Understanding Intron DNA: Its Role and Importance

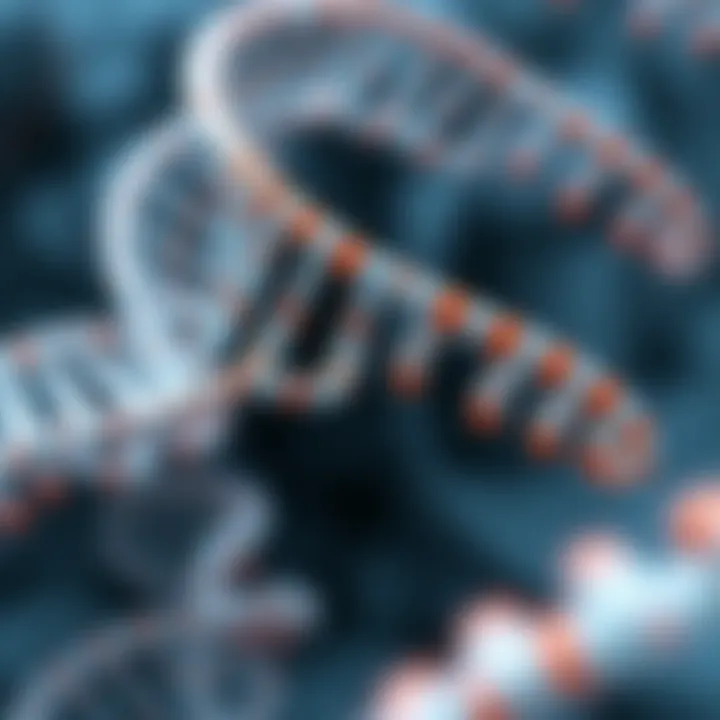
Intro
In the vast realm of genetics, an often overlooked yet crucial component resides in the non-coding regions of our DNA, known as introns. These segments, once dubbed ‘junk DNA,’ have showcased their significance, it’s time we unravel the layers that comprise their intricate roles. While we stand on the threshold of understanding how these seemingly silent players can impact gene expression and evolution, their contributions to the genomic landscape unfold in fascinating ways.
To get a clearer picture of how introns function and what roles they play across diverse organisms, we must delve deep into their structure and significance. From facilitating alternative splicing to regulating gene expression, intron DNA serves as a key player in biological complexity. This discourse aims not only to highlight the intricate dynamics of introns, but also to explore their implications in molecular biology and biotechnology.
As we traverse this fascinating topic, the following key points will be explored:
- The structure and function of intron DNA.
- The evolutionary significance of introns.
- Their role in alternative splicing and gene regulation.
- Applications for introns in modern biotechnology.
This exploration invites students, researchers, educators, and professionals alike to engage with a subject that stands as a testament to nature’s elaborate genomic fabric.
Intro to Intron DNA
Understanding intron DNA is akin to opening a window into the intricate workings of genetics and molecular biology. These segments within the genes, which do not code for proteins, have long puzzled scientists. However, recent research has underscored their potential significance. They serve roles beyond what was once believed, impacting gene regulation, cellular mechanisms, and evolutionary processes. By delving into the concept of introns, we can appreciate their complexity and wonder about the natural world’s design.
Definition and Basic Concepts
An intron is defined as a non-coding section of a gene, strategically sandwiched between coding sequences known as exons. These sequences are transcribed into RNA but are spliced out during the formation of messenger RNA (mRNA), thereby leaving only the exons. This process is vital for the production of functional proteins and enables cells to diversify their protein output via alternative splicing.
To grasp the essence of intron DNA, one must recognize a few foundational concepts:
- Intron and Exon Dynamics: The interplay between exons and introns determines the ultimate structure of proteins.
- Alternative Splicing: This dance of cutting and joining allows a single gene to give rise to multiple proteins, expanding the toolkit a cell can use.
- Evolutionary Perspective: The presence of introns in many organisms suggests their evolutionary advantages, but the full scope of their function is still a puzzle waiting to be pieced together.
Overall, the study of introns is crucial for a deeper understanding of genetics, and their mere existence suggests a more sophisticated regulatory framework than previously conceived.
Historical Perspective
Historically, introns were viewed as genetic junk—leftover remnants from an evolutionary past. This viewpoint began to shift in the 1970s, when researchers uncovered the significance of introns in determining gene expression and protein variability. Figures like Richard J. Roberts and Phillip A. Sharp unlocked critical insights into intron splicing, paving the way for modern molecular biology.
This shift in perception caused a domino effect:
- Recognition of Complexity: Introns were recognized as essential players, not mere bystanders in the genomic landscape.
- Genomic Research Expansion: The advent of genetic mapping and sequencing technologies has led to introns becoming a focus for evolutionary biologists and geneticists alike.
- Emergence of New Tools: Advancements in CRISPR technology provided avenues to manipulate introns, revealing their potential in gene therapy and biotechnology.
In essence, the shifting perspective on introns reflects broader trends in biology—from a simplistic view of genes as linear blueprints to acknowledging a dynamic, intricate interplay of cellular components in genetic regulation.
Intron Structure and Characteristics
Understanding intron structure and characteristics is crucial for grasping their role in molecular biology. These segments are not just gaps in the coding sequence; they are vital pieces of the genetic puzzle, influencing how genes are expressed and regulated.
Nucleotide Composition
Introns are composed of nucleotides, which are the building blocks of DNA. Typically, they are made of the same four nucleotides—adenine, thymine, cytosine, and guanine—as exons. However, the sequence and arrangement of these nucleotides can greatly differ across species. The specific nucleotide composition can direct where introns are found in a gene, and this positioning matters when it comes to splicing.
Moreover, some introns contain sequences that aid in the splicing process itself, highlighting their dual functionality. These splicing signals can vary not just in their makeup, but also in their length, which affects the efficiency of splicing. In a way, the intricate nature of nucleotide composition can be likened to the varied tones of a symphony; while each note is essential, how they are arranged contributes to the overall harmony.
Intron-Exon Organization
The organization of introns and exons within a gene is like the chapters and paragraphs of a book; it defines how the genetic story unfolds. Eukaryotic genes often contain multiple exons interrupted by one or more introns. The sizes and numbers of these components are not uniform across organisms or even within species, highlighting a level of complexity that can be puzzling.
Introns may be considered as the editing rooms of a gene, where unnecessary sequences are removed to create the final product that codes for proteins.
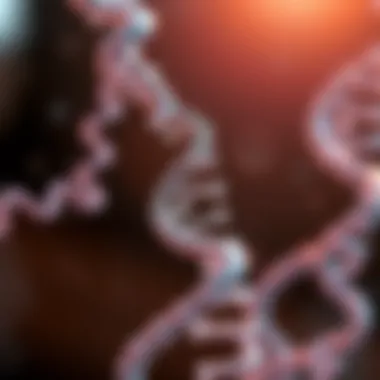
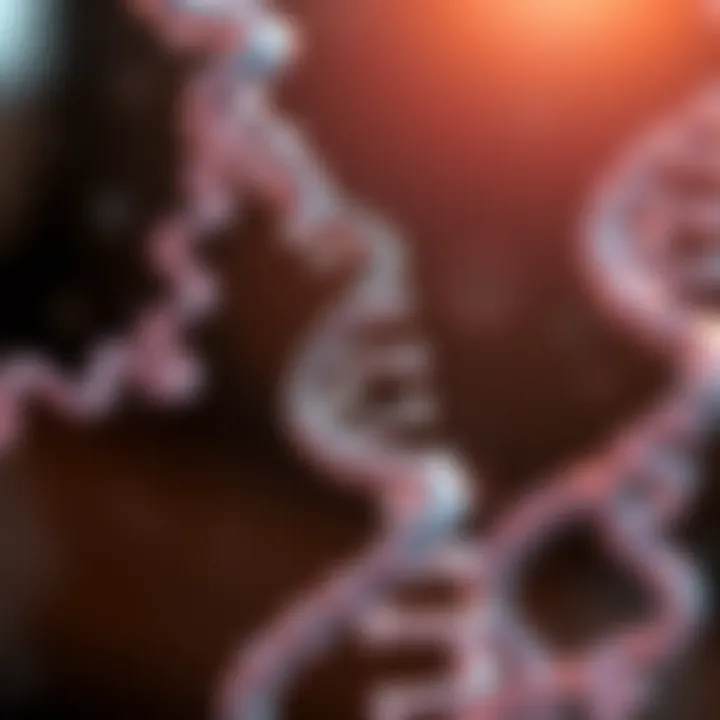
This structure plays a pivotal role in alternative splicing, a process through which a single gene can produce multiple proteins. Various combinations of exons can be spliced together, leading to a diverse range of proteins from a single DNA sequence. This flexibility can be particularly useful in responding to different environmental conditions or developmental stages, maximizing the utility of a limited number of genes.
Variability Across Organisms
The variability of intron structure across different organisms is another fascinating aspect. In plants, for instance, introns can be larger and more abundant compared to those found in animals. Conversely, certain prokaryotic organisms exhibit almost no introns; they tend to streamline their genetic material for efficiency rather than versatility.
This variability poses questions about evolutionary adaptation.
- Higher Plants: Have introns that can range from hundreds to thousands of base pairs, possibly contributing to their complex regulatory mechanisms.
- Animals: Generally possess a moderate number of introns but with intricate regulatory roles in gene expression.
- Prokaryotes: Often lack introns altogether, signifying a different evolutionary pathway focused on rapid reproduction and less regulatory complexity.
In summary, exploring the organization and variability of introns offers insights into evolutionary strategies, gene function, and the intricate tapestry that makes up an organism's genome. By appreciating these elements, researchers can better understand how life on Earth has adapted and flourished.
Functional Roles of Introns
Introns, often viewed as mere gaps within genes, actually play a multifaceted role in gene expression and regulation. Their functional significance reaches far beyond simple placeholders, providing essential benefits across various biological processes. Understanding the roles of introns sheds light on their contributions to the intricate dance of genetic machinery.
Alternative Splicing Mechanisms
At the heart of intron functionality lies alternative splicing, a process that allows for the generation of diverse protein isoforms from a single gene. When a pre-mRNA transcript is formed, it contains both introns and exons. By selectively retaining or removing different exons—thanks to the presence of introns—cells can produce various proteins in response to specific developmental cues or environmental stresses. This flexibility provides cells with a powerful means to adapt.
For instance, consider a gene encoding a protein involved in muscle development. Through alternative splicing, one could create proteins assisting in different aspects of muscle function based on the tissue type or developmental stage. This diversifies the functional repertoire of proteins available to cells without the need for additional genes.
"Alternative splicing is a key factor impacting functional diversity in the proteome, allowing a single gene to contribute to many outcomes."
Regulation of Gene Expression
Introns also have a role in the regulation of gene expression. They harbor various regulatory elements that can interact with transcription factors, influencing when and how much a gene is expressed. Certain introns can enhance gene activity through mechanisms like the strengthening of promoter regions, thereby elevating transcriptional levels.
The presence of introns can also mediate the effects of non-coding RNAs, such as microRNAs, which can bind within intronic sequences. By doing so, they can inhibit or promote gene expression, further complicating the picture of how genes are regulated. This interaction hints at a deeper layer of genetic control that reflects the biological complexity of an organism.
Impact on Protein Diversity
When discussing the impact on protein diversity, one must appreciate that introns serve as more than just passive parts of the genetic code. They contribute to the evolution of protein functions by allowing the mixing and matching of domains through recombination events and alternative splicing. A significant amount of the human proteome arises from this very process.
As specifics fluctuate through evolutionary timelines, characteristics such as domain shuffling become crucial in generating new protein functions. In essence, introns are instrumental in enabling organisms to develop novel traits fitted to particular environments.
In summary, introns are not excess baggage within genes but essential players in the realm of molecular biology. Their contribution to alternative splicing, regulation of gene expression, and the diversification of proteins underscores their value in genetic architecture. As research continues, understanding these mechanisms may illuminate more about evolution and the complexity of life.
Evolutionary Significance of Intron DNA
The evolutionary significance of intron DNA cannot be overstated. They are not merely interruptions in the genetic code; rather, these seemingly redundant segments play a critical role in shaping the genomic narrative of all living organisms. To grasp their importance, one must delve into how introns can influence not just individual genes but also entire evolutionary trajectories.
Intron Evolution Theories
There are several theories surrounding the evolution of introns, each painting a different picture of their developmental timeline. One prominent theory suggests that introns were once present in all genes and that their gradual loss in some organisms, particularly prokaryotes, has contributed to their streamlined genomes. However, this doesn't mean that introns are just leftover DNA. Their presence can confer evolutionary advantages, such as facilitating the alternative splicing mechanism, which allows one gene to produce multiple proteins. This adaptability can increase the functional diversity of proteins in organisms.
It's also worth mentioning the "intron early" versus "intron late" hypothesis. The former suggests that introns were present in the earliest forms of life and that they were subsequently lost through evolution, while the latter posits that introns arose later as organisms became more complex. Both theories underscore the intron's role as a crucial genetic player.
"Introns are not just gaps; they are bridges connecting genetic evolution with functional diversity."
Comparative Genomics Insights
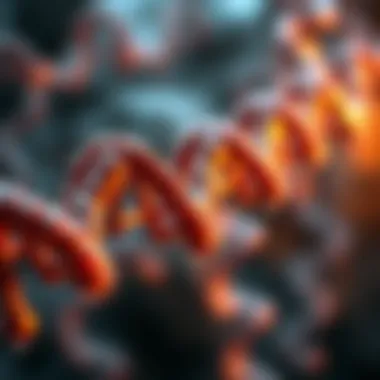
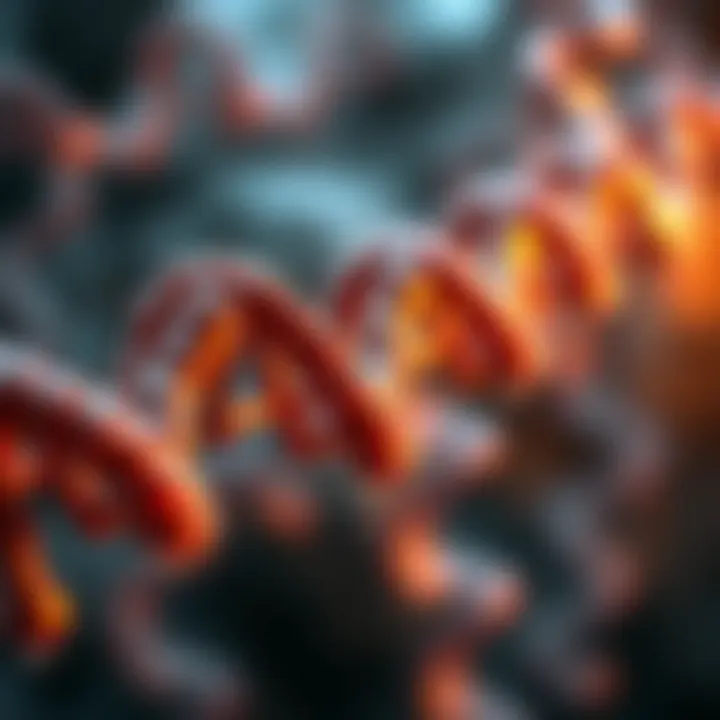
Comparative genomics offers valuable insights into the significance of introns, revealing patterns across different life forms. By analyzing genomes from various species, researchers can observe how introns differ in number, size, and distribution. For instance, humans possess approximately 200,000 introns compared to a simpler organism like E. coli, which has very few. This disparity hints at a correlation between intron counts and the complexity of an organism, suggesting that more introns may allow for greater regulatory capabilities and versatility in protein expression.
Moreover, studies have shown that the presence and conservation of introns across distantly related species can indicate evolutionary relationships. These conserved regions may have played critical roles in maintaining essential functions throughout evolution, offering clues about not just the past but potential pathways for future evolution.
In summary, the evolutionary significance of intron DNA touches on complex theories and empirical evidence. From insights gleaned through comparative genomics to the functional advantages they confer, introns are key players in the grand game of life's evolution. These insights not only enhance our understanding of genetics but also lay the groundwork for advances in fields such as biotechnology and medicine.
Intron DNA in Various Organisms
The study of intron DNA across different life forms reveals not just a rich tapestry of genomic architecture, but also underscores the diverse roles these elements play in various biological processes. Understanding how introns function in distinct organisms can illuminate the evolutionary significance of these pieces of genetic material and their contributions to complexity in cellular systems. Introns are not mere genetic noise; they are significant players that shape gene expression and protein diversity within organisms.
Intron Function in Eukaryotes
In eukaryotes, introns serve multiple important functions pivotal to gene expression. Primarily, they participate in alternative splicing, which allows a single gene to produce multiple proteins by varying the combinations of exons included in the final mRNA transcript. By this mechanism, organisms can increase protein diversity without the genomic burden of having numerous genes.
Moreover, introns may also harbor regulatory elements that fine-tune when and how genes are expressed. For example, certain intronic sequences can interact with various proteins to facilitate enhanced transcription or stabilization of mRNA. This regulatory role is essential, particularly in response to environmental stimuli, where genes may need to be activated or silenced quickly. Understanding these functions is beneficial for areas such as medical research, where gene expression patterns can indicate disease states.
Prokaryotic Introns: An Exception
When it comes to prokaryotes, the picture differs markedly. Historically, it was thought that introns were rare in prokaryotic genomes. However, some bacteria, like Archaeal species, do possess introns. Unlike their eukaryotic counterparts, prokaryotic introns mainly exist within rRNA and tRNA genes, and they often follow a different splicing process, known as self-splicing. This characteristic distinct sets prokaryotic introns apart as a curiosity rather than a complex regulatory feature.
The existence of introns in prokaryotes hints at a potentially underestimated role in bacterial evolution and adaptation. Some studies suggest that these intron-containing systems could provide mechanisms for rapid variation and plasticity in responses to environmental changes. So while prokaryotic introns are few and far between, their presence still raises intriguing questions regarding the overarching evolutionary narrative of intron DNA.
Fungi and their Unique Intron Patterns
Fungi represent another fascinating realm of intron studies. Unlike plants and animals, fungi showcase a diverse pattern of intron presence and absence. Certain fungal species, such as Neurospora crassa, have been shown to have introns that function similarly to those in eukaryotes, with implications for gene regulation and mRNA processing. In fact, the Neurospora system has been frequently used as a model for understanding intron dynamics.
Interestingly, the function of introns in fungi can also involve complex interactions with other cellular processes. Their varied patterns suggest a potential evolutionary pressure or need for flexibility in gene expression tied to their ecological niches. Moreover, the unique mechanisms by which some fungi also can splice introns, even when they diverge significantly from eukaryotic processes, makes them informative subjects for understanding the functional versatility of introns.
"The role of introns across different biological kingdoms reveals not just variability but essential adaptivity in genetic expression systems."
Technological Applications of Intron Research
In recent decades, intron research has blossomed, revealing various technological applications that are shaping genetics and beyond. The significance of introns extends well beyond their presence in genomic sequences; they have become focal points for innovative techniques in biotechnology and molecular medicine. Understanding their roles provides insights not only into fundamental biology but also into practical tools that are being developed to manipulate genetic information for beneficial uses.
Biotechnological Innovations
The integration of intron research into biotechnological advancements has opened new avenues for product development and process enhancement. One notable area is the development of transgenic organisms. Inserting introns into the genes of plants, for example, can improve their expression levels significantly, leading to better growth rates and resilience against environmental stresses.
Moreover, researchers have been harnessing introns for gene silencing technologies, such as RNA interference (RNAi). Introns can bolster the effectiveness of these methods by enhancing the stability and specificity of the RNA molecules involved. Here are some key points surrounding these innovations:
- Enhanced Gene Expression: Including introns can increase the efficiency of gene transcription in genetically modified organisms.
- Stability of RNA Products: Introns can shield mRNA from rapid degradation, thus prolonging its functional lifespan.
- Targeted Gene Editing: Techniques like CRISPR-Cas9 can utilize introns for precise modifications, improving agricultural crop strains or developing therapies for genetic disorders.
"The strategic use of introns in biotechnology not only fortifies our understanding of genomic functions but also paves the way for sustainable solutions to global challenges."
Intron-Based Gene Therapy
The promise of intron-based therapies is yet another facet of their application. Gene therapy aims to correct dysfunctional genes responsible for disease development. By leveraging the unique properties of introns, researchers hope to enhance the delivery and efficacy of therapeutic genes.
One approach involves using vectors that incorporate intronic sequences. These can improve the reliability of gene expression in target cells. Several points are noteworthy in this context:
- Increased Therapeutic Efficacy: Introns can facilitate more effective action of therapeutic proteins by ensuring proper processing, such as splicing, that produces functional mRNA.
- Reduced Immunogenicity: Utilizing introns can help to mask therapeutic genes from the immune system, making them less likely to provoke an adverse reaction.
- Broader Application Spectrum: This strategy can be applied to a vast array of conditions, ranging from genetic disorders to cancers, broadening the potential impacts of gene therapy.
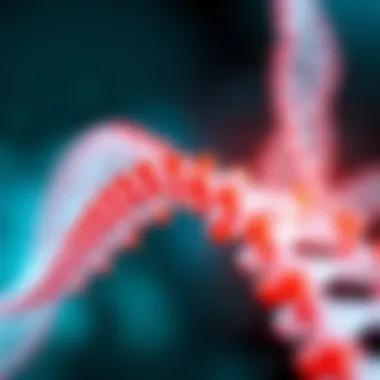
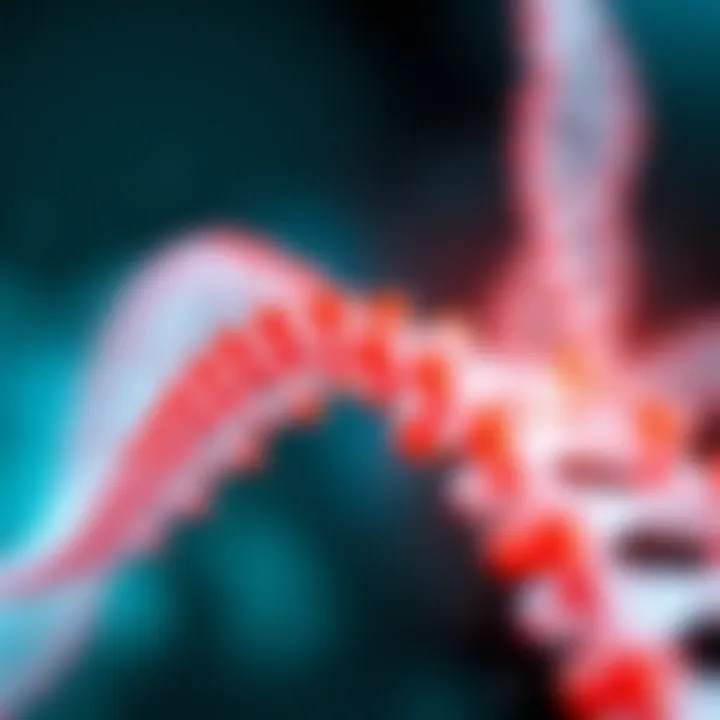
Challenges in Intron Research
Researching introns presents a rich tapestry of complexities that scholars must weave through to fully grasp their role within genetics. These challenges are not merely technical hurdles; they dig deep into the very framework of our biological understanding. Given that introns are often viewed as mere gaps in genetic material, deciphering their multifaceted contributions requires meticulous analysis and innovative approaches. The significance of addressing these challenges lies in the potential for unlocking new dimensions in genetic research and biotechnology.
Complexity in Analysis
Analyzing intronic sequences is akin to looking for a needle in a haystack. The sheer size of genomes can be daunting, with the average human genome containing over three billion base pairs, amongst which only a fraction is adventitious coding sequences. Introns, by their nature, can complicate splicing patterns and gene regulation processes, posing a challenge for researchers trying to pinpoint the exact function of each intron. Moreover, the variability in intron size and position across various organisms adds layers of intricacy to the analysis.
Researchers must employ advanced computational methods to sift through massive datasets. For instance, bioinformatics tools are often used to predict the potential functions of specific introns, but these predictions can still fall short without robust experimental validation. Consequently, while these tools are invaluable, they are also a source of potential inaccuracies, requiring a careful balance of data interpretation and biological evidence.
Issues with Sequencing Technologies
The rapid advancements in sequencing technologies have transformed the way we understand genetic data, yet challenges remain, especially concerning intron research. Traditional sequencing techniques, like Sanger sequencing, struggle to accurately capture large intronic regions due to their size. On the other hand, newer technologies, such as next-generation sequencing, promise higher throughput and speed. However, these methods also introduce unique problems, such as difficulties in distinguishing between closely related sequences or accurately aligning long repetitive regions.
These sequencing issues can result in incomplete or erroneous data, which may lead researchers astray. Furthermore, as the field shifts toward longer-read sequencing technologies, the need for improved algorithms to assemble and annotate intronic regions becomes evident. The complexity of intron structures means that researchers must remain vigilant about the potential for sequencing errors, making it imperative to constantly refine methods and validate findings through complementary approaches.
In summary, the intricate nature of intron analysis and the limitations of current sequencing technologies present formidable challenges in the quest to understand the full spectrum of intron function and significance. Continued evolution of these technologies, combined with a dedicated focus on improved analytical frameworks, is vital to unraveling the mysteries held within intronic DNA.
Future Directions in Intron Studies
In the ever-evolving landscape of genetics and molecular biology, understanding intron DNA takes on a new urgency. As research progresses, several potential pathways beckon, offering insights that not only deepen our knowledge of genetic regulation but also broaden horizons in medical and biotechnological applications. The significance of these future directions in intron studies cannot be overstated, as they interconnect various facets of biology, genetics, and health.
Emerging Research Trends
Current trends pointing to a greater understanding of introns suggest an increasing focus on their roles in gene expression regulation and alternative splicing mechanisms. Researchers are diving into sophisticated techniques like CRISPR-Cas9 to better manipulate introns and test their functions in vivo. This not only bridges gaps in our existing knowledge but also paves the way for potential treatments of diseases linked to splicing errors, such as certain cancers or genetic disorders.
Moreover, sequencing technologies have markedly advanced, allowing for comprehensive studies of intron structures across multiple species. In other words, scientists are pulling back the curtain on how introns operate across different organisms, enriching our comparative genomics database. Adopted tools, like single-cell RNA sequencing, may soon unravel individual cellular behaviors, emphasizing how introns can provide crucial regulatory functions in specific contexts.
Potential Areas for Exploration
Numerous avenues for exploration stand out in the growing body of research surrounding introns:
- Intron Function in Disease: Investigating how specific introns contribute to genetic disorders holds promise. If researchers can pinpoint how certain introns affect gene activity, there may be potential to formulate targeted therapies.
- Role in Aging: The relationship between intron functions and aging processes is an emerging field. Some researchers speculate that variations in intronic sequences may contribute to the aging process, opening up new dialogues regarding genomic stability and longevity.
- Synthetic Biology Applications: With the advent of synthetic biology, using introns for designed gene circuits could lead to novel biotechnological innovations. Manipulating intron-exon architectures might lead to optimized pathways for producing pharmaceuticals or biofuels.
- Epigenetic Interactions: Future studies could delve into how intron DNA interacts with epigenetic modifiers, potentially leading to insights on regulating genes without changing the actual DNA sequence.
Investigating these areas can lead to profound discoveries, influencing not only genetics research but also our understanding of evolutionary biology and disease mechanisms.
"The more we know about introns, the more tools we can develop to tackle complex genetic issues. It’s like finding a missing piece of a massive puzzle."
The End
The exploration of intron DNA has unfolded a world rich in complexity and significance, shedding light on an often overlooked aspect of our genetic material. Understanding the roles that introns play in gene regulation, evolution, and protein diversity is more than just an academic exercise; it has profound implications for various fields, from medicine to biotechnology.
Summary of Findings
Throughout this article, we have dissected the intricate functions and structural characteristics of intron DNA. Key findings include:
- Intron Variability: Introns exhibit significant diversity across different organisms, suggesting a dynamic evolution tailored to specific biological needs.
- Functional Significance: Our examination highlighted the role of introns in alternative splicing, which allows for the production of multiple protein isoforms from a single gene. This mechanism enhances cellular complexity and adaptability.
- Technological Innovations: Introns are not just passive elements; they offer exciting prospects in biotechnology, particularly in gene therapy and genetic engineering applications.
The interplay of introns and exons is like a finely tuned orchestra, where each part contributes to the symphony of gene expression and cellular function.
The Importance of Understanding Introns
Understanding introns is not merely beneficial but crucial for advancing our comprehension of genetics. As researchers delve deeper into the realms of genomics, recognizing the role introns play in shaping gene structure and function provides insights into biological processes and disease mechanisms. This understanding can lead to:
- Enhanced Disease Treatment: Grasping intron dynamics may revolutionize approaches in treating genetic disorders where splicing defects are implicated.
- Innovative Genetic Research: The ongoing study of introns illuminates pathways for developing cutting-edge therapies and innovations in genetic engineering.
- Broader Evolutionary Insights: As we probe the evolutionary significance of introns, we can better understand life's diversity and adaptability, opening up new avenues in evolutionary biology.
By placing intron DNA at the forefront of research, we position ourselves to harness its potential benefits fully, ensuring that the pursuit of knowledge translates into real-world applications. The narrative surrounding intron DNA is one of ongoing discovery, prompting us not only to question what we know but also to pave the way for future research that could unlock even more secrets of our DNA.