Understanding Lithium Cycles in Energy Storage
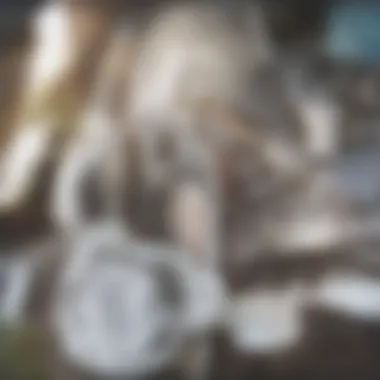
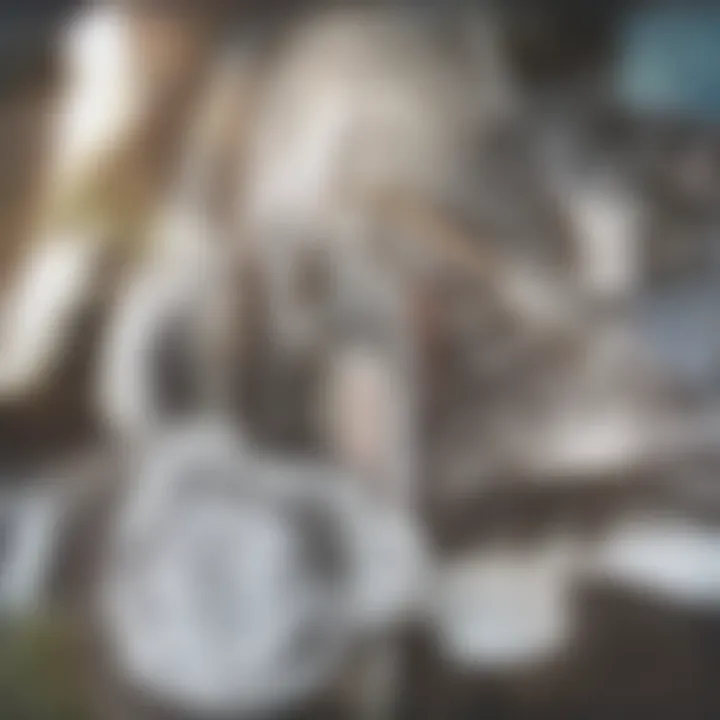
Intro
Lithium cycles play a central role in the ever-evolving landscape of energy storage technology. The application of lithium in rechargeable batteries has transformed how we use and manage energy, enabling a shift toward more sustainable practices. Understanding these cycles is crucial, not just for energy enthusiasts, but also for researchers, policymakers, and industry professionals focused on sustainability and technological advancement.
This article aims to provide an in-depth analysis of lithium cycles. We will delve into the thermodynamics that dictate lithium-ion behavior, examine the complexities surrounding its extraction, and investigate the challenges and innovations in recycling processes. The need for lithium continues to grow, and exploring its cycles helps to grasp the full implications of its use on environmental sustainability.
Research Methodology
Description of Research Design and Approach
In this exploration, we adopt a comprehensive research methodology that combines qualitative and quantitative approaches. This framework synthesizes existing literature, case studies, and recent innovations in lithium technology. By integrating various data sources, we aim to paint a holistic picture of lithium cycles. Moreover, interviews with industry professionals provide valuable insights that complement theoretical findings.
Materials and Methods Used in the Study
The study draws from diverse materials, including:
- Academic journals focusing on lithium extraction and recycling.
- Technical reports from leading battery manufacturers such as Tesla and Panasonic.
- Data from environmental assessments provided by research institutions.
Additionally, we utilize analytics software to process quantitative data regarding lithium production rates, recycling efficiencies, and environmental impacts. Such a blend of methodologies allows for a thorough examination of lithium cycles.
Discussion and Interpretation
Interpretation of Results in the Context of Existing Literature
The results indicate that while lithium holds great promise for energy storage, significant challenges remain. Analysis shows that extraction methods often introduce substantial environmental concerns. For instance, lithium mining consumes vast amounts of water and alters local ecosystems. On the recycling front, innovations are emerging, yet widespread implementation remains a hurdle.
Implications for Future Research or Practical Applications
Future research should focus on enhancing lithium extraction efficiencies and minimizing associated environmental impacts. Additionally, exploring alternative materials for energy storage may lead to more sustainable solutions. Practically, industries must prioritize recycling technologies to create a closed-loop system that lessens resource demand.
"Understanding lithium cycles is essential for advancing sustainability in energy storage. Addressing extraction, usage, and recycling challenges will dictate the future of this critical resource."
This exploration serves as a guide for students, researchers, and professionals seeking to understand the intricacies of lithium cycles and their broader implications for technological progress and environmental stewardship.
Prolusion to Lithium Cycles
Lithium cycles play a crucial role in today's energy infrastructure, particularly as the world shifts towards sustainable energy solutions. As a key component in lithium-ion batteries, lithium facilitates energy storage, which is essential for balancing demand and supply in renewable energy systems. The growing interest in electric vehicles further elevates its importance. The efficiency of lithium usage impacts not only technological performance but also environmental sustainability.
Definition and Importance
Lithium cycles refer to the natural and industrial processes through which lithium is extracted, utilized, and recycled. Defining these cycles is essential for understanding their relevance in various applications, such as batteries and energy storage systems. The processes involved include mining, processing, usage in devices, and subsequent recycling or disposal. Understanding these cycles allows researchers and industry professionals to optimize lithium usage, increase battery efficiency, and reduce ecological footprints.
In terms of significance, lithium is often regarded as the backbone of modern energy storage. The global shift to renewable resources, combined with the rise in electric vehicle adoption, underscores the need for effective lithium management. Without a comprehensive grasp of lithium cycles, addressing challenges in sustainability, efficiency, and environmental impact would be inadequate.
Historical Context
The historical context of lithium usage highlights its evolution from a niche chemical to a fundamental resource in energy technology. Initially, lithium was primarily used in specialized applications like psychiatric medications. However, the advent of lithium-ion batteries in the 1990s marked a turning point. This technology revolutionized electronic devices, providing a lightweight and efficient power source. As consumer electronics began to proliferate, so did the demand for lithium.
The rise of renewable energy sources such as solar and wind has further increased lithium's importance, as these technologies require robust energy storage solutions. Governments and companies have started to invest heavily in lithium extraction and utilization technologies, reflecting an urgent need to secure this critical resource.
"Lithium's transformation in the 21st century from an overlooked metal to a strategic resource reflects our changing energy paradigms."
As we face the pressing challenges of climate change and sustainable development, lithium cycles will continue to evolve. Understanding their complexities and implications will be vital for future innovations in energy storage technologies.
Mechanics of Lithium Ion Behavior
The mechanics of lithium ion behavior are fundamental to understanding how lithium functions within energy storage systems. This section unpacks the underlying principles that govern these dynamics, which are crucial both for students studying chemistry and for professionals engaged in battery technology. A firm grasp on these concepts provides insights into the efficiency and performance of lithium-ion batteries, which are at the center of many modern technological advancements.
Electrochemical Principles
The electrochemical principles governing lithium ions revolve around the movement of charged particles through various media, primarily in the context of batteries. In a lithium-ion battery, lithium ions move between the anode and cathode during charge and discharge cycles. This process induces a flow of electrons through an external circuit, providing the electricity that powers devices. The operation of lithium-ion batteries is primarily based on the redox reactions that occur during charging and discharging.
Key points to understand include:
- Lithium Ion Migration: Lithium ions migrate from the anode to the cathode during discharge and back again when charging, highlighting the reversible nature of the reactions involved.
- Electrode Material Influence: The choice of electrode materials can significantly impact the battery's efficiency, lifespan, and energy density. Materials like graphite for the anode and lithium cobalt oxide for the cathode are commonly used.
- Electrolyte Role: The electrolyte facilitates the transfer of lithium ions while also preventing short circuits. Its properties directly influence battery performance and stability.
As we understand these principles, we highlight the essential relationship between the chemistry of lithium and the efficacy of lithium-ion energy storage systems. These insights are critical for future innovation and optimization within the energy sector.
Charge and Discharge Cycles
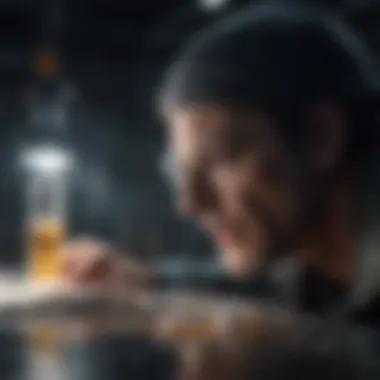
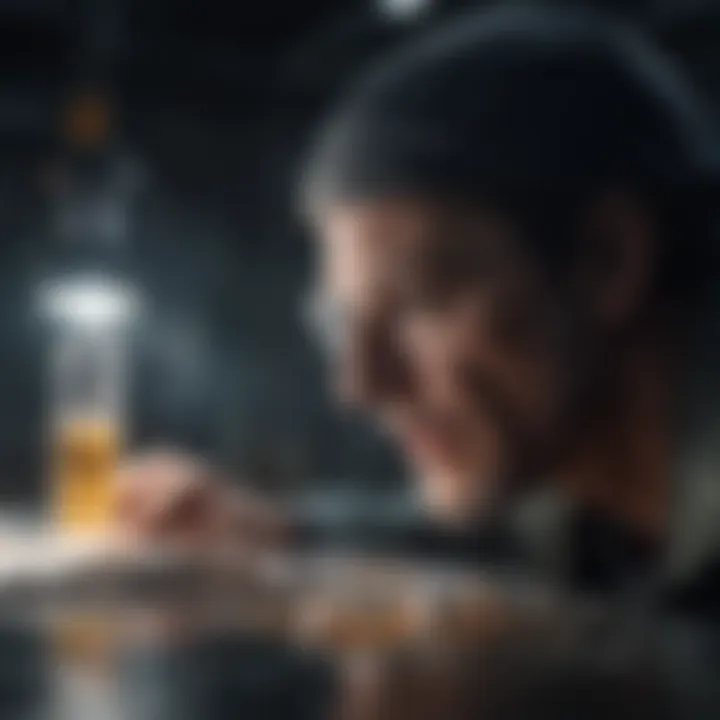
The charge and discharge cycles of lithium-ion batteries are integral to their application in energy storage technologies. This section will delve into how these cycles work and what factors can affect them.
- Charging Phase: When a lithium-ion battery is charged, lithium ions are driven toward the anode through the electrolyte. This process stores energy for later use. The charging time can vary based on several factors, including the current applied and the battery's capacity.
- Discharging Phase: During discharging, the reverse occurs. Lithium ions move back to the cathode, generating electrical power for devices. The discharge rate influences how quickly the energy can be consumed.
The efficiency of these cycles is influenced by factors like:
- Temperature: High or low temperatures can impair performance and cycle life.
- Voltage Levels: Operating consistently at high voltages can lead to faster capacity loss.
“Optimizing charge and discharge cycles is vital for extending battery life and ensuring reliable performance.”
Integrating this knowledge of charge and discharge processes covers necessary aspects required to advance battery technology and utilization practices.
Understanding these mechanics ultimately serves as a cornerstone in the continued development of lithium-based energy systems. This knowledge will facilitate enhanced designs that push current boundaries in energy storage capacity and efficiency.
Extraction Processes of Lithium
The extraction processes of lithium are critical to understanding its role in energy technologies and sustainability. The demand for lithium has surged, driven by its importance in rechargeable batteries for electric vehicles and renewable energy storage. This section discusses extraction methods in detail, shedding light on their respective benefits and drawbacks.
Mining Methods
Mining methods for lithium extraction can be categorized into two main types: hard rock mining and brine extraction. Each method has distinct processes and implications for production.
- Hard Rock Mining: This involves the extraction of lithium from igneous rock formations, specifically spodumene deposits. The process includes blasting, crushing, and grinding the rock to liberate lithium. The resultant ore undergoes chemical processes to convert lithium into a usable form. Hard rock mining tends to offer a more stable supply of lithium but involves larger environmental footprints due to land disruption.
- Brine Extraction: In contrast, brine extraction involves harvesting lithium from salt flats. This process entails pumping mineral-rich brine, which often contains lithium, to the surface where it evaporates in large ponds. As water evaporates, lithium concentrates, and the salts are further processed. Brine extraction is generally less invasive and can yield higher lithium concentrations; however, it is also slower, taking months or years to complete the full cycle.
Understanding these methods provides insight into the supply chain and resource availability, as well as the environmental challenges associated with each technique.
Brine vs. Hard Rock Extraction
The debate between brine extraction and hard rock mining raises various considerations for the industry.
- Resource Availability: Brine sources can often lead to higher lithium yields per ton, particularly from regions like the Lithium Triangle in South America. Hard rock sources, such as those in Australia, provide directly accessible lithium but may have lower yield rates compared to brine.
- Environmental Impact: The impact on local ecosystems greatly varies. Hard rock mining often results in significant land disturbance, while brine extraction necessitates managing large bodies of evaporating water, which can deplete local water supplies.
- Economic Factors: Brine extraction may incur lower operational costs but can be influenced by climatic conditions. Hard rock mining can be more economically favorable in some cases but involves higher initial setup costs and infrastructure.
Both methods present unique challenges and opportunities that must be balanced in light of growing lithium demands and sustainability commitments.
Utilization in Energy Storage Technologies
The role of lithium in energy storage technologies cannot be overstated. As societies continue to shift towards renewable energy sources, the demand for efficient and reliable energy storage systems increases significantly. Lithium has emerged as a crucial component in this domain due to its light weight, high energy density, and ability to undergo many charge and discharge cycles without substantial deterioration. This section delineates the importance of lithium usage in various energy storage applications, including its integration in battery systems and fuel cells, emphasizing the advantages and key considerations surrounding each technology.
Batteries: The Backbone of Lithium Cycles
Batteries are fundamentally dependent on lithium ions for their operation, making them central to the lithium cycle narrative. The most common type, lithium-ion batteries, utilize lithium salts as the electrolyte, ensuring efficient ion transfer between the anode and cathode during charging and discharging. These batteries power a wide spectrum of devices, from smartphones to electric vehicles, showcasing their versatility.
Key points regarding lithium-ion batteries include:
- High Energy Density: Lithium-ion batteries store a considerable amount of energy in a compact form, making them ideal for portable electronics and electric vehicles.
- Long Cycle Life: These batteries can typically withstand hundreds to thousands of charge cycles, sustaining their performance over years of use.
- Low Self-Discharge Rate: Lithium-ion batteries retain their charge for extended periods, thus providing reliability when needed.
However, there are considerations to be mindful of:
- Thermal Stability: Lithium-ion batteries can be prone to overheating, which can lead to safety risks if not properly managed.
- Material Sourcing: Extraction and processing of lithium can have environmental impacts, thus necessitating sustainable practices.
"The advancement of battery technology significantly dictates the progress of lithium cycles and energy storage solutions."
As technology evolves, so does the potential for lithium batteries to contribute meaningfully to energy storage solutions in diverse applications, making them indispensable in achieving energy efficiency goals.
Fuel Cells and Other Applications
While lithium-ion batteries are widely recognized, fuel cells represent another significant utilization of lithium in energy storage technologies. Fuel cells convert chemical energy into electrical energy through an electrochemical reaction between hydrogen and oxygen, producing water as a byproduct. Lithium is often employed in fuel cell systems to enhance performance and efficiency.
Advantages of using lithium in fuel cells include:
- Improved Efficiency: Lithium can assist in better catalysis, increasing the energy conversion efficiency of fuel cells.
- Reduced Weight: Lightweight lithium components can contribute to overall weight reduction in fuel cell systems, which is particularly important for applications in transportation.
- Durability: Integrating lithium compounds can enhance the longevity and stability of fuel cells under varying operating conditions.
In addition to batteries and fuel cells, lithium plays a role in various other energy storage solutions, including supercapacitors and thermal energy storage systems. Each application presents unique benefits and challenges regarding lithium utilization, but the overarching theme remains clear: lithium is a vital player in energizing the future of storage technologies.
Environmental Impact of Lithium Production
The environmental impact of lithium production is a crucial element of this exploration. As lithium becomes increasingly integral to energy storage solutions, understanding its ecological footprint is essential. The extraction and processing of lithium can have significant repercussions on local ecosystems, water resources, and communities.
Chemical Footprint
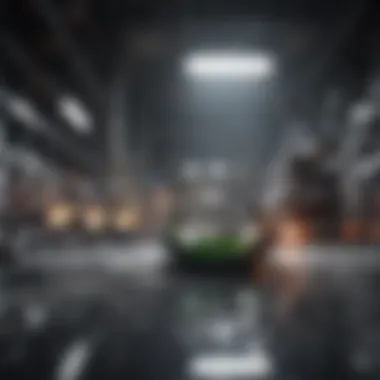
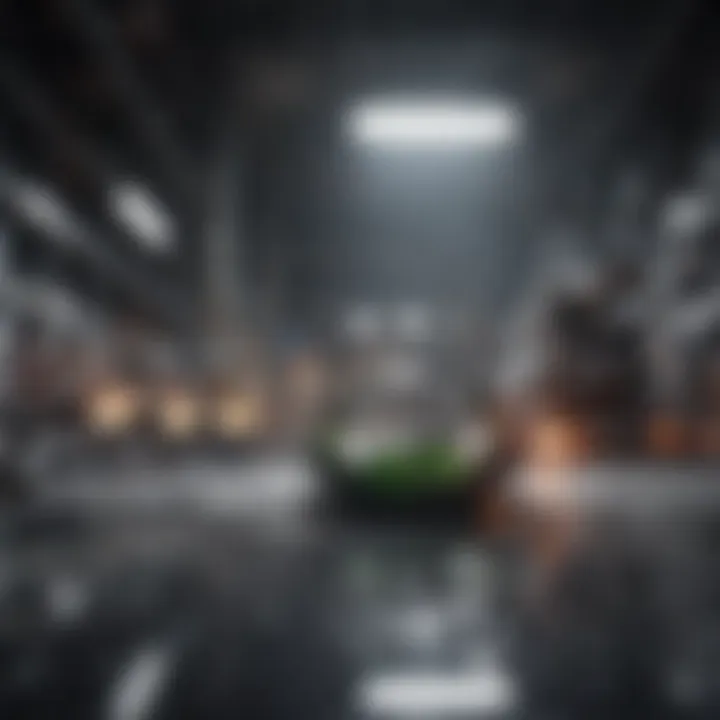
Lithium production entails various chemical processes that can contribute to environmental degradation. The chemical footprint of lithium production includes the emissions from mining operations, transportation, and refining processes. Some of these emissions release volatile organic compounds and greenhouse gases into the atmosphere. Notably, mining lithium from brine involves evaporation ponds, where large amounts of water are utilized, potentially leading to increased salinity and disruption of local flora.
The use of chemicals such as hydrochloric acid during extraction adds another layer of environmental concern. If not managed properly, these chemicals can contaminate surrounding soil and water, posing risks to wildlife and plant life. The implementation of stricter regulations on chemical use can help mitigate these issues, yet further research on greener extraction methods is essential.
Land and Water Use
Mining lithium significantly impacts land use and water resources. The extraction process usually requires vast expanses of land, which may lead to habitat loss, soil erosion, and changes in land composition. This disruption can have long-term consequences on biodiversity, affecting not only plant life but also the animals that rely on these ecosystems.
Water availability is another pressing issue. Lithium extraction, especially through brine evaporation, utilizes substantial amounts of freshwater. In regions where water scarcity is prevalent, lithium mining can exacerbate the existing demand on water resources. This necessitates a delicate balancing act between meeting energy storage needs and preserving water for local communities.
Regulatory Framework
A robust regulatory framework is vital for addressing the environmental impacts of lithium production. Governments across the globe are beginning to recognize the ecological challenges presented by lithium mining. Regulations must ensure sustainable practices that minimize the environmental footprint during every stage of lithium production.
As the market for lithium grows, so does the need for transparent reporting and compliance with environmental standards. Regulations should not only focus on immediate concerns but also consider long-term impacts on ecosystems and local communities. Policymakers must engage with environmental scientists to develop guidelines that prioritize sustainable mining practices and promote innovation in extraction technologies.
The future of lithium production lies in the balance between technological advancement and environmental stewardship.
Recycling and Circular Economy Initiatives
Recycling lithium and implementing circular economy initiatives play a crucial role in the future sustainability of lithium cycles. The rapid growth of energy storage technologies, particularly in batteries, has created an increasing demand for lithium. As a finite resource, ensuring a steady supply while minimizing environmental impact is essential. Recycling helps achieve these goals by reintroducing lithium and other valuable materials back into the supply chain, thereby reducing the need for virgin resources.
The transition towards a circular economy emphasizes not only recycling but also extending the lifecycle of products. This approach counters the traditional linear model, which follows a "take, make, dispose" pattern. By reclaiming materials through recycling, companies can significantly lessen resource depletion and decrease waste generation.
Here are some specific benefits associated with recycling lithium:
- Resource Recovery: Recycling can recover up to 95% of lithium, along with other precious materials such as cobalt and nickel from spent batteries. This recovery reduces the need for new mining operations.
- Reduced Environmental Impact: Battery production often results in significant ecological damage. Recycling mitigates this by lessening the carbon footprint and harm to land and water.
- Cost Efficiency: By utilizing recycled materials, manufacturers can often reduce production costs compared to sourcing and processing raw materials from mines.
However, several considerations must be addressed in the recycling landscape. Adequate infrastructure, investment in advanced recycling technologies, and consumer awareness are critical for improving recycling rates. Overall, initiatives in this realm are essential to balance the benefits of lithium usage with the environmental costs associated with its extraction and disposal.
Current Recycling Methods
The methods currently used for recycling lithium vary, primarily focusing on battery technologies. Some effective approaches are:
- Hydrometallurgical Processes: This involves using aqueous solutions to separate lithium from other materials in batteries. The process is considered environmentally friendly compared to traditional methods.
- Pyrometallurgical Processes: Here, high temperatures are applied to incinerate the battery material, which facilitates the recovery of lithium. This method can be less sustainable due to high energy consumption.
- Direct Recycling: This technique aims to recover the original structure of lithium-ion battery components without breaking them down into metals. This can maintain their efficiency during recovery.
Despite the advantages of these methods, challenges remain. Ensuring quality and purity of reclaimed lithium can be complex, and different battery chemistries require tailored approaches. Moreover, as battery technology continues to evolve, recycling processes must also adapt swiftly to meet these innovations.
Innovations in Recapturing Lithium
Recent years have seen an upsurge in innovations aimed at improving lithium recapture. These advancements focus on enhancing efficiency, reducing costs, and minimizing environmental impacts. Some notable innovations include:
- Selective Separation Technologies: New methods are being developed that target specific materials in mixed waste, allowing for more effective extraction of lithium while preserving other valuable components.
- Biotechnological Approaches: Research into using microorganisms to leach lithium from batteries has shown promise. These bioleaching processes are more eco-friendly than traditional methods.
- Advanced Sorting Technologies: Innovations in robotics and data analytics enhance material sorting in recycling facilities, increasing the speed and accuracy of lithium recovery before the recycling stage begins.
"The true potential of lithium recycling lies in our ability to innovate and adapt rapidly in response to changing technologies and market demands."
These innovations are crucial. As demand for lithium continues to rise, enhancing recycling capabilities will not only help sustain resources but also promote a more sustainable and circular economy.
In summary, recycling lithium and engaging in circular economy initiatives is pivotal for future sustainability. Through ongoing development in recycling methods and technologies, it is possible to address challenges while capitalizing on the environmental benefits and economic opportunities.
Future of Lithium Cycles
The future of lithium cycles holds significant promise and presents crucial challenges. As global energy consumption shifts towards more sustainable sources, the role of lithium becomes even more pronounced. Emerging technologies and innovations in this sector have the potential to revolutionize energy storage, making it more efficient while reducing environmental impact. Understanding the directions in which these cycles are headed is essential for stakeholders, including researchers, policymakers, and the industry.
Emerging Technologies
New advancements in lithium extraction and recycling processes are reshaping how the industry operates. Enhanced lithium mining techniques focus on reducing waste and minimizing environmental impact. Areas such as selective lithium recovery from brines and mining waste are showing potential. Furthermore, solid-state batteries are on the horizon, offering higher energy densities and greater safety compared to conventional lithium-ion cells. Solid-state technology may allow longer-range electric vehicles and faster charging times, presenting a leap forward in energy storage.
Moreover, innovations in the recycling process are noteworthy. Techniques like direct recycling aim to recover valuable materials directly from spent batteries, reducing the need for new raw materials. This method also helps to decrease the carbon footprint associated with production processes.
"Innovations in recycling can significantly mitigate the environmental challenges related to lithium cycles, leading to a more circular economy."
Listed below are some key technologies to watch in the lithium sector:
- Solid-state Batteries: Potential for improved energy density and safety.
- Direct Recycling: Efficiency in recovering materials from used batteries.
- Technology Integration: Combining AI and data analytics to optimize lithium extraction processes.
Socioeconomic Implications
The socioeconomic implications of lithium cycles are profound. As demand for lithium rises, driven by electric vehicles and renewable energy solutions, the need to address the ethical dimensions of resource extraction intensifies. Countries rich in lithium reserves also face the dilemma of balancing economic gain with environmental protection and community impact.
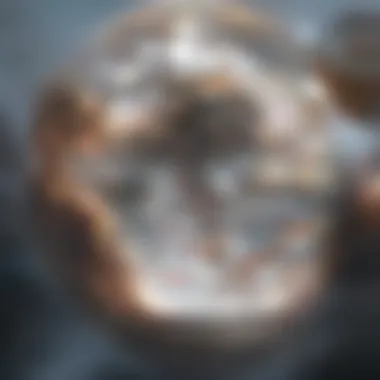
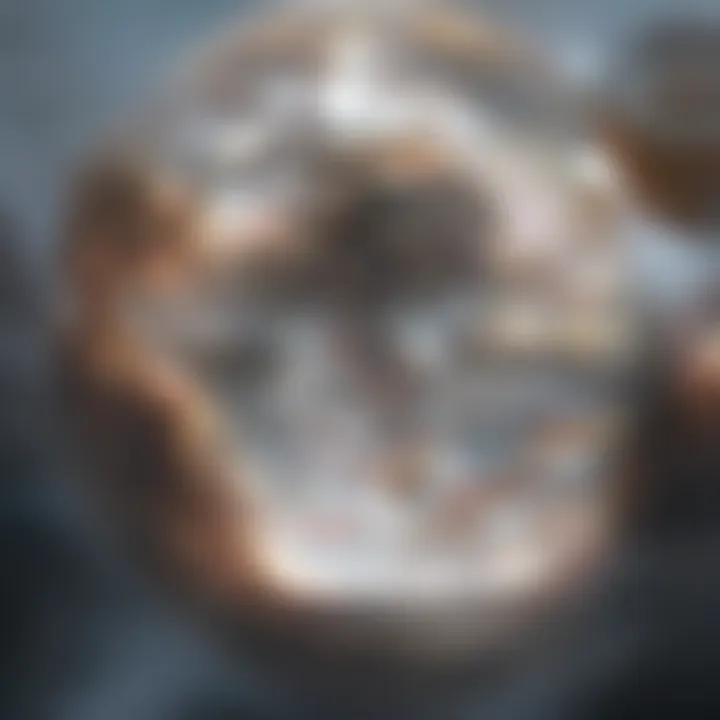
Lithium extraction processes often affect local communities and ecosystems. Investments in sustainable practices and technologies can provide local employment opportunities while supporting community development. However, there is a risk that the gains may not be equitably shared, potentially leading to social tensions.
Furthermore, geopolitical factors complicate this landscape. Countries with substantial lithium resources may experience fluctuations in power dynamics and economic stability. As lithium becomes increasingly vital for the energy transition, nations that can secure stable supply chains will hold strategic advantages.
Understanding these implications requires careful analysis and ongoing research into the balance between technological advancement and social equity.
The Role of Global Supply Chains
The global supply chains for lithium are pivotal in ensuring the stability and efficiency of lithium cycles. These supply chains encompass every step from extraction to utilization in energy storage. Understanding their role is crucial to grasping the complexities of the lithium market and the broader implications for sustainability.
Global supply chains involve multiple stakeholders, including mining companies, manufacturers, and governments. The cooperation of these entities enables a steady flow of lithium, which is essential for the production of batteries and other applications. The interconnectedness of these players can lead to efficiencies and innovations but also creates vulnerabilities when faced with geopolitical tensions or supply chain disruptions.
Geopolitical Considerations
Geopolitical factors greatly influence lithium supply chains. Countries rich in lithium, such as Argentina, Chile, and Australia, hold significant importance in this landscape. Political stability in these regions can impact global supply. For instance, shifts in trade policies or regulations can alter export capabilities, influencing market prices and availability.
Moreover, the strategic importance of lithium in energy transition makes it a focus of geopolitical competition.
- Countries are investing in securing their access to lithium resources to ensure their technological advancements are not hindered.
- Nations are forming partnerships to facilitate resource sharing and mitigate risks associated with supply chain disruptions.
This reality underlines the necessity for diversified sourcing strategies by companies dependent on lithium.
Market Dynamics
The dynamics of the lithium market are complex. Demand continues to rise due to the proliferation of electric vehicles and renewable energy technologies. In turn, this increasing demand puts pressure on the supply side, which must adapt to changing market conditions.
- Price Volatility: Prices can fluctuate significantly based on geopolitical events, resource scarcity, and shifts in manufacturing demand.
- Market Players: Major players include Albemarle Corporation and SQM, which dominate the extraction and production landscape. These companies often navigate not just the extraction of lithium but also the negotiation of trade agreements and enforcement of environmental regulations.
The alignment of supply chain logistics with market demands can greatly affect operational efficiencies. Companies that can adapt quickly to changes in demand are more likely to succeed, while those stuck with rigid supply chains may face challenges.
"The interdependency of geopolitics and market conditions makes it vital for stakeholders to continuously assess their strategies in the lithium landscape."
Challenges Facing Lithium Cycles
Lithium cycles encompass a complex interplay between extraction, utilization, and recycling. However, these cycles are not without their challenges. Understanding these hurdles is essential for researchers, practitioners, and policymakers. Addressing these challenges can foster sustainable practices that ensure lithium remains a viable resource in the growing demand for energy storage solutions.
Resource Scarcity and Demand
The demand for lithium is rising due to its critical role in modern technologies, especially lithium-ion batteries. These batteries power electric vehicles and store energy for renewable sources, creating urgency in lithium extraction.
Despite abundant lithium reserves, significant challenges exist. The geographical concentration of lithium reserves means that some regions may struggle to meet local and global demands. This scarcity can lead to competitive market conditions, driving prices up and creating supply instability. Sustainable extraction practices are essential to balance environmental considerations and meet global needs.
The increasing demand also raises concerns about the social and economic implications of lithium resource extraction. Communities near mining operations may face exploitation or environmental degradation. Thus, understanding resource scarcity and corresponding demands is crucial for long-term sustainability.
Technological Barriers
While lithium is a focal point in energy technology, barriers exist that limit its efficient extraction and recycling. Current extraction methods often rely on water-intensive processes that can deplete local resources. Innovations in extraction technology, such as direct lithium extraction, hold promise but require significant investment and research.
The recycling of lithium is another barrier. Despite the need for effective recycling, current methods are not yet optimized for efficiency or cost-effectiveness. Enhancing these technologies will not only recover valuable lithium but also reduce the environmental impact of mining.
"Advancements in technology are vital to overcome barriers in lithium extraction and recycling, promoting a circular economy within the industry."
Moreover, as more batteries reach end-of-life, the challenge will intensify. Developing infrastructure capable of processing used batteries safely and effectively is essential to manage the increasing lithium waste.
Concluding Thoughts
The exploration of lithium cycles presents vital insights into their significance for current and future energy systems. Understanding these cycles not only illuminates the technological advancements but also highlights the ongoing environmental and social challenges related to lithium extraction and use. The interaction of these cycles with global supply chains and geopolitical factors frames the broader context in which research and innovation regarding lithium occur.
Summary of Key Points
In summary, this article covered several important aspects:
- Lithium plays a crucial role in energy storage technologies, especially in batteries and fuel cells.
- The extraction processes, whether brine or hard rock, have varying impacts on the environment and economy.
- Environmental consequences of lithium production require attention, including regulatory oversight and sustainable practices.
- Recycling initiatives are essential for extending lithium's lifecycle and reducing dependency on new material.
- Future trends depend on technological advancements and socioeconomic factors that will shape the global demand and supply of lithium.
These points underscore the multifaceted nature of lithium cycles and their implications across various fields.
Call for Ongoing Research
The landscape of lithium cycles is rapidly changing, necessitating continued research and development. Exploration in innovative extraction methods can provide sustainable alternatives to current practices. Additionally, advancing recycling technologies will be key in addressing resource scarcity and waste management.
Future research should also consider:
- Studying the impact of new materials that can complement or replace lithium in energy storage solutions.
- Analyzing the social implications of lithium mining, especially in regions where communities are affected by extraction operations.
- Investigating the long-term effects of increased lithium demand on ecosystems and biodiversity.
The journey does not end here; understanding lithium cycles is crucial for transitioning toward sustainable energy futures. By focusing on equity, environmental sustainability, and technological advancement, we can harness the potential of lithium responsibly.