS are particularly pronounced when human subjects are involved. With advancements in medical research and diagnostic methods, the capacity for manipulating human tissues and cells introduces complexities that merit caution. In the context of human studies, ethical considerations extend beyond mere compliance with existing regulations.
For instance, the potential for discrimination arises if specific cellular traits become associated with certain populations. This concern necessitates careful thought about how sorted cells are utilized in both research and clinical settings. Adopting a framework that promotes fairness and equality is key to addressing this challenge.
Moreover, when investigating human-related diseases, ensuring that the benefits of research are disseminated equitably among all population groups is essential. Ethical frameworks should actively combat biases that could lead to underrepresentation of certain demographics in studies involving FACS technology.
Ultimately, addressing ethical challenges in FACS research contributes to the field's credibility and acceptance. It lays the groundwork for informed discussions on the societal implications of biotechnological advancements, ensuring that scientific endeavors resonate positively with public sentiment.
"Ethics in research is about maintaining a balance between innovation and societal responsibility, guiding researchers to act within a framework of integrity."
By prioritizing ethical considerations, researchers can not only enhance the quality of their work but also foster a culture of responsibility that prevails in the scientific community.
The End
The conclusion of this article serves as a vital component in reinforcing the importance of fluorescence-activated cell sorting (FACS) and its multifaceted applications across various scientific disciplines. As we have explored, FACS is not merely a technique; it is an indispensable tool that shapes modern research methodologies in fields such as immunology, cancer research, and beyond. By systematically isolating specific cell populations, researchers gain the ability to study these entities in detail, allowing for a deeper understanding of cellular mechanisms and interactions.
In this review, we analyzed key points regarding the principles of FACS, the intricate sorting process, and diverse applications. Each section unveiled how this technology opens new avenues for research, facilitating breakthroughs that would be challenging without FACS. Furthermore, we highlighted best practices and ethical considerations, ensuring a responsible approach to cell sorting.
Summary of Key Points
- Definition and Functionality: FACS separates cells based on their fluorescent characteristics, enabling targeted analysis.
- Instrumentation Insights: A thorough understanding of the components, including lasers and detection systems, enhances the effectiveness of FACS.
- Applications Across Fields: FACS has transformative impacts on various areas including immunology and cancer research, driving forward our understanding of disease.
- Challenges and Limitations: Recognizing the technical limitations and biological variability aids in optimizing research outcomes.
- Ethical Considerations: Awareness of the ethical implications is critical in the responsible use of FACS.
Future Implications for Research
The future of FACS technology appears promising, with advancements poised to enhance its effectiveness and application scope. Research is steering toward integrating FACS with other innovative technologies, such as CRISPR and next-generation sequencing. This integration could offer synergistic benefits, allowing for the simultaneous analysis of genetic material and cellular phenotypes.
Moreover, continued development in fluorochrome refinement will likely improve sorting accuracy and reduce background noise, leading to clearer data interpretation. As researchers seek to overcome existing limitations, the expansion of FACS capabilities is anticipated, leading to novel insights in cellular biology.
"The power of FACS lies not only in its sorting capabilities but in its ability to uncover the subtleties of cellular behavior, shaping the future of biomedical research."